Serotoninergic Chemoreceptive Neurons: A Search for a Shared Function
The serotonin-producing neurons of the brainstem raphe nuclei are a component of the reticular formation and form two main clusters of nuclei which innervate the midbrain and forebrain and the pons, medulla, and spinal cord. The superior raphe group consists of four main nuclei [the largest being the dorsal raphe nucleus (B7and B6)], and the inferior group consists of five main nuclei (the largest being the raphe magnus and raphe pallidus). Carbon dioxide chemoreceptors on medullary raphe serotoninergic neurons have been identified in in vitro preparations (1) and in whole animals (2). Recent characterization of dorsal raphe serotoninergic neurons in the midbrain has revealed that they also are chemosensitive (3). Somewhat surprisingly, the authors suggest their results are relevant to sudden infant death syndrome (SIDS), panic disorder, and migraine headaches. Severson et al. (3) state that “despite a tendency to study these neurons in relation to only a shared brain function or disease, their highly divergent projections and the homogeneity of their cellular properties [(4) ] suggest that there may be a shared function of serotonergic neurons.” What is this “shared function” with respect to chemoreceptivity to CO2 and pH? One is puzzled by this cognitive leap from studies of a handful of raphe neurons in the medulla and midbrain to the whole serotoninergic system. Even if one allows for a homogenous anatomical and pharmacological classification, to invoke a “shared function” may appear overly simplistic and grandiose. The number of functions associated with serotonin (5-HT) is nearly endless. Can they all be linked?
Several authors have tried before to find this shared function. Brodie and Shore proposed a metabolic role for 5-HT in the neuronal activity of the brain (5). In their hypothesis, norepinephrine and 5-HT modulated opposite systems in the brain, based on Hess’s concept of the functional integration of the autonomic system with the CNS (6). 5-HT, it was argued, is the modulator of the trophotrophic system. As the name implies, trophotrophic is the literally the nutrition for the nutritional network, or, on an organismic level, those behavioral patterns that are recuperative in nature, such as sleep, hibernation, or eating. Later, Woolley proposed that 5-HT is essential for normal mental health (7). This hypothesis was based on the structure of 5-HT, which is similar to that of lysergic acid diethylamide (LSD), and is discussed elsewhere in a historical chapter (8). In 1975, Scheibel et al., using Golgi-stained brainstem material, noted a close relationship between the raphe reticular neurons and blood vessels (9). They proposed the raphe neurons function either as a chemoreceptor or a mechanoreceptor, although a neuroscretory role of a bioactive substance into the vascular system from these raphe neurons could not be ruled out.
If a shared function could be agreed upon, how would this finding impact therapeutic pharmacological interventions to ameliorate 5-HT neuronal dysfunction? I will first discuss the results reported by Severson et al. (3) and emphasize the earlier work performed by Scheibel et al. (9), then consider if such a shared function makes evolutionary sense. Finally, I will deal with one shared function—a mechanism of action—of serotoninergic neurons suitable for such a Herculean task, namely homeostasis.
Severson et al. (3) made perforated–patch clamp recordings from neurons in rat midbrain slices while pCO2 was changed between 9% and 3% (pH 7.18 to pH 7.58). Out of 100 recordings, sixteen were acidosis-stimulated in the dorsal raphe nucleus and satisfied the criteria of being chemosensitive. Studies of midbrain raphe cultures showed out of seventy-eight neurons studied for their response to acidosis, twenty-one neurons were acidosis-stimulated, with an increase in firing rate to 405 ± 70% above that from control-treated neurons, in response to a decrease in pH from 7.4 to 7.2. The neurons were identified by location (in the case of the slices), firing characteristics, and in a selected number of cases by immunocytochemical staining with a specific antibody against tryptophan hydroxylase (TPH). In subsequent experiments, the authors demonstrated that major branches of the basilar artery outline the dorsal and median raphe nuclei. The arteries were visualized by intra-arterial injections of fluorescent albumin, combined with TPH-specific immunocytochemistry. The authors establish the anatomical framework for their physiological studies. In order to solidify their claim, electrophysiological evidence of an acidosis-stimulated TPH-positive neuron with a dendritic branch around a fluorescent-albumin containing artery is presented.
As Scheibel et al. (9) wrote nearly three decades ago after they found raphe neurons in contact with blood vessels: “A chemosensitive role for these raphe elements represents a reasonable extension of putative reticular function. Proactive evidence already available suggests that some brain stem neurons may be sensitive to blood CO2 levels [(10) ], and to the osmolarity of the circulating medium [(11, 12) ]. Indeed, it is conceivable that raphe neurons themselves may be sensitive to one or another of these...given their intimate neurovascular position and their apparent obligatory role in the onset of sleep [(13) ], they may be capable of detecting circulating substances such as plasma cortisol and ACTH, etc. [(12, 14) ], whose concentrations are time-locked to circadian rhythms and possibly to the shorter rest-activity cycle of Kleitman [(15) ].” The raphe serotoninergic neuronal ties to cortisol and locomotor activity exist in all raphe nuclei neurons (4, 16). The paper by Severson et al. (3) now suggests a tie between serotoninergic raphe neurons and chemoreceptors.
We proposed that the raphe neurons are a key component of neural tissue homeostasis because they are functionally and morphologically plastic and respond to a variety of neuronal and non-neuronal signals from internal and external environments (17). It is easy to appreciate how 5-HT neurons are pharmacologically dynamic: 5-HT synthesis is regulated by enzyme and substrate levels, O2 and reduced cofactor availability, and the phosphorylation state of TPH (18, 19) (Figure 1⇓). Release of 5-HT can either be vesicle- or transporter-mediated and the number of 5-HT receptors at the cell surface can be modulated. The morphology of 5-HT neurons is plastic, and changes in innervation density occur during development, aging, trauma, stress, and certain diseases, such as depression, alcoholism, Alzheimer Disease, Parkinson Disease, and suicide.
What is more interesting is that the cytoskeleton (i.e., the dynamic microtubular network) of target neurons is modified by fluctuating 5-HT concentrations that regulate the availability of S-100B (formerly called S-100β) a neurite extension factor produced by astrocytes and Schwann cells (16). Cheng et al. (20) showed that removal of 5-HT from adult rat cortex resulted in a loss of 70% of the synaptic contacts within seventy-two hours. Thus, a structural imprint in the neuronal circuitry can be formed to represent the fluctuations in 5-HT. The dynamic interplay between 5-HT and neuronal circuits is the basis of the idea of neural homeostasis.
Homeostasis can be locally regulated. A shared function needs to originate in the raphe nuclei, where the intrinsic raphe interconnections are evident. Most raphe neurons show a firing pattern linked to motor activity, and a increase in size linked to stress-related hormones (4). 5-HT raphe neurons are reticular neurons and they sample neuronal activity as it ascends and descends the brainstem (Figure 2⇓). Additionally, these neurons have specialized contacts with glial, ependymal, and endothelial cells (18, 21). What we did not know was the extent to which they function as central pH chemoreceptors. If we assume these serotoninergic neurons comprise a homeostatic system, then one can envision the dynamics of breathing, temperature, locomotor activity, stress, and sex all impinging on higher order cognitive functions such as learning and memory and even creativity. Thus, SIDS, anxiety, and migraines are but one subset of a homeostatic network involving serotoninergic neurons with pH chemoreceptors.
How do we develop therapeutic pharmacological interventions to ameliorate 5-HT neuron dysfunction, if the dysfunction impacts on the shared serotoninergic function of homeostasis? We can adjust the mechanical and chemical sensors, or the motor and endocrine outflows to try to stabilize fluctuations, but the problem may be the homeostatic system itself, evidenced as a 5-HT neuron dysfunction. Selye (22) spent a lifetime trying to quantify and regulate our response to stress, and yet the problem persists into the twenty-first century. A new concept of therapy needs to be formulated, one that considers evolution and homeostasis and the brain and environment as a whole. It will involve pharmacological modulation of: 1) neuronal raphe firing, 2) serotonin release, and 3) 5-HT receptor activity/availability. Additionally, we may need to adjust external “homeostatic” parameters such as the availability of carbon dioxide and molecular oxygen; our food choice; activity and stress levels; the amount of energy we expend and sleep we need; the time of day or season of the year or our stage of life and health status; and the dosing and absorption rate of modulating drugs. Ameliorating dysfunctional 5-HT neurons in unhealthy humans can help reestablish primordial Feng Shui within the brain, and encourage mental health.
Serotonin Synthesis and CO2. Primitive cells used the enzyme ribulosebisphosphate (RuBP) carboxylase/oxygenase to fix atmospheric carbon dioxide to produce biologically useful molecules; the same enzyme can alternatively utilize atmospheric oxygen, effectively catalyzing a hydroxylation reaction. The activity of tryptophan hydroxylase (TPH) is the rate-limiting step in 5-HT synthesis and its activity was used by Severson et al. (3) to identify serotoninergic neurons. The second step of 5-HT synthesis involves 5-HT decarboxylase, which cleaves off the alpha carboxyl group to form CO2. The aromatic decarboxylase enzyme is detected in bacteria and in plants has attracted considerable attention because of its role in the biosynthesis of pharmaceutically important monoterpenoid indole alkaloids (23). Thus, a relationship between CO2 and serotonin synthesis has long existed.
Homeostasis and 5-HT Neurons. A schematic diagram illustrates the homeostasis involving the serotoninergic raphe neurons and mental health, represented by a six-sided star. The relationships between hard-wired (point-to-point) systems of sensory, motor and association systems are represented by black triangle. The relationships between the limbic, endocrine and autonomic (PNS) systems is represented by the red triangle. The serotoninergic raphe neurons is shown as the large blue circle overlying the two triangles and serves to represent the shared function of serotonin on these systems. Note that serotoninergic neurons are in contact with vascular, glial, and ventricular systems, which supply gases, nutrition, and thermal inputs. PNS, peripheral nervous system.
Acknowledgments
Research supported by NIMH, K05 MH01838-04 career development award and R01 MH55250-07.
- © American Society for Pharmacology and Experimental Theraputics 2004
References
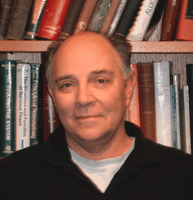
Efrain C. Azmitia, PhD, is a Professor of Biology and Center for Neural Science and Adjunct Professor of Psychiatry at the Medical Center of New York University. Please address all correspondence to ECA. Email: eca1{at}nyu.edu; fax 212-995-4015.