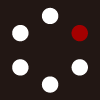
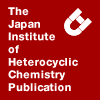
HETEROCYCLES
An International Journal for Reviews and Communications in Heterocyclic ChemistryWeb Edition ISSN: 1881-0942
Published online by The Japan Institute of Heterocyclic Chemistry
e-Journal
Full Text HTML
Received, 17th September, 2010, Accepted, 26th October, 2010, Published online, 11th November, 2010.
DOI: 10.3987/COM-10-S(E)126
■ Regiospecific Rearrangement of Hydroxylamines to Secondary Amines Using Diisobutylaluminum Hydride
Hidetsura Cho,* Kenji Sugimoto, Yusuke Iwama, Nakako Mitsuhashi, Kentaro Okano, and Hidetoshi Tokuyama*
Department of Chemistry, Graduate School of Science, Tohoku University, Aoba-ku, Sendai 980-8578, Japan
Abstract
A systematic investigation of a reductive ring-expansion reaction of N-monosubstituted hydroxylamines with diisobutylaluminum hydride (DIBALH) was carried out. The reaction regiospecifically provided a variety of bicyclic or tricyclic heterocycles or linear secondary amines containing nitrogen attached to an aromatic ring.INTRODUCTION
The development of synthetic methods for unsubstituted basic skeletons of heterocycles is important from the viewpoint of both synthetic chemistry and medicinal chemistry. In particular, the synthesis of bicyclic or tricyclic fused heterocycles containing nitrogen attached to an aromatic ring such as benzazepine, benzoxazine, benzoxazepine, benzthiazine, and benzthiazepine, is essential, because these skeletons often form the core structures of pharmaceuticals or clinical candidates. However, only a few straightforward and versatile synthetic procedures are available to construct the heterocyclic systems.1
Recently, we have reported an investigation of the reductive ring expansion reaction of cyclic ketoximes fused to aromatic rings using diisobutylaluminum hydride (DIBALH) (Scheme 1). 2a,2b The treatment of an E/Z mixture of ketoximes with 5 equiv of DIBALH furnished the corresponding ring expansion product with nitrogen attached to the aromatic ring as the sole isomer. According to preliminary results (Table 1, entry 2) showing that the treatment of the hydroxylamine with DIBALH gave the reductive ring expansion product and theoretical calculations based on the density functional theory (DFT), we proposed a reaction mechanism involving the 1,2-reduction of an oxime to hydroxylamine and a regiospecific rearrangement via a partial phenonium cation intermediate (Scheme 2).2b
It is known that hydroxylamines activated by an electron-withdrawing group on the oxygen and O-alkyl hydroxylamines activated by aluminum agents undergo rearrangement reactions. Hoffman described the rearrangement of O-arylsulfonyl hydroxylamines (Scheme 3, eq 1).3a,b Yamamoto and Maruoka reported a rearrangement-alkylation sequence of hydroxylamine carbonate effected by trialkylaluminum (eq 2).3c The reductive migration of O-methyl hydroxylamine with LAH was reported by Booth (eq 3),3d and the reaction of hydroxylamine with Red-Al was described by Naito and Miyata (eq 4).3e-h In contrast to these examples, no report has appeared on the reductive rearrangement of unfunctionalized hydroxylamines to the best of our knowledge.
Therefore, we carried out a systematic investigation of the rearrangement reaction of hydroxylamine. Herein, we report its scope and generality of the reaction.
RESULTS AND DISCUSSION
First, bicyclic hydroxylamines were synthesized from readily accessible fused bicyclic ketoximes by 1,2-reduction with sodium cyanoborohydride4 under acidic conditions; they were then subjected to reductive conditions with DIBALH (Table 1). Thus, hydroxylamines were treated with 6 equiv of DIBALH at 0 °C to room temperature. The reaction was quenched by adding NaF.1,2 Although the theoretical amount of DIBALH was 3 equiv, we used an excess amount of the reagent to achieve complete consumption of the substrate.
The reductive rearrangement of hydroxylamines was found to be applicable to a variety of bicyclic substrates (Table 1). In all cases, the reaction gave the expected reductive ring expansion product as the sole isomer as observed in the reaction of the corresponding oximes with DIBALH.2 Thus, the aromatic ring migrated to the nitrogen atom to form bicyclic compounds having nitrogen attached to the aromatic ring. Reactions of five- to seven-membered ring substrates 8a, 8b, and 8c provided 1,2,3,4-tetrahydroquinoline 9a, 2,3,4,5-tetrahydro-1H-benz[b]azepine 6, and 1,2,3,4,5,6-hexahydrobenz[b]azocine 9c, respectively (entries 1 to 3). In addition, the reactions were applicable to the synthesis of 2,3,4,5-tetrahydrobenzo[b][1,4]oxazepine 9d and 2,3,4,5-tetrahydrobenzo[b][1,4]thiazepine 9e (entries 4 and 5). Furthermore, a cyclohexyl ring fused to thiophene expanded smoothly to give 5,6,7,8-tetrahydrothieno[3,2-b]azepine 9f, 1a which was isolated as a p-nitrobenzoyl amide 10f owing to the instability of 9f (entry 6).
Although the reaction yields of hydroxylamines with DIBALH are slightly lower than those of the corresponding oximes with the reagent,2b one of the advantages of using hydroxylamine instead of an oxime as a substrate should be its broad synthetic accessibility.5–12 Thus, in addition to the reduction of oximes, hydroxylamines can be prepared from alcohols or primary amines by substitution reactions or oxidation through the regioselective formation of nitrone in a stepwise protocol. For instance, tricyclic hydroxylamine 8g was prepared by the reaction of alcohol 11 with hydroxylamine hydrochloride in the presence of sodium acetate and dichloroacetic acid (Scheme 4).8a,b
On the other hand, N-(9-fluorenyl)hydroxylamine 8h was prepared from primary amine 12 by a stepwise protocol including cyanomethylation, regioselective oxidation to nitrone, and hydroxyaminolysis7c (Scheme 5). The reductive ring expansion reaction of tricyclic hydroxylamines 8g and 8h proceeded smoothly to give the corresponding secondary amines 9g and 9h (Table 2, entries 1 and 2).
Finally, we examined the reaction of linear hydroxylamines, which were easily prepared by reduction of the corresponding ketoximes (Table 3).5 We observed the specific migration of aryl groups providing the corresponding aniline derivatives as the sole product. Thus, the reaction of N-(1-phenylethyl)- hydroxylamine 8i13 and N-(1-phenylpropyl)hydroxylamine 8j afforded N-ethylaniline 9i and N-propylaniline 9j via specific aryl migration (entries 1 and 2). N-Diphenylmethanehydroxylamine 8k14 was also transformed into benzylaniline 9k in high yield (entry 3). The preferential migratory aptitude of an aryl group over that of an alkyl chain of hydroxylamines was also observed in the reaction of oximes with DIBALH.2b
In summary, we have established the reductive rearrangement reaction of hydroxylamines with DIBALH. A systematic investigation demonstrated that the N-monosubstituted hydroxylamines serve as a suitable substrate for the reductive rearrangement reaction. In addition, the reaction gave cyclic or acyclic secondary amines having nitrogen attached to the aromatic rings specifically owing to the preferential migratory aptitude of the aromatic rings. Because hydroxylamines can be easily prepared from a various precursors such as oximes,4–6 primary amines,7 alcohols,8 nitroalkanes,9,10 conjugated nitroalkenes,11 and nitronates,12 the rearrangement reaction of hydroxylamines with DIBALH should be useful and the resulted aromatic amines will likely find use in the field of fine and medicinal chemistry.
EXPERIMENTAL
General
Column chromatography was performed on silica gel 60N (Kanto, 63-210 μm) and flash column chromatography was performed on silica gel 60N (40-60 μm) using the indicated solvents. Reactions and chromatography fractions were monitored by employing pre-coated silica gel 60 F254 plates (0.25 mm). All melting points were uncorrected. IR spectra were measured with a FT-IR spectrometer and were reported in wave numbers (cm-1). NMR spectra were recorded on 400 MHz or 500 MHz spectrometers with tetramethylsilane (0 ppm) or chloroform (7.24 ppm) as the internal standard. Chemical shifts were reported in δ (ppm downfield from tetramethylsilane). Coupling constants were reported in Hz. Mass spectra were obtained using following ionization techniques: electron impact (EI), fast atom bombardment (FAB).
General synthetic procedure of hydroxylamines
From representative oxime derivatives: N-4-chromanylhydroxylamine (8d): To a stirred solution of chroman-4-one oxime (326 mg, 2.00 mmol) in MeOH (4.0 mL) was added NaBH3CN (624 mg, 9.93 mmol) at rt and added hydrogen chloride in MeOH (1.0 M, 6.0 mL) at 0 ºC. After stirring for 1.5 h at rt, the reaction was quenched with saturated aqueous NaHCO3. The reaction mixture was extracted with EtOAc, and the combined extracts were washed with brine, dried over anhydrous Na2SO4, concentrated under reduced pressure. The residue was purified by recrystallization (from EtOAc/n-hexane) to give hydroxylamine 8d (226 mg, 68%) as colorless needles: mp 142-144 °C; IR (KBr) 3258, 3175, 2887, 1491, 1229, 770 cm-1; 1H NMR (400 MHz, CDCl3) δ 7.25 (1H, dd, J = 8.8, 1.6 Hz), 7.19 (1H, ddd, J = 8.8, 6.8, 1.6 Hz), 6.89 (1H, ddd, J = 8.8, 6.8, 0.8 Hz), 6.84 (1H, dd, J = 8.8, 0.8 Hz), 5.86 (1H, br s), 5.25 (1H, br s), 4.28-4.21 (2H, m), 4.10 (1H, dd, J = 3.6, 3.6 Hz), 2.22 (1H, ddd, J = 14.8, 6.8, 3.6 Hz), 2.10-1.97 (1H, m); 13C NMR (100 MHz, CDCl3) δ 155.7, 130.3, 129.5, 120.3, 119.7, 117.2, 62.0, 54.9, 25.2; LRMS (EI) m/z 165 (M+); HRMS m/z Calcd for C9H11NO2 (M+) 165.0790, found 165.0778.
From alcohol derivatives: Preparation of 10,11-dihydro-5-hydroxyamino-5H-dibenzo[a,d]- cyclopentane (8g): To a stirred solution of dibenzosuberone (1.00 g, 4.80 mmol) in MeOH (12.0 mL) was added NaBH4 (545 mg, 14.4 mmol) potionwise at 0 °C. After stirring for 10 min at the same temperature, the reaction was quenched with saturated aqueous NH4Cl and the mixture was extracted with EtOAc. The combined organic extracts were washed with brine, dried over Na2SO4, and concentrated under reduced pressure. The residue was purified by flash column chromatography (SiO2, n-hexane:EtOAc = 85:15) to afford alcohol 11 (1.00 g, quant.) as a colorless oil: IR (neat) 3317, 1483, 1456, 1030 cm-1; 1H NMR (400 MHz, CDCl3) δ 7.44 (2H, d, J = 8.0 Hz), 7.22-7.13 (6H, m), 5.96-5.95 (1H, m), 3.47-3.38 (2H, m), 3.15-3.06 (2H, m), 2.24 (1H, br s); 13C NMR (100 MHz, CDCl3) δ 140.5, 138.9, 130.2, 127.9, 127.0, 126.2, 76.6, 32.4; LRMS (EI) m/z 210 (M+); HRMS m/z Calcd for C15H14O 210.1045, Found 210.1048. To a stirred solution of dichloroacetic acid (4.75 mL, 57.6 mmol), sodium acetate (394 mg, 4.80 mmol) in CH2Cl2 (5.0 mL) was added hydroxylamine hydrochloride (1.33 g, 19.2 mmol) at rt and stirred for 1 h. To a stirred suspension was added dropwise alcohol 11 (1.00 g, 4.80 mmol) in CH2Cl2 (5.0 mL) at rt. After stirring for 20 h at the same temperature, the reaction was quenched with saturated aqueous NaHCO3 at 0 °C and the mixture was extracted with CH2Cl2. The combined extracts were washed with brine, dried over Na2SO4, and concentrated under reduced pressure. The residue was purified by flash column chromatography (SiO2, n-hexane:EtOAc = 85:15) to afford hydroxylamine 8g (899 mg, 83%) as a colorless oil: IR (neat) 3528, 3260, 3061, 3018, 2928, 2887, 760 cm-1; 1H NMR (400 MHz, CDCl3) δ 7.31 (2H, d, J = 7.2 Hz), 7.22-7.13 (6H, m), 5.05 (1H, s), 4.21 (1H, br s), 3.71-3.63 (2H, m), 2.91-2.83 (2H, m) (one proton signal was missing owing to exchanging to deuterium); 13C NMR (100 MHz, CDCl3) δ 141.1, 136.8, 131.3, 130.4, 128.2, 126.0, 74.6, 32.7; LRMS (EI) m/z 193 [(M−NHOH)+]; HRMS m/z Calcd for C15H13 [(M−NHOH)+] 193.1018, Found 193.1022.
From amine derivatives: N-(9H-fluoren-9-yl)hydroxylamine (8h): To a stirred solution of N-(9H-fluoren-9-yl)amine 12 (739.5 mg, 4.08 mmol) in MeCN (8.2 mL) and i-Pr2NEt (1.42 mL, 8.16 mmol) was added bromoacetonitrile (313 µL, 4.49 mmol) dropwise over 15 min. The reaction mixture was stirred at rt for 2 days and evaporated, diluted with CH2Cl2. The organic layer was washed with saturated aqueous NaHCO3, dried over Na2SO4 to give the residue, which was purified by flash column chromatography (SiO2, n-hexane:EtOAc = 3:1) to afford 2-(9H-fluoren-9-yl)aminoacetonitrile 13 (858 mg, 95%). To a stirred solution of 13 (192 mg, 873 µmol) in CH2Cl2 (2.7 mL) was added m-chloroperbenzoic acid (471 mg, 2.10 mmol) in portions over 15 min at 0 °C. Stirring was continued at rt for 20 min and the reaction mixture was quenched with aqueous Na2S2O3 and saturated aqueous NaHCO3, then extracted with CH2Cl2. The combined extracts were washed with brine, dried over Na2SO4 and concentrated under reduced pressure to give nitrone 14. To a stirred solution of 14 in MeOH (3.5 mL) was added hydroxylamine hydrochloride (303 mg, 4.35 mmol). The mixture was heated at 60 °C for 2.5 h. Then the reaction mixture was diluted with CH2Cl2 (6.0 mL) and filtered. The filtrate was washed with saturated aqueous NaHCO3 and brine. The organic layer was dried over Na2SO4 and concentrated under reduced pressure. The residue was purified by flash column chromatography (SiO2, CH2Cl2:MeOH = 50:1), followed by preparative TLC (SiO2, CH2Cl2:MeOH = 50:1) to afford compound 8h (83.8 mg, 49% for 2 steps): colorless powder; IR (neat) 3140, 2897, 1448, 1402, 1290, 1063, 1026, 1018, 910, 737, 681 cm-1; 1H NMR (500 MHz, CDCl3) δ 7.75-7.67 (4H, m), 7.40 (2H, dd, J = 7.5, 7.5 Hz), 7.34-7.28 (2H, m), 6.08 (1H, brs), 5.10 (1H, s) (one proton signal was missing owing to exchanging to deuterium); 13C NMR (100 MHz, CDCl3) δ 142.9, 141.1, 128.7, 127.4, 125.6, 120.0, 67.3; LRMS (EI) m/z 197 (M+); HRMS (EI) m/z Calcd for C13H11NO (M+) 197.0841, Found 197.0830.
2-(9H-Fluoren-9-ylamino)acetonitrile (13): yellow powder; IR (neat) 3329, 3065, 2249, 1448, 1296, 1200, 743, 667 cm-1; 1H NMR (500 MHz, CDCl3) δ 7.70 (2H, d, J = 7.0 Hz), 7.58 (2H, d, J = 7.0 Hz), 7.41 (2H, dd, J = 7.5, 7.0 Hz), 7.36 (2H, ddd, J = 7.5, 7.0, 1.2 Hz), 5.02 (1H, s), 3.22 (2H, s), 2.27 (1H, brs); 13C NMR (125 MHz, CDCl3) δ 143.0, 141.0, 128.9, 127.6, 125.0, 120.2, 118.2, 62.5, 31.9; LRMS (EI) m/z 220 (M+); HRMS (EI) m/z Calcd for C15H12N2 (M+) 220.1000, Found 220.1000.
2-(9H-Fluoren-9-ylimino)acetonitrile N-oxide (14): Trituration with EtOAc, n-hexane and CHCl3 afforded pure nitrone. colorless powder; IR (KBr) 3096, 2932, 2220, 1541, 1448, 1429, 1275, 1182, 1157, 966, 750, 725, 694 cm-1; 1H NMR (500 MHz, CDCl3) δ 7.75 (2H, d, J = 7.5 Hz), 7.71 (2H, dd, J = 8.0, 1.0 Hz), 7.54 (2H, dd, J = 7.5, 7.5 Hz), 7.41 (2H, ddd, J = 8.0, 7.5, 1.0 Hz), 6.40 (1H, s), 5.93 (1H, s); 13C NMR (125 MHz, CDCl3) δ 141.2, 137.8, 131.0, 128.8, 125.9, 120.8, 112.1, 104.2, 80.7; LRMS (FAB) m/z 235 ([M+H]+); HRMS (FAB) m/z Calcd for C15H11N2O ([M+H]+) 235.0871, Found 235.0874.
Data of hydroxylamines
N-(1-Indanyl)hydroxylamine (8a): colorless powder; IR (KBr) 3155, 2941, 2882, 760 cm-1; 1H NMR (400 MHz, CDCl3) δ 7.38 (1H, d, J = 7.6 Hz), 7.24-7.14 (3H, m), 4.50 (1H, dd, J = 7.2, 4.0 Hz), 3.06-2.99 (1H, m), 2.87-2.80 (1H, m), 2.32-2.23 (1H, m), 2.10-2.01 (1H, m) (two proton signals were missing owing to exchanging to deuterium); 13C NMR (100 MHz, CDCl3) δ 144.7, 141.6, 128.2, 126.3, 125.1, 124.9, 67.1, 30.4, 29.7; LRMS (EI) m/z 149 (M+); HRMS (EI) m/z Calcd for C9H11NO (M+) 149.0841, Found 149.0836.
1,2,3,4-Tetrahydro-N-hydroxyl-1-naphthaleneamine (8b): colorless powder; IR (KBr) 3155, 2941, 2882, 760 cm-1; 1H NMR (400 MHz, CDCl3) δ 7.32 (1H, dd, J = 6.4, 2.0 Hz), 7.25-7.09 (3H, m), 4.09 (1H, dd, J = 4.0, 4.0 Hz), 2.84-2.68 (2H, m), 2.25-2.20 (1H, m), 1.95-1.89 (1H, m), 1.85-1.75 (2H, m); 13C NMR (100 MHz, CDCl3) δ 138.5, 134.3, 129.6, 129.2, 127.4, 125.8, 59.3, 29.4, 25.8, 18.3; LRMS (EI) m/z 163 (M+); HRMS (EI) m/z Calcd for C10H13NO (M+) 163.0997, found 163.1015.
N-(6,7,8,9-Tetrahydro-5H-benzocyclohepten-5-yl)hydroxylamine (8c): colorless powder; IR (KBr) 3269, 2914, 1450, 1442, 1364, 1040, 905, 756 cm-1; 1H NMR (400 MHz, CDCl3) δ 7.25-7.06 (4H, m), 5.79 (2H, br s), 4.22 (1H, d, J = 8.4 Hz), 2.93 (1H, dd, J = 13.6, 10.0 Hz), 2.81-2.72 (1H, m), 2.02-1.85 (2H, m), 1.83-1.65 (3H, m), 1.63-1.50 (1H, m); 13C NMR (100 MHz, CDCl3) δ 142.2, 141.1, 129.8, 127.0, 126.3, 126.1, 65.8, 35.6, 31.1, 27.7, 27.7; LRMS (EI) m/z 160 ([M–OH]+); HRMS m/z Calcd for C11H14N([M–OH]+) 160.1126, Found 160.1101.
N-(Thiochroman-4-yl)hydroxylamine (8e): colorless powder; IR (KBr) 3254, 3167, 2885, 1587, 1562, 1475, 1441, 1408, 1034, 945, 887, 750 cm-1; 1H NMR (500 MHz, CDCl3) δ 7.25-7.00 (4H, m), 5.43 (1H, br s), 4.14 (1H, dd, J = 3.5, 3.5 Hz), 3.33 (1H, ddd, J = 13, 12, 3.5 Hz), 2.81 (1H, dd, J = 13, 4.0, 3.5 Hz), 2.60 (1H, dddd, J = 14, 4.0, 3.5, 3.5 Hz), 1.95 (1H, dddd, J = 14, 12, 3.5, 3.5 Hz) (one proton signal was missing owing to exchanging to deuterium); 13C NMR (100 MHz, CDCl3) δ 134.5, 131.4, 130.4, 128.3, 126.8, 124.0, 58.4, 24.4, 21.5; LRMS (EI) m/z 181 (M+); HRMS (EI) m/z Calcd for C9H11NOS (M+) 181.0561, Found 181.0562.
N-(4,5,6,7-Tetrahydrobenzo[b]thiophen-4-yl)hydroxylamine (8f): colorless powder; IR (KBr) 3155, 2941, 2882, 760 cm-1; 1H NMR (400 MHz, CDCl3) δ 7.08 (1H, d, J = 5.6 Hz), 7.00 (1H, d, J = 5.6 Hz), 4.12 (1H, dd, J = 4.8, 4.8 Hz), 2.83 (1H, ddd, J = 16.4, 5.2, 5.2 Hz), 2.77-2.69 (1H, m), 2.08-1.80 (4H, m) (two proton signals were missing owing to exchanging to deuterium); 13C NMR (100 MHz, CDCl3) δ 139.8, 133.7, 127.0, 122.3, 57.0, 26.1, 25.1, 19.8; LRMS (EI) m/z 169 (M+); HRMS (EI) m/z Calcd for C8H11NOS (M+) 169.0561, Found 169.0567.
N-(1-Phenylethyl)hydroxylamine (8i): colorless powder; IR (neat) 3252, 1499, 1452, 988, 760, 698 cm-1; 1H NMR (500 MHz, CDCl3) δ 7.37-7.26 (5H, m), 4.11 (1H, q, J = 6.8 Hz), 1.38 (3H, d, J = 6.8 Hz) (two proton signals were missing owing to exchanging to deuterium); 13C NMR (125 MHz, CDCl3) δ 142.3, 128.5, 127.6, 127.1, 61.8, 19.4; LRMS (EI) m/z 137 (M+); HRMS (EI) m/z Calcd for C8H11NO (M+) 137.0841, Found 137.0858.
N-(1-Phenylpropyl)hydroxylamine (8j): colorless powder; IR (KBr) 3265, 3144, 2864, 1495, 1452, 1346, 1026, 758, 696 cm-1; 1H NMR (400 MHz, CDCl3) δ 7.36-7.24 (5H, m), 3.82 (1H, dd, J = 8.8, 5.6 Hz), 1.93-1.81 (1H, m), 1.68-1.55 (1H, m), 0.81 (3H, t, J = 7.6 Hz) (two proton signals were missing owing to exchanging to deuterium); 13C NMR (100 MHz, CDCl3) δ 141.0, 128.4, 127.8, 127.6, 68.7, 26.2, 10.5; LRMS (EI) m/z 151 (M+); HRMS (EI) m/z Calcd for C9H13NO (M+) 151.0997, Found 151.1023.
Diphenylmethylhydroxylamine (8k): colorless powder; IR (KBr) 3252, 3084, 3028, 2880, 1493, 1450, 1429, 1085, 1034, 1022, 894, 752, 698, 636 cm-1; 1H NMR (400 MHz, CDCl3) δ 7.52-7.20 (10H, m), 5.17 (1H, br s) (two proton signals were missing owing to exchanging to deuterium); 13C NMR (100 MHz, CDCl3) δ 140.5, 128.5, 127.7, 127.5, 70.6; LRMS (EI) m/z 199 (M+); HRMS (EI) m/z Calcd for C13H13NO (M+) 199.0997, Found 199.0992.
General procedure for rearrangement reaction of hydroxylamines with DIBALH
5,6,11,12-Tetrahydrodibenz[b,f]azocine (9g): To a stirred solution of hydroxylamine 8g (68.9 mg, 0.306 mmol) in CH2Cl2 (3.1 mL), DIBALH (1.79 mL, 1.83 mmol, 1.02 M in n-hexane) was added dropwise at 0 °C under an argon atmosphere. Stirring was continued for 3.5 h at 0 °C. NaF powder (385 mg, 9.18 mmol) and water (100 µL) were added at 0 °C, and the resulting mixture was stirred for 30 min at the same temperature. Then the reaction mixture was filtered through a Celite pad. The filter cake was washed with CH2Cl2 and Et2O, and the combined organic solutions were evaporated under reduced pressure. The residue was purified by preparative TLC (SiO2, n-hexane:EtOAc = 9:1) yielded 9g (44.4 mg, 69%):2b colorless crystals; mp 128-130 °C; IR (KBr) 3364, 2924, 1603, 1477 cm-1; 1H NMR (400 MHz, CDCl3) δ 7.10-7.05 (3H, m), 7.02 (1H, dd, J = 8.0, 1.6 Hz), 6.97 (1H, dd, J = 7.6, 1.6 Hz), 6.89 (1H, ddd, J = 8.0, 7.6, 1.6 Hz), 6.68 (1H, ddd, J = 7.6, 7.6, 1.6 Hz), 6.49 (1H, dd, J = 8.0, 1.6 Hz), 4.42 (2H, s), 3.93 (1H, br s), 3.29 (2H, t, J = 7.2 Hz), 3.17 (2H, t, J = 7.2 Hz); 13C NMR (100 MHz, CDCl3) δ 147.3, 140.6, 137.3, 131.1, 130.3, 129.5, 129.3, 127.3, 126.8, 126.5, 120.0, 119.0, 51.8, 35.5, 32.5; LRMS (EI) m/z 209 (M+); HRMS (EI) m/z Calcd for C15H15N (M+) 209.1204, Found 209.1179.
Similarly, the rearrangement reactions of hydroxylamines 8a-f or 8h-k5-12 with DIBALH were carried out to afford compounds 6, 9a, 9c-e, 9h-k, or 10f,15 which were identified with those of the literatures reported previously.
ACKNOWLEDGEMENTS
This work was supported by the MEXT, the KAKENHI, a Grant-in-Aid for Scientific Research (B) (20390003), Tohoku University G-COE program ‘IREMC,’ and the JSPS predoctoral fellowship to Y.I.
References
1. (a) H. Cho, K. Murakami, H. Nakanishi, H. Isoshima, K. Hayakawa, and I. Uchida, Heterocycles, 1998, 48, 919; CrossRef (b) H. Cho, K. Murakami, A. Fujisawa, M. Niwa, H. Nakanishi, and I. Uchida, Heterocycles, 1998, 48, 1555; CrossRef (c) H. Cho, K. Murakami, H. Nakanishi, A. Fujisawa, H. Isoshima, M. Niwa, K. Hayakawa, Y. Hase, I. Uchida, H. Watanabe, K. Wakitani, and K. Aisaka, J. Med. Chem., 2004, 47, 101. CrossRef
2. (a) H. Cho, Y. Iwama, K. Sugimoto, E. Kwon, and H. Tokuyama, Heterocycles, 2009, 78, 1183; CrossRef (b) H. Cho, Y. Iwama, K. Sugimoto, S. Mori, and H. Tokuyama, J. Org. Chem., 2010, 75, 627. CrossRef
3. (a) R. V. Hoffman and A. Kumar, J. Org. Chem., 1985, 50, 1859; CrossRef (b) R. V. Hoffman and G. A. Buntain, J. Org. Chem., 1988, 53, 3316; CrossRef (c) J. Fujiwara, H. Sano, K. Maruoka, and H. Yamamoto, Tetrahedron Lett., 1984, 25, 2367; CrossRef (d) S. E. Booth, P. R. Jenkins, and C. J. Swain, J. Chem. Soc., Chem. Commun., 1993, 147; CrossRef (e) T. Kiguchi, K. Tajiri, I. Ninomiya, and T. Naito, Tetrahedron, 2000, 56, 5819; CrossRef (f) O. Miyata, T. Koizumi, H. Asai, R. Iba, and T. Naito, Tetrahedron, 2004, 60, 3893; CrossRef (g) O. Miyata, T. Ishikawa, M. Ueda, and T. Naito, Synlett, 2006, 2219; CrossRef (h) M. Ueda, S. Kawai, M. Hayashi, T. Naito, and O. Miyata, J. Org. Chem., 2010, 75, 914. CrossRef
4. Reduction of oxime with NaBH3CN; (a) R. F. Borch, M. D. Bernstein, and H. D. Durst, J. Am. Chem. Soc., 1971, 93, 2897; CrossRef (b) G. W. Gribble, R. W. Leiby, and M. N. Sheeha, Synthesis, 1977, 856. CrossRef
5. Reduction of oxime derivatives with borane complexes; (a) H. Feuer and B. F. Vincent, Jr., J. Am. Chem. Soc., 1962, 84, 3771; CrossRef (b) H. Feuer, B. F. Vincent, Jr., and R. S. Bartlett, J. Org. Chem., 1965, 30, 2877; CrossRef (c) Y. Kikugawa and M. Kawase, Chem. Lett., 1977, 6, 1279; CrossRef (d) M. Kawase and Y. Kikugawa, J. Chem. Soc., Perkin Trans. 1, 1979, 643. CrossRef
6. Reduction of oxime with H2/PtO2; F. Benington, R. D. Morin, and L. C. Clark, Jr., J. Med. Chem. 1965, 8, 100. CrossRef
7. Oxidation of amines; (a) A. H. Beckett, R. T. Coutts, and F. A. Ogunbona, Tetrahedron, 1973, 29, 4189; CrossRef (b) A. H. Beckett, K. Haya, G. R. Jones, and P. H. Morgan, Tetrahedron, 1975, 31, 1531; CrossRef (c) H. Tokuyama, T. Kuboyama, A. Amano, T. Yamashita, and T. Fukuyama, Synthesis, 2000, 1299.. CrossRef
8. Substitution reaction of alcohol with hydroxylamine hydrochloride; (a) T. R. Lamanec, D. R. Bender, A. M. DeMarco, S. Karady, R. A. Reamer, and L. M. Weinstock, J. Org. Chem., 1988, 53, 1768; CrossRef (b) Y. Hoshino and H. Yamamoto, J. Am. Chem. Soc., 2000, 122, 10452. Mitsunobu reaction of alcohol with hydroxylamine derivatives, followed by deprotection; CrossRef (c) O. Mitsunobu, Synthesis, 1981, 1; CrossRef (d) D. W. Knight and M. P. Leese, Tetrahedron Lett., 2001, 42, 2593. CrossRef
9. Reduction of nitro compounds with Zn/NH4Cl; E. G. Janzen and R. C. Zawalski, J. Org. Chem., 1978, 43, 1900. CrossRef
10. Reduction of nitro compounds with LAH; R. T. Gilsdorf and F. F. Nord, J. Am. Chem. Soc., 1952, 74, 1837. CrossRef
11. Reduction of α,β-unsaturated nitro compounds with BH3·THF/cat. NaBH3CN; M. S. Mourad, R. S. Varma, and G. W. Kabalka, J. Org. Chem., 1985, 50, 133. CrossRef
12. Reduction of nitronate with borane complexes; H. Feuer, R. S. Bartlett, B. F. Vincent, Jr., and R. S. Anderson, J. Org. Chem., 1965, 30, 2880. CrossRef
13. Z.-Y. Chang and R. M. Coates, J. Org. Chem., 1990, 55, 3464. CrossRef
14. (a) R. Sivappa, N. M. Hernandez, Y. He, and C. J. Lovely, Org. Lett., 2007, 9, 3861; CrossRef (b) W. Adam, A. K. Beck, A. Pichota, C. R. Saha-Moller, D. Seebach, N. Vogl, and R. Zhang, Tetrahedron: Asymmetry, 2003, 14, 1355. CrossRef
15. Compound 9a: T. Kubo, C. Katoh, K. Yamada, K. Okano, H. Tokuyama, and T. Fukuyama, Tetrahedron, 2008, 64, 11230; CrossRef Compound 9b and 9d: M. Ortiz-Marciales, L. D. Rivera, M. D. Jesús, S. Espinosa, J. A. Benjamin, O. E. Casanova, I. G. Figueroa, S. Rodriguez, and W. Correa, J. Org. Chem., 2005, 70, 10132; CrossRef Compound 9c: H. Ahlbrecht, E. O. Düber, J. Epsztajn, and R. M. K. Marcinkowski, Tetrahedron, 1984, 40, 1157; CrossRef Compound 9e: C. Mukheerjee and E. Biehl, Heterocycles, 2004, 63, 2309; CrossRef Compound 9f and 10f: see, reference 1a. Compound 9h: J. Barluenga, F. J. Fañanas, R. Sanz, and Y. Fernández, Chem. Eur. J., 2002, 8, 2034; CrossRef Compounds 9i and 9j: E. Byun, B. Hong, K. A. De Castro, M. Lim, and H. Rhee, J. Org. Chem., 2007, 72, 9815; CrossRef Compound 9k: D. Hollmann, S. Bahn, A. Tillack, and M. Beller, Angew. Chem. Int. Ed., 2007, 46, 8291. CrossRef