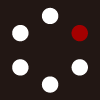
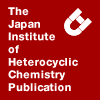
HETEROCYCLES
An International Journal for Reviews and Communications in Heterocyclic ChemistryWeb Edition ISSN: 1881-0942
Published online by The Japan Institute of Heterocyclic Chemistry
e-Journal
Full Text HTML
Received, 14th September, 2010, Accepted, 4th November, 2010, Published online, 11th November, 2010.
DOI: 10.3987/COM-10-S(E)124
■ Design and Synthesis of Photocleavable Biotinylated-Dopamine with Polyethyleneoxy Photocleavable Linkers
Kengo Hanaya, Yoshiyuki Kageyama, Masanori Kitamura, and Shin Aoki*
Faculty of Pharmaceutical Sciences, Tokyo University of Science, 2641 Yamazaki, Noda, Chiba 278-8510, Japan
Abstract
Previously, we reported the synthesis of a biotin–dopamine conjugate (Btn–DA) with a photocleavable 8-quinolinyl benzenesulfonate (QB) linker for the isolation of an intact complex of DA and anti-DA antibody (IgG1) (DA–IgG1 complex). In this work, we synthesized a photocleavable Btn–DA conjugate with a polyethyleneoxy linker to improve the complexation efficiency and the recovery yields of DA–IgG1 complex. The results of QCM, ELISA, and Western blot analyses indicate that the introduction of polyethyleneoxy linkers improved the efficiency of DA–IgG1 complexation.INTRODUCTION
The isolation and identification of specific ligands and receptors of biologically active ligands such as proteins, peptides, and drugs are very important operations in the biological and medicinal sciences. The most popular method in these manipulations is affinity column chromatography that uses affinity resins, to which ligands are bound to trap specific receptor molecules. In this technique, avidin proteins (“avidin”)-coated resins and ligands modified with (+)-biotin (Btn) are often used (“avidin” includes three species of avidin proteins, as described below), because they form extraordinary stable complexes (Kd values ≈ on the order of fM).1 Due to the very stable “avidin”–Btn complexation, the separation of target molecules from the affinity matrix requires harsh denaturing conditions, such as treatment with detergent, high or low pH, high salt concentrations, and other similar conditions. To date, various attempts to obtain intact target receptors and/or ligand–receptor complexes (including Btn derivatives,2 avidin mutant,3 and chemically,4 enzymatically5 or photochemically6a—c cleavable Btn linkers) have been reported.
We recently reported that 8-quinolinyl benzenesulfonates (QB) (general structure: 1) undergo photolysis upon UV irradiation at 300—330 nm in aqueous solution at neutral pH to give the corresponding 8-quinolinol 2 and benzenesulfonates 3 with very small amounts of byproducts.7 Mechanistic investigations have suggested that this photolytic reaction proceeds mainly via homolytic cleavage of the S–O bond in the excited triplet state (Scheme 1).8
We applied this photochemical cleavage reaction of QB to a photocleavable linker. We designed and synthesized a biotin–dopamine (Btn–DA) conjugate with a QB linker 4 (Figure 1). Complexation of 4 with anti-DA antibody (IgG1) on an “avidin”-coated surface and the photochemical release of 4–IgG1 complex were confirmed by 27-MHz quartz-crystal microbalance (QCM) experiments and enzyme-linked immunosorbent assay (ELISA).9 However, the efficiency of the recovery of IgG1 using 4 was not so high. Because we assumed that steric hindrance between “avidin” and IgG1 may hamper the efficient complexation of the DA part of 4 with IgG1, we designed and synthesized 5, which has longer linkers than those of 4 (Figure 1) in this work. We also compared the complexation of 5 with IgG1 and the recovery efficiency of the photorelease of IgG1 to those of 4 by QCM, ELISA, and Western blot analyses.
RESULTS AND DISCUSSION
Synthesis of biotinylated dopamine 5 with a polyethyleneoxy linker
The synthesis of 5 was carried out similarly to that of 4 (Scheme 2).9 (+)-Biotin 6 was reacted with N-Boc-1,8-bis(aminomethyl)-3,6-dioxaoctane 710 to give 8,11 the Boc group of which was deprotected by treatment with trifluoroacetic acid (TFA) to afford 9. The treatment of 9 with 1012 gave 11. For the alkyne part, N-Fmoc-ε-aminocaproic acid 1213 was reacted with propargylamine 13 to give 14, the Fmoc group of which was deprotected (piperidine, THF) to afford 15. The reaction of 15 with 4-(chlorosulfonyl)phenylisocyanate 16 gave 17,14 which was successively reacted with 11 to yield 18. Huisgen 1,3-dipolar [3+2] cycloaddition of the terminal alkyne group of 18 with 199 in the presence of a catalytic amount of Cu2+ and sodium ascorbate according to the procedures of click chemistry15 gave 20 in moderate yields. Finally, deprotection of the TBDMS groups of 20 by treatment with HCl/dioxane gave 5 as the HCl salt.
Evaluation of the complexation properties of 4 and 5 with neutravidin (Nevn) and anti-DA antibody (IgG1) using a 27-MHz quartz-crystal microbalance (QCM)
The complexation of 4 and 5 with “avidin” was studied by means of a 27-MHz quartz-crystal microbalance (QCM), as we previously reported.16 “Avidin” proteins are classified into three categories, avidin (Avn) isolated from egg white, neutravidin (Nevn), which is deglycosylated form of avidin,1g and streptavidin (Stvn) isolated from the bacterium Streptmyces avidinii.1 It is described that the Kd values for the complexes of these “avidin” species with biotin are almost equal.1a,1f,1g In this QCM titrations, Nevn was used to minimize nonspecific binding. The complexation constants, Ks1, of 4 and 5 with Nevn were determined to be 10(8.2 ± 0.2) M-1 and 10(7.6 ± 0.5) M-1, respectively. The Ks1 value for photocleavable biotin linker 18, which lacks the DA part, was determined to be 10(8.1 ± 0.2) M-1, indicating that the introduction of DA to the biotin linker has a negligible effect on the Ks1 value. In addition, anti-DA antibody (IgG1) was added to the Nevn–4 and Nevn–5 complexes to obtain the QCM titration curves, from which the Ks2 values for complexation of the Nevn–4 and Nevn–5 complexes with IgG1 were estimated to be 10(7.5 ± 0.3) M-1 and 10(7.1 ± 0.9) M-1, respectively.
Verification of the complexation of 5 with anti-dopamine antibody and comparison to that of 4 by enzyme-linked immunosorbent assay (ELISA)
The complexation of 5 with IgG1 was compared with that of 4 by ELISA (Scheme 3).9 In these experiments, commercially available Stvn-coated 96-well plates (21) were used to react with 4 or 5 to prepare Stvn–4 (or 5) complexes in the wells (22), to which IgG1 was added to obtain the ternary complex 23. In Scheme 3, Stvn and Avn that have tetrameric structures are illustrated as a monomer form for simplicity. After 23 was washed with phosphate-buffered saline containing 0.05% (v/v) Tween 20 (PBST), the 22–IgG1 complex 23 was treated with an anti-IgG antibody conjugated with horseradish peroxidase (secondary IgG–HRP conjugate, 2nd IgG–HRP) to give 24, as indicated on the left half of Scheme 3. HRP-catalyzed oxidative dimerization of o-phenylenediamine (OPD) using H2O2 gave 2,3-diaminophenazine, which has an absorption maximum at 450 nm (OD450).17 Figure 2 shows the increases in the OD450 values with increasing concentrations of 4 and 5, which strongly suggests the formation of quaternary complex 24 (Stvn–4 (or 5)–IgG1–2nd IgG–HRP). Note that larger OD450 values were observed with 5 than with 4, which suggests that the complexation with IgG1 was somewhat improved and/or the rate of the enzymatic reaction was enhanced possibly by reduction of the steric hindrance around HRP.
Photoreaction of the complexes of 4 or 5 with anti-dopamine antibody (IgG1) on avidin (Avn)-beads, examined by ELISA and Western blot analysis.
Photocleavage of the 5–IgG1 complex in Stvn-coated 96-well plates was carried out by photoirradiation at 313 nm using a 500 W high pressure mercury-vapor lamp (USHIO), as shown on the right half of Scheme 3. After irradiation of the ternary complex (23→25 in Scheme 3), the amount of remaining 23 was analyzed by ELISA. As shown in Figure 3, OD450 decreased with an increase in the duration of photoirradiation, suggesting that the DA–IgG1 complex is released from the wells in a similar efficiency as that of 4.
The photoreaction products 26 (Scheme 3) were analyzed by Western blotting. In this experiment, Avn-agarose (Avn-coated) beads were used as the solid phase instead of Stvn-coated 96-well plates. Lane 1 is the sample obtained by treatment of the 4 (or 5)–IgG1 complex on Avn-agarose beads with SDS without photoirradiation. Lanes 2 and 3 show that the amount of photoreleased DA–IgG1 complex depends on the duration of photoirradiation (lane 2, 0 min; lane 3, 30 min), and the amounts of photoreleased DA–IgG1 with 4 and 5 were almost identical, which agreed fairly well with the results of ELISA shown in Figure 3. Comparison of the products after photoreaction (Lane 3 in Figure 4a and b) and the products after SDS treatment without photoirradiation (Lane 1 in Figure 4a and b) indicated that the recovery efficiencies of DA–IgG1 from 4 and 5 were almost the same.
Discussion about the amount of isolated IgG1 using these data is not easy, because these experiments may give only qualitative data, due to the use of polyclonal anti-IgG1 antibody in ELISA and Western blotting and the signals in these experiments are amplified by enzymatic reaction of HRP. In addition, the discrepancy between the results of ELISA and Western blotting may be due to the use of different solid phases and detection methods in two methods (Stvn-coated 96-wells plates for ELISA and avidin-coated beads for Western blotting). For instance, the results of our recent experiments suggested that chemical properties and/or shapes of solid phase may affect the photoreactivity of QB linker (details will be reported elsewhere).
CONCLUSION
In this report, we described the design and synthesis of a biotinylated dopamine 5 that contained photocleavable linkers that are longer than those of 4. The photocleavable biotin-linker can be easily incorporated into target molecules using a Huisgen 1,3-dipolar [3+2] cycloaddition or other coupling reactions. We found that the efficiency of complexation of 5 with DA–IgG1 was somewhat greater than that of 4 and the photoreactivities of the complexes of 4 and 5 with IgG1 were almost identical. These methods may provide effective strategies for the recovery of intact ligand–receptor complexes under mild conditions, without the need for damaging chemical reagents. The isolation of dopamine receptors using this methodology is now under way.
EXPERIMENTAL
All reagents and solvents purchased were of the highest commercial quality and used without further purification. All aqueous solutions were prepared using deionized and distilled water. IR spectra were recorded on a Horiba FTIR-710 spectrophotometer at room temperature. 1H (300 MHz) and 13C (75 MHz) NMR spectra were recorded on a JEOL Always 300 spectrometer. Thin-layer (TLC) and silica gel column chromatography were performed using Merck 5554 (silica gel) TLC plates and Fuji Silysia Chemical FL-100D, respectively.
Compound 8: A solution of (+)-biotin 6 (55 mg, 0.227 mmol), N-Boc-1,8-bis(methylamino)-3,6-dioxaoctane 710 (69 mg, 0.250 mmol) and 1-ethyl-3-(3-dimethylaminopropyl)carbodiimide hydrochloride (EDC·HCl) (57 mg, 0.235 mmol) in a mixture of MeOH and MeCN (1:3) (20 mL) was stirred for 6 h at room temperature and the reaction mixture was concentrated under reduced pressure. The residue was resuspended in MeOH and filtered through celite (No. 545). The filtrate was concentrated under reduced pressure and the residue was purified by silica gel column chromatography (CH2Cl2/MeOH, 10:1) to yield 8 as a pale yellow oil (103 mg, 90% yield). IR (KBr) 3264, 3073, 2974, 2929, 2867, 1698, 1638, 1458, 1396, 1161, 1119 cm-1; 1H NMR (CDCl3) δ = 1.45 (10H, s), 1.67—1.78 (4H, m), 2.32—2.42 (2H, m), 2.74 (1H, d, J = 12.8 Hz), 2.88—3.07 (7H, m), 3.18 (1H, dt, J = 4.8, 4.8 Hz), 3.39 (2H, br), 3.48—3.67 (10H, m), 4.32 (1H, m), 4.46 (1H, m). 13C NMR (CDCl3) δ = 24.8, 25.2, 28.2, 28.3, 28.4, 28.5, 32.4, 33.0, 33.7, 33.9, 37.1, 40.5, 47.7, 49.7, 51.6, 55.4, 55.5, 60.1, 61.8, 61.9, 69.0, 69.6, 70.3, 70.8, 79.4, 163.6, 173.0, 173.4, 174.1.
Compound 11: TFA (0.2 mL) was added to a solution of 8 (100 mg, 0.199 mmol) in CH2Cl2 (3 mL) and the mixture was stirred for 3 h at room temperature. After the reaction mixture was concentrated under reduced pressure, the resulting residue was azeotroped with toluene to remove TFA. The residue was dissolved in CHCl3 and the solution was washed with 1 N aqueous NaOH. After the organic layers were dried over Na2SO4, the solvent was filtered and concentrated under reduced pressure to yield crude 9 as a colorless oil (79 mg, 98% crude yield). IR (KBr) 3298, 3092, 2923, 2863, 1702, 1636, 1459, 1117 cm-1; 1H NMR (CDCl3) δ = 1.44—1.52 (2H, m), 1.63—1.76 (4H, m), 2.33—2.44 (2H, m), 2.45 (2H, s), 2.72—2.78 (3H, m), 2.89—3.07 (3H, m), 3.15—3.19 (1H, m), 3.47—3.65 (10H, m), 4.30—4.34 (1H, m), 4.49—4.51 (1H, m).
Et3N (42 μL, 0.3 mmol) was added to a solution of crude 9 in CH2Cl2 (8 mL) at 0 °C under an argon atmosphere, and then a solution of 10 (39 mg, 0.150 mmol)12 in CH2Cl2 (2 mL) was added dropwise. The reaction mixture was stirred for 30 min at 0 °C and overnight at room temperature. The solvent was removed under reduced pressure and the remaining residue was purified by silica gel column chromatography with a CH2Cl2-MeOH gradient (1:0, 50:1, 30:1 v/v) as solvent to yield 11 as a yellow amorphous solid (81 mg, 87% yield). IR (KBr) 3422, 2928, 2867, 1702, 1636, 1325, 1175, 1115 cm-1; 1H NMR (CDCl3) δ = 1.45—1.47 (2H, m), 1.65—1.70 (4H, m), 2.32—2.39 (2H, m), 2.72 (1H, d, J = 13.1 Hz), 2.76 (3H, s), 2.80 (3H, s), 2.90—3.05 (4H, m), 3.17—3.19 (1H, m), 3.34 (2H, t, J = 5.7 Hz), 3.46—3.68 (8H, m), 4.33—4.35 (1H, m), 4.53 (1H, m), 7.15 (1H, d, J = 8.2 Hz), 7.48 (1H, d, J = 8.8 Hz), 8.08 (1H, d, J = 8.2 Hz), 8.91 (1H, d, J = 8.8 Hz); 13C NMR (CDCl3) δ = 24.7, 24.7, 25.1, 28.2, 28.3, 30.3, 32.3, 32.9, 33.8, 35.6, 35.6, 37.0, 40.5, 47.6, 49.0, 49.6, 55.3, 60.1, 61.7, 68.8, 69.4, 69.7, 70.1, 70.4, 70.7, 108.0, 123.3, 123.3, 124.4, 131.5, 134.3, 137.6, 156.2, 157.9, 163.3,172.9, 173.3; MS (FAB+) m/z 624.2526 (calcd for C28H42N5O7S2: 624.2520).
Compound 15: EDC·HCl (141 mg, 0.736 mmol) and propargylamine 13 (31 mg, 0.566 mmol) were added to a solution of 1213 (200 mg, 0.566 mmol) in a 1/1 mixture of MeOH and MeCN (3 mL). The reaction mixture was stirred overnight at room temperature and the solvent was removed under reduced pressure. The remaining residue was resolved in CHCl3 and the solution was washed with water, dried over Na2SO4, and filtered. After the filtrate was concentrated under reduced pressure, the resulting residue was purified by silica gel chromatography (CHCl3) to yield 14 as a colorless solid (209 mg, 94% yield). 1H NMR (CDCl3) δ = 1.25—1.38 (2H, m), 1.52—1.56 (2H, m), 1.67—1.70 (2H, m), 2.18—2.21 (3H, m), 3.20 (2H, td, J = 6.4, 6.4 Hz), 4.50 (2H, dd, J = 2.6, 5.3 Hz), 4.21 (1H, t, J = 7.0 Hz), 4.40(2H, d, J = 7.0 Hz), 7.31 (2H, dd, J = 7.3, 7.3 Hz), 7.40 (2H, dd, J = 7.3, 7.3 Hz), 7.59 (2H, d, J = 7.3 Hz), 7.77 (2H, d, J = 7.3 Hz).
Piperidine (600 μL) was added to a solution of 14 (90 mg, 0.230 mmol) in THF (3 mL) and the reaction mixture was stirred for 1 h at room temperature. After the solvent was removed under reduced pressure, the resulting solid was resuspended in Et2O and filtered to yield 15 (40 mg, quant) as a colorless solid. IR (neat) 3290, 2931, 2859, 1637, 1541 cm-1; 1H NMR (CDCl3) δ = 1.32—1.50 (4H, m), 1.67 (2H, tt, J = 7.5, 7.5 Hz), 2.19—2.24 (3H, m), 2.70 (2H, t, J = 6.8 Hz), 4.06 (2H, dd, J = 2.6, 5.1 Hz); 13C NMR (CD3OD) δ = 26.5, 27.3, 29.4, 32.2, 36.6, 72.0, 80.7, 175.7; MS (FAB+) m/z 169.1342 (calcd for C9H17N2O: 169.1335) .
Compound 18: Compound 15 (39 mg, 0.230 mmol) was added to a solution of 4-(chlorosulfonyl)phenyl isocyanate 16 (50 mg, 0.230 mmol) in THF (3 mL). The reaction mixture was stirred for 3 h at room temperature and the solvent was removed to give crude 17 as a yellow solid. A solution of crude 17 (18 mg) in THF (0.5 mL) and 4-N,N-dimethylaminopyridine (2 mg) was added to a mixture of 11 (29 mg, 0.047 mmol) and diisopropylethylamine (6 mg, 0.048 mmol) in CH2Cl2 (2 mL) at 0 °C, and the reaction mixture was stirred overnight at reflux. After the solvent was removed, the remaining residue was dissolved in CHCl3 and washed with water. The organic layer was dried over Na2SO4, filtered, and concentrated under reduced pressure. The resulting residue was purified by silica gel column chromatography with a CHCl3-MeOH gradient (1:0, 30:1, 25:1, 20:1, 13:1 v/v) to yield 18 as a colorless amorphous solid (22 mg, 48% yield based on 11). IR (KBr) 3427, 3266, 2928, 2861, 1697, 1634, 1372, 1321, 1223, 1169, 1142, 1112 cm-1; 1H NMR (CDCl3) δ = 1.25—1.67 (10H, m), 2.19—2.24 (3H, m), 2.31 (2H, m), 2.63 (3H, s), 2.76 (1H, d, J = 13.0 Hz), 2.84—3.00 (7H, m), 3.15—3.24 (3H, m), 3.37—3.54 (12H, m), 4.33 (1H, m), 4.53 (1H, m), 7.37 (1H, d, J = 8.9 Hz), 7.49—7.63 (3H, m), 7.69—7.76 (2H, m), 8.08 (1H, d, J = 8.4 Hz), 8.83 (1H, d, J = 8.9 Hz); 13C NMR (CDCl3) 24.6, 25.0, 25.3, 26.2, 27.6, 27.8, 29.1, 29.3, 29.7, 31.0, 32.5, 36.0, 36.9, 39.4, 40.5, 47.5, 49.2, 55.3, 60.3, 61.7, 68.6, 69.0, 69.1, 70.1, 70.2, 70.5, 71.5, 79.6, 117.0, 120.7, 120.9, 124.1, 127.8, 130.2, 133.3, 133.4, 133.6, 141.5, 146.3, 148.8, 155.3, 160.7, 163.6, 173.1, 173.3, 173.5. MS (FAB+) m/z 973.3619 (calcd for C44H61N8O11S3: 973.3622).
Compound 20: Sodium ascorbate (1.0 μmol, 10 μL of a 100 mM solution in water) and CuSO4·5H2O (0.5 μmol, 5 μL of a 100 mM solution in water) were added to a suspension of 18 (10 mg, 10 μmol) and 199 (5 mg, 10 μmol) in a 1/1 mixture of water and THF (1mL). The reaction mixture was stirred overnight at room temperature, and the solvent was removed under reduced pressure. After the remaining residue was resuspended in CHCl3, the solution was washed with water, dried over Na2SO4, filtered, and concentrated under reduced pressure. The resulting residue was purified by silica gel column chromatography with a CH2Cl2-MeOH gradient (15:1, 13:1, 10:1 v/v) to yield 20 as a pale yellow amorphous (10 mg, 63% yield). 1H NMR (CDCl3) δ = 0.18 (12H, s), 0.94 (18H, s), 1.33—1.70 (12H, m), 2.21—2.32 (4H, m), 2.61 (3H, s), 2.73—2.76 (1H, m), 2.85—2.98 (11H, m), 3.12 (1H, m), 3.21 (2H, td, J = 5.9, 11.8 Hz), 3.38—3.54 (14H, m), 4.53—4.54 (3H, m), 6.64 (1H, d, J = 8.0 Hz), 6.68 (1H, s), 6.74 (1H, d, J = 8.0 Hz), 7.35 (1H, d, J = 8.9 Hz), 7.43 (2H, d, J = 7.8 Hz), 7.49 (2H, d, J = 9.0 Hz), 7.56—7.61 (3H, m), 7.71 (2H, d, J = 9.0 Hz), 8.00 (1H, s), 8.07 (1H, d, J = 8.3Hz), 8.83 (1H, d, J = 8.9 Hz).
Compound 5: 4N HCl in dioxane (57 μL, 0.228 mmol) was added to a solution of 20 in a 1/1 mixture of MeOH and THF (1 mL), and the reaction mixture was stirred overnight at room temperature. After the solvent was removed under reduced pressure, the remaining residue was purified by silica gel column chromatography with a CHCl3-MeOH gradient (5:1, 4:1, 3:2 v/v) to yield 5 as a colorless amorphous solid (8 mg, 96% yield). IR (KBr) 3422, 2969, 2932, 2871, 2819, 1685, 1611, 1373, 1325, 1170, 1057, 1033, 1015 cm-1; 1H NMR (CD3OD) δ = 1.37—1.68 (12H, m), 2.26 (2H, t, J = 7.6 Hz), 2.38 (2H, m), 2.56 (3H, s), 2.65 (1H, dd, J = 5.9 Hz), 2.84—3.06 (10H, m), 3.22—3.34 (3H, m), 3.39 (2H, m), 3.46—3.63 (12H, m), 4.27 (3H, m), 4.44 (1H, m), 4.56 (2H, s), 6.58 (1H, d, J = 8.0 Hz), 6.68 (1H, s), 6.73 (1H, d, J = 8.0 Hz), 7.43 (1H, d, J = 8.9 Hz), 7.48 (1H, d, J = 9.1Hz), 7.66 (2H, d, J = 8.4 Hz), 7.67 (2H, d, J = 8.9 Hz), 7.76 (1H, d, J = 8.0 Hz), 7.93 (2H, d, J = 8.4 Hz), 8.15 (1H, d, J = 8.0Hz), 8.43 (1H, s), 8.87 (1H, d, J = 9.1 Hz). MS (FAB+) m/z 1257.4893 (Calcd for C59H77N12O13S3: 1257.4895).
Determination of Ks values for 4 and 5 with Nevn and anti-DA IgG1 by 27-MHz quartz-crystal microbalance (QCM) analysis: QCM experiments were performed on an Affinix-Q4 apparatus (Initium Inc., Japan).16 In QCM experiments, Nevn was used to minimize nonspecific binding. A clean Au (4.9 mm2) electrode on a quartz crystal was incubated with an aqueous solution of 3,3′-dithiodipropionic acid (3 mM) at room temperature for 45 min. The surfaces were activated by treatment with a mixture of EDC·HCl (0.26 M) and N-hydroxysuccinimide (0.44 M) for 45 min. The chip was allowed to stand to reach equilibrium at 25 °C in 500 μL phosphate-buffered saline (PBS). Next, 5 μL of Nevn solution (1 mg/mL) was injected and the change in the frequency of the quartz oscillator was recorded at specific time points. After 1 μL of an aqueous solution of ethanolamine (1 M) was added to block the remaining activated groups, PBS was replaced with PBST. For the immobilization of Nevn–4 or 5, 1 μL of an aqueous solution of 4 or 5 (0.5 μg/mL) was injected at several time points and the change in frequency was recorded. Based on the fact that a frequency decrease (ΔF) of 1 Hz corresponds to a mass increase of 30 pg on the electrode (0.049 cm2), Ks1 values were determined from the relationship between the decrease in frequency and the time for equilibration. To determine the Ks2 values for Nevn–4 or –5 complexes and IgG1, Nevn–4 or –5 complexes, which had been prepared beforehand, was immobilized on a Au electrode. 1 μL of an aqueous solution of IgG1 was injected at several time points and Ks2 values were determined as described above.
ELISA: A monoclonal anti-dopamine antibody (IgG1) (Abcam) was used as a primary antibody. A streptavidin-immobilized 96-well plate was purchased from Nunc Co. Ltd and washed three times with phosphate-buffered saline containing 0.05% (w/v) Tween 20 (PBST) prior to use. The biotinylated dopamine 4 or 5 diluted with PBST was added at 100 μL/well, and the plates were then incubated at room temperature for 1 h. After being washed with PBST, the 4 or 5 in each well was sequentially incubated at room temperature for 1 h with IgG1 (1:2500 dilution) and 2nd IgG–HRP conjugate (1:1000 dilution), and the plates were incubated for 1 h at room temperature. After the plates were washed, OPD and H2O2 were added to each well. After incubation at room temperature for 15 min, the absorbance at 450 nm was measured on a Bio-Rad model 550 microplate reader (Bio-Rad, Hemel, Hempstead, UK).
Photoreaction for the release of DA–IgG1 complex: Stvn-coated wells were treated with 4 or 5 and IgG1 as described above and photoirradiated at 313 nm using a USHIO Optical Module X equipped with a high pressure mercury-vapor lamp (USHIO), a UV-transmitting and visible-absorbing filter (U-330, Kenko, Co. Ltd., Japan), and an optical filter (HQBP313-UV, Asahi Spectra, Co. Ltd., Japan). After irradiation for defined durations, the wells were washed with PBST (100 μL) and 2nd IgG–HRP (diluted 1:1000 with PBST) was added to the wells. After the samples were incubated at room temperature and washed, OPD and H2O2 were added and the absorbance was measured at 450 nm on a Bio-Rad model 550 microplate reader (Bio-Rad, Hemel, Hempstead, UK).
Western blotting: Avidin–agarose (Avn-coated) beads (Sigma-Aldrich) were reacted with 4 or 5 in PBST at room temperature for 2 h, washed three times with PBST, and then reacted with IgG1 at room temperature for 1 h. After the resulting avidin beads were washed three times with PBST, the beads were resuspended in 0.5 mM Tris–HCl buffer and then transferred to a 24-well plate. These suspensions were photoirradiated at 313 nm as described above to release DA–IgG1 complexes. The resulting mixture was filtered through DISMIC-13HP PTFE (0.45 μm, ADVANTEC) to remove the beads. The precipitate was washed with 5 mM Tris-HCl buffer and the combined filtrate was freeze-dried. The resulting materials were dissolved in a mixture of 10% SDS (8 μL), glycerol (4 μL), and water (8 μL), loaded on 15% SDS-polyacrylamide gel for electrophoresis (20 μL/lane), and then transferred to a PVDF membrane (Immobilon-P Transfer Membrane, Millipore) under wet conditions. The membrane was blocked with 3% bovine serum albumin (BSA, Sigma) and incubated with 2nd IgG1–HRP diluted with PBST for 1 h at room temperature. The membrane was rinsed with Tris-buffered saline containing 0.05% (w/v) Tween20 (5 minutes × 3) and visualized using ECL Plus Western blotting detection reagents on a LAS 3000 lumino-image analyzer (FUJIFILM).
ACKNOWLEDGEMENTS
This study was supported by grants-in-aid from the Ministry of Education, Culture, Sports, Science and Technology (MEXT) of Japan (No. 18390009, 19659026, 22390005, and 22659005) and the “Academic Frontier” project for private universities: matching fund subsidy from MEXT, 2009—2013. M. K. is also thankful for a Sasakawa Scientific Research Grant from the Japan Science Society.
References
†. Faculty of Pharmaceutical Sciences, Tokyo University of Science; Center for Technologies against Cancer, Tokyo University of Science.
1. (a) 'Methods in Enzymology: Avidin Biotin Technology,' ed. by M. Wilchek and E. A. Bayer, Academic Press, Inc., San Diego, 1990; (b) 'A Laboratory Guide to Biotin-Labeling in Biomolecule Analysis,' ed. by T. Meier and F. Fahrenholz, Birkhauser, Boston, 1996; (c) 'Biotin,' Icon Group Intl., Inc., San Diego, 2004; (d) K. Hofmann and Y. Kiso, Proc. Natl. Acad. Sci. U.S.A., 1976, 73, 3516; CrossRef (e) M. Wilchek and E. A. Bayer, Anal. Biochem., 1988, 171, 1; CrossRef (f) E. P. Diamandis and T. K. Christpoulos, Clin. Chem., 1991, 37, 625; (g) Y. Hiller, J. Gershoni, E. A. Bayer, and M. Wilchek, Biochem. J., 1987, 248, 167.
2. (a) K. Hofmann, G. Titus, J. A. Montibeller, and F. M. Finn, Biochemistry, 1982, 21, 978; CrossRef (b) K. Hofmann, W. J. Zhang, H. Romovacek, F. M. Finn, A. A. Bothner-By, and P. K. Mishra, Biochemistry, 1984, 23, 2547; CrossRef (c) J. D. Hirsch, L. Eslamizar, B. J. Filanoski, N. Malekzadeh, R. P. Haugland, J. M. Beechem, and R. P. Haugland, Anal. Biochem., 2002, 308, 343; CrossRef (d) B. Fudem-Goldin and G. A. Orr, Methods Enzymol., 1990, 184, 167. CrossRef
3. (a) G. O. Reznik, S. Vajda, T. Sano, and C. R. Cantor, Proc. Natl. Acad. Sci. U.S.A., 1998, 95, 13525; CrossRef (b) K. P. Henrikson, S. H. G. Allen, and W. L. Maloy, Anal. Biochem., 1979, 94, 366. CrossRef
4. (a) M. Shimkus, J. Levy, and T. Herman, Proc. Natl. Acad. Sci. U.S.A., 1985, 93, 2593; CrossRef (b) G. A. Soukup, R. L. Cerny, and L. J. Maher, Bioconjugate Chem., 1995, 6, 135; CrossRef (c) Y. -S. Kang, K. Voigt, and U. J. Bickerl, Drug Targeting, 2000, 8, 3425; (d) W.-C. Lin and T. H. Morton, J. Org. Chem., 1991, 56, 6850; CrossRef (e) P. van der Veken, E. H. C. Dirksen, E. Ruijter, R. C. Elgersma, A. J. R. Heck, D. T. S. Rjiker, M. Slijper, and R. M. L. Liskamp, ChemBioChem, 2005, 6, 2271. CrossRef
5. S. Sato, Y. Kwon, S. Kamisuki, N. Srivastava, Q. Mao, Y. Kawazoe, and M. Uesugi, J. Am. Chem. Soc., 2007, 129, 873. CrossRef
6. (a) J. Olejnik, S. Sonar, E. Krzymanska-Olejnik, and K. J. Rothschild, Proc. Natl. Acad. Sci. U.S.A., 1995, 92, 7590; CrossRef (b) M. W. Pandori, D. A. Hobson, J. Olejnik, E. Krzymanska-Olejnik, K. J. Rothchild, A. A. Palmer, T. J. Phillips, and T. Sano, Chem. Biol., 2002, 9, 567; CrossRef (c) J. Olejnik, E. Krzymanska-Olejnik, and K. J. Rothchild, Nucl. Acids Res. 1996, 24, 361; CrossRef (d) J. J. Park, Y. Sadakane, K. Masuda, T. Tomohiro, T. Nakano, and Y. Hatanaka, ChemBioChem, 2005, 6, 814. CrossRef
7. (a) S. Aoki, K. Sakurama, R. Ohshima, N. Matsuo, Y. Yamada, R. Takasawa, S. Tanuma, and K. Takeda, Inorg. Chem., 2008, 47, 2747; CrossRef (b) R. Ohshima, M. Kitamura, A. Morita, M. Shiro, Y. Yamada, M. Ikekita, E. Kimura, and S. Aoki, Inorg. Chem., 2010, 49, 888. CrossRef
8. Y. Kageyama, R. Ohshima, K. Sakurama, Y. Fujiwara, Y. Tanimoto, Y. Yamada, and S. Aoki, Chem. Pharm. Bull., 2009, 57 1257. CrossRef
9. S. Aoki, N. Matsuo, K. Hanaya, Y. Yamada, and Y. Kageyama, Bioorg. Med. Chem., 2009, 17, 3405. CrossRef
10. (a) R. J. Amir, N. Pessah, M. Shamits, and D. Shabat, Angew. Chem. Int. Ed., 2003, 42. 4494; CrossRef (b) T. M. Fyles and C. Hu, J. Supramol. Chem., 2003, 1, 207. CrossRef
11. A. Eiseführ, S. P. Arora, G. Sengle, R. L. Takaoka, S. J. Nowick, and M. Famulok, Bioorg. Med. Chem., 2003, 11, 235. CrossRef
12. (a) D. A. Pearce, N. Jotterand, I. S. Carrico, and B. Imperiali, J. Am. Chem. Soc., 2001, 123, 5160; CrossRef (b) S. Aoki, K. Sakurama, N. Matsuo, Y. Yamada, R. Takasawa, S. Tanuma, M. Shiro, K. Takeda, and E. Kimura, Chem. Eur. J., 2006, 12, 9066. CrossRef
13. A. Paquet, Can. J. Chem., 1982, 60, 976. CrossRef
14. J. Leban, S. Pegoraro, M. Dormeyer, M. Lanzer, A. Aschenbrenner, and B. Kramer, Bioorg. Med. Chem. Lett., 2004, 14, 1979. CrossRef
15. (a) H. C. Kolb and K. B. Sharpless, Drug Discov. Today, 2003, 24, 1128; CrossRef (b) V. D. Bock, H. Hiemstra, and J. H. van Maarseveen, Eur. J. Org. Chem., 2006, 51; CrossRef (c) W. G. Lewis, L. G. Green, F. Grynszpan, Z. Radić, P. R. Carlier, P. Taylor, M. G. Finn, and K. B. Sharpless, Angew. Chem. Int. Ed., 2002, 41, 1053; CrossRef (d) V. V. Rostovtsev, L. G. Green, V. V. Fokin, and K. B. Sharpless, Angew. Chem. Int. Ed., 2002, 41, 2596. CrossRef
16. (a) Y. Okahata, Y. Matsunobu, K. Ijiro, M. Mukai, A. Murakami, and K. Makino, J. Am. Chem. Soc., 1992, 114, 8299; CrossRef (b) Y. Okahata, M. Kawase, K. Niikura, F. Ohtake, H. Furusawa, and Y. Ebara, Anal. Chem., 1998, 70, 1288; CrossRef (c) H. Nishino, T. Nihira, T. Mori, and Y. Okahata, J. Am. Chem. Soc., 2004, 126, 2264 CrossRef