Combating Atherosclerosis With LXRα And PPARα Agonists: Is Rational Multitargeted Polypharmacy the Future of Therapeutics in Complex Diseases?
Pathologic changes caused by atherosclerosis in the cardiovascular system, such as coronary heart disease, aneuyrisms of the aorta, and ischemia/infarction of the brain or limbs, are nowadays the most profound threat to the quality and expectancy of life in developed countries (1). Lipid metabolism and circulating cholesterol and triglycerides are crucial in the development of atherosclerosis. Native low-density lipoprotein (LDL) is manufactured and secreted into the circulation by the liver as a carrier of cholesterol to peripheral tissues. Macrophages contribute to the vascular accumulation of cholesterol by absorbing the oxidized forms of LDL and adhering to inflamed endothelium to ultimately form stenosis-causing plaques (2). Pharmacologic interventions that reduce the serum levels of LDL cholesterol by inhibiting de novo synthesis of cholesterol from AcetylCoA in the liver, such as the 3-hydroxy-3-methylglutaryl coenzyme A (HMG-CoA) reductase inhibitors termed statins, together with dietary restriction of cholesterol uptake, are the main preventive/therapeutic approach to atherosclerosis-related pathologic conditions (3).
Macrophages are capable of excreting cholesterol into the circulation as high-density lipoprotein (HDL), in combination with the apolipoprotein (apo) A1 and E1 acceptor molecules. Circulating HDL is then taken up by the liver and its cholesterol fraction is directly excreted into the bile or metabolized to bile acids (4, 5). The reverse cholesterol movement from the periphery to the liver is a promising novel target for pharmacologic intervention in the prevention or treatment of atherosclerosis. Agonists of the liver X receptor (LXR) influence this pathway and produce beneficial effects on lipid metabolism, indicating that they can be used against atherosclerosis (6–8). LXR, which functions as a sensor of cholesterol levels in tissues (5–8), is a type II nuclear receptor that consists of two isoforms, LXRα and LXRβ. The former is important for the regulation of cholesterol metabolism, and is expressed in the liver, spleen, adipose tissue, lung, and pituitary gland, whereas the latter is expressed ubiquitously. LXRα binds to and is activated by a variety of oxysterols, such as 22(R)-hydroxycholesterol, 24(S)-hydroxycholesterol, 24(S), 25-epoxycholesterol, and 27-hydroxycholesterol, as well as by metabolites of the mevalonate cholesterol synthesis pathway.
Activated LXRα stimulates transcription by forming a heterodimer with the retinoid X receptor (RXR) and binding to the consensus responsive sequence DR4 in the promoter regions of several target genes, including those of cholesterol 7α-hydroxylase (CYP7A1), cholesterol ester–transfer protein (CETP), ATP-binding cassette proteins (ABC), apolipoprotein E (ApoE), lipoprotein lipase (LPL), and sterol response element-binding protein 1c (SREBP-1c). Through transcriptional regulation of these and other target molecules, LXRα decreases circulating LDL and tissue cholesterol by 1) facilitating cholesterol excretion in the gallbladder and catabolism through bile acid formation in the liver, 2) reducing cholesterol absorption in the intestine, and 3) promoting cholesterol efflux from peripheral tissues such as resident macrophages (Figure 1⇓) (6–8). LXRα agonists also increase circulating levels of HDL cholesterol by stimulating the expression of ABCA1, apoE, and phospholipid transfer protein (PLTP) (9).
Administration of LXRα agonists in addition to conventional cholesterol-lowering compounds could produce added benefit in the treatment/ prevention of atherosclerosis.
The apparent beneficial effects of LXRα agonists on lipid metabolism, including a decrease of LDL and an increase of HDL, however, are associated with increased lipogenesis and production of triglycerides in the liver with resultant hypertriglyceridemia, an independent risk factor for atherosclerosis (Figure 1⇓) (8, 10). This activity of LXRα is mediated by its induction of SREBP-1c, fatty acid synthase (FAS), sterol coenzyme A desaturase 1 (SCD-1), and acyl coenzyme A carboxylase (ACC) via direct activation of their promoters (8). SREBP-1c, a helix-loop-helix type transcription factor, plays a central role in LXRα-mediated lipogenesis by stimulating the transcription rates of several genes whose products are associated with fatty acid metabolism (10). Development of selective LXRα agonists, preserving the beneficial reverse cholesterol transport effect but devoid of the triglyceride-producing effect, would be ideal for the prevention or treatment of atherosclerosis, but no such compounds are as yet available.
In their recent article in The Journal of Pharmacology and Experimental Therapeutics, Beyer et al. attempted to diminish the pro-lipogenesis and hypertriglyceridemia effect of LXRα agonists by co-administering peroxisome proliferator activator receptor α (PPARα) agonists, known anti-lipogenic compounds (11, 12). Like the LXRs, PPARα belongs to the nuclear receptor superfamily and is distributed in tissues that have high lipid-metabolizing activity, such as the liver, brown fat, kidney, heart and skeletal muscles. Fatty acids, eicosanoids, and the fibrates (e.g., fenofibrate, clofibrate, and WY14643), a well-known class of hypolipidemic drugs,s are PPARα ligands (5). As with the LXRs, PPARα forms a heterodimer with RXR and stimulates the transcriptional activity of its responsive genes by binding to its consensus sequence, DR1, located in their promoter regions. PPARα activates β-oxidation in the liver, generating energy by catabolizing fatty acids, by increasing the transcription rates of the genes of several enzymes involved in this process. Furthermore, PPARα increases the production of the apolipoproteins apoAV and apoCIII, which results in decreased levels of triglycerides in the circulation (12). Thus, activation of PPARα reduces the fatty acid content of the liver and the circulating levels of triglycerides––exactly the reverse of the effects of LXRα activation (Figure 1⇓). Some of the PPARα agonists also slightly increase HDL cholesterol in humans (13), thus working in the same direction with the LXRα agonists.
Bayer et al. demonstrated that co-treatment of mice with the LXRα agonist T0901317 and the PPARα agonists fenofibrate or WY14643 successfully attenuated the elevation of circulating triglycerides induced by LXRα activation, even though the content of triglycerides in the liver persisted at elevated levels (11). The expression of SREBP-1c remained stimulated during the co-administration of the LXRα and PPARα agonists, whereas fatty acid β-oxidation in the liver was increased, explaining the observed triglyceride findings in the serum and liver. The combined treatment also produced synergistic elevation of the serum levels of HDL-cholesterol, enlargement in the diameter of the HDL particles, and an increase of their apoE and apoAI but not apoB content. A synergistic increase of liver PLTP might have contributed to the enlargement of the HDL particles. These results indicate that co-administration of PPARα agonists may be helpful in eliminating or reducing a significant unwanted effect of LXRα agonists, hypertriglyceridemia, while it might enhance their beneficial effects on the vasculature by increasing HDL-cholesterol and enlarging the HDL particles. And, importantly, this combined treatment exerts these effects while allowing the increased cholesterol efflux and excretion caused by LXRα agonists. Thus, pharmacologic manipulation of LXRα agonism by PPARα ligands may provide a novel way to exploit the ability of LXR ligands to prevent/treat dyslipidemia and atherosclerosis.
There are additional complexities in the combined actions of LXRα and PPARα systems (Figure 2⇓). First, activation of PPARα induces the expression of the LXRα gene through PPARα response elements located in the promoter of this gene in both hepatocytes and adipocytes (14, 15). Second, some fatty acids and the acidic forms of fibrates both bind LXRα and suppress its transcriptional activity, and function as ligand agonists of PPARα (16–18). Third, LXRα and PPARα may suppress each other’s transcriptional activity by competing for possibly limited amounts of their common heterodimer partner RXR (19, 20). Fourth, LXRα increases its own protein expression through direct activation of its promoter (21). These remarkable complexities in the interaction between the LXRα and PPARα signaling pathways and their target effects might produce unexpected biological actions in humans and other species, especially when LXRα and PPARα agonists are simultaneously administered for the long-term. We know very little about the carcinogenic potential of LXRα agonists, whereas PPARα agonists can act as carcinogenic agents in the rodent liver, possibly through stimulation of H2O2 generation during the β-oxidation of fatty acids (1, 22). Thus, extensive bench, animal, and clinical research is necessary before rational, combined therapeutic regimens using LXRα and PPARα agonists reach wide clinical practice.
Atherosclerosis is a complex multifactorial, multigenic, and environmental disorder with lipid metabolism representing only one, albeit important, aspect (23). The description by Bayer et al. of a successful combined regimen against two rationally selected molecular targets is an excellent example of what will progressively become a common practice of medicine. Multitargeted therapies against multifactorial disorders sounds like polypharmacy, a word that two decades ago was considered anathema. Yet such an approach now appears to be the best way to proceed for diseases as complex and common as the dysmetabolic syndrome (characterized by visceral obesity, insulin resistance, glucose intolerance/diabetes mellitus type 2, dyslipidemia, blood hypercoagulation and/or hypertension), as well as anxiety and depression, autoimmune, inflammatory and allergic disorders, and cancer (24).
Effects of LXRα and PPARα activation on the regulation of lipid metabolism. Note the combined decrease and increase of LDL and HDL cholesterol, respectively, and the opposing actions on triglyceride levels. The end - effect is a highly beneficial anti-atherosclerotic lipid profile with low LDL, high HDL, and normal triglyceride concentrations. ABC, ATP-binding cassette transporter; ACC, acyl coenzyme A carboxylase; apo, apolipoprotein; CETP, cholesterol ester-transfer protein; CYP7A1, cholesterol 7α-hydroxylase; FAS, fatty acid synthase; HDL, high-density lipoprotein; LDL, low-density lipoprotein; LXR, liver X receptor; PLTP, phospholipid transfer protein; PPAR, peroxisome proliferator activator receptor; SCD-1, sterol coenzyme A desaturase-1; SREBP-1c, sterol regulatory element-binding protein-1c; TG, triglyceride.
The complex interactions between the LXRα and PPARα intracellular signaling pathways and their overall effects on cholesterol and triglyceride metabolism. Activation of PPARα induces LXRα , while several PPARα ligands (some fatty acids and the acidic forms of fibrates) suppress LXRα transcriptional activity. LXRα and PPARα may also suppress each other’s transcriptional activity by competing for their common heterodimer partner RXR, while LXRα regulates its own expression in an ultrashort positive feedback loop. Chol, cholesterol; LXRα, liver X receptor α; PPARα, peroxisome proliferators activator receptor α; RXR, retinoid X receptor; TG, triglycerides.
- © American Society for Pharmacology and Experimental Theraputics 2004
References
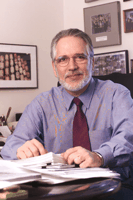
George P. Chrousos, MD, is Chief of the Pediatric and Reproductive Endocrinology Branch, National Institute of Child Health and Human Development, National Institutes of Health. Dr. Chrousos’s laboratory focuses on translational research regarding the physiologic and cellular interactions of the neuroendocrine and immune systems, and their relevance for human disease. His group has defined basic mechanisms and clinical implications of molecular stress mediators that have led to new concepts and novel diagnostic and therapeutic strategies. Please address correspondence to GPC. E-mail chrousog{at}mail.nih.gov; fax 301-402-0884.
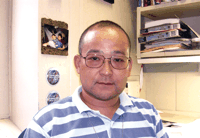
Tomoshige Kino, MD, PhD, is a Staff Scientist at the Pediatric and Reproductive Endocrinology Branch, National Institute of Child Health and Human Development, National Institutes of Health. His research focuses on the understanding of the mechanisms of action of nuclear hormones, the influences of these hormones on metabolic and immune functions, and their involvement in human physiology and disease.