Stem Cells from Differentiated Cells
Urodele amphibians, such as salamanders and newts, are largely known for their extraordinary regenerative capabilities. These animals can regenerate amputated limbs, tail, removed retina and lens, heart, spinal cord, brain––virtually anything as long as the animal is left alive (1). What is truly striking is that regeneration occurs in adult amphibians and involves the usage of already existing terminally differentiated cells, rather than undifferentiated stem cells. For example, after limb amputation, the tissues in the stump, such as muscle and cartilage, lose their tissue characteristics that distinguish them. This “dedifferentiation” process leads to the formation of the blastema (2, 3). Cells within the blastema are undifferentiated, but soon after a period of proliferation, these cells redifferentiate to build a faithful replica of the lost part of the limb (Figure 1⇓). Likewise, when the eye lens is removed from urodeles, the pigment epithelial cells (PECs) of the dorsal iris dedifferentiate, thereby losing their characteristic pigmentation and producing a lens vesicle, which, in turn, differentiates to lens epithelium at the anterior and to lens fibers at the posterior (Figure 1⇓). Even isolated single PECs can transdifferentiate to lens in vitro (4). From these two examples, we can firmly conclude that regeneration occurs via “transdifferentiation,” because one terminally differentiated cell has been reprogrammed to become another. This reprogramming has virtually no parallel in other normal differentiation processes.
In the past few years, however, many studies have shown that stem cells––cells that are reserved and used for repair––might have greater potential for regeneration than originally thought. Stem cells can be local (i.e., located in the brain and involved in nervous tissue repair), or nonlocal (i.e., hematopoieitic and involved in repair of several different tissues, such as liver, nervous, or cardiac) (5–8). Despite some spectacular results, a direct role for non-local stem cells in repair has been disputed, mainly because stem cells can fuse with the cells at the site of repair (9). One should keep in mind that, unlike the strategy used by urodeles, mammalian stem cells do not undergo dedifferentiation, but rather pre-exist as multipotent cells. Therefore, this repair strategy is not the same as the one used in urodeles.
It has been hypothesized, however, that some similarities between stem cells and transdifferentiating cells, at the molecular level, are inevitable (2, 10). For example, mesenchymal stem cells located in the bone marrow can differentiate to chondrocytes, myocytes, osteoblasts, or adipocytes. Therefore, at some particular stage these cells might not differ from blastema cells. Such common signatures of stem cells and dedifferentiated cells might provide very important insights about the biology of stem cells and of transdifferentiation of terminally differentiated cells.
A recent paper provides the first strong support that mammalian myotubes can transdifferentiate by generating progenitor cells (11). The murine C2C12 myogenic cell line, which upon serum withdrawal fuses into characteristic multinucleated myotubes, was used in this study. Such myotubes cannot normally revert to single-celled myoblasts. Chen et al. (11) screened this cell line with individual samples from a library of 50,000 discrete small molecules for four days, after which the cells were transferred to medium containing either osteogenesis-inducing substances (such as ascorbic acid, dexamethasone and glycerophosphate) or to medium containing adipogenesis-inducing substances. The cultures were maintained for seven additional days and then assayed for osteogenesis or adipogenesis using specific markers. A 2-(4-morpholinoanilino)-6-cyclohexyaminopurine analog was found to inhibit the formation of myotubes, resulting in mononucleated cells. Under conditions favoring osteogenesis, 35% of the cells stained positive for the alkaline phosphatase, a marker for osteogenic activity. Similarly, this 2,6-disubstituted purine compound, under conditions that favor adipogenesis, induced fat-cell morphology, with oil droplets accumulating inside the cytoplasmic membrane and with 40% of the cells positive for the adipose marker oil red O. The compound was named reversine because of its ability to reverse terminally differentiated cells to progenitor cells, which in turn were able to differentiate to different cell types. What Chen et al. have discovered is that murine cells are able to dedifferentiate and create multipotent progenitor cells. In this sense, they have created for the first time stem cells from differentiated cells. Conceivably, these cells could be regarded as analogous to the blastema cells created by dedifferentiation during limb regeneration (Figure 1⇓).
These finding are of enormous importance. First, they show that such screening can identify small molecules with transdifferentiation properties. These molecules can be used for therapeutic applications, since similar strategies can be devised for other cell types as well. Secondly, the generation of multipotent progenitor cells from differentiated cells can provide useful information and comparison with the transdifferentiation events seen in urodele amphibians. Such comparisons at the molecular level will eventually lead to the understanding of mechanisms used by the two strategies of regeneration, that of the urodeles and that of stem cell recruitment.
It will also be interesting to see whether such small molecules can induce dedifferentiation in a variety of cell types. In other words, can we identify a common signal for dedifferentiation, or are signals unique to different cell types? In urodeles, for example, it would be logical to assume that common signals have prevailed. Other implications of the identification and usage of these small molecules are in their application to induce regeneration in mammals. Can they work also in vivo? The findings by Chen et al. will generate much interest and will stimulate research to answer these questions.
Transdifferentiation in urodele limb and lens regeneration and in a committed murine cell line. After amputation of the newt limb (or tail) the intact terminally differentiated cells (such as, mesenchymal cells, myotubes, nervous tissue, bone, or cartilage) dedifferentiate by losing the characteristics of their origin. This dedifferentiation process produces the blastema cells, which then redifferentiate to reconstitute the lost limb. After lentectomy, the dorsal iris pigment epithelial cells lose their pigments and become dedifferentiated cells, which consequently regenerate a perfect lens. A small molecule, reversine, is able to induce dedifferentiation of myotubes formed by murine C2C12 cells. This dedifferentiation produces mesenchymal progenitor cells that are able to differentiate to different cell types, such as adipocytes and osteoblasts. According to this scheme, the reversine produced progenitor cells could be analogous to the blastema cells or other dedifferentiated cells used during regeneration in urodeles.
- © American Society for Pharmacology and Experimental Theraputics 2004
References
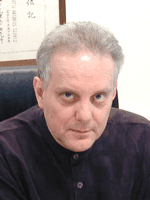
Panagiotis A. Tsonis, PhD, is a Professor of Molecular Biology and the Leonard A. Mann Chair in the Sciences at the University of Dayton, Ohio. He is very interested in many issues of tissue regeneration, the mechanisms of transdifferentiation, and induction of regeneration in mammals. E-mail Panagiotis.Tsonis{at}notes.udayton.edu; fax 937-229-2021.