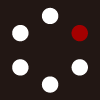
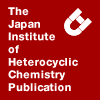
HETEROCYCLES
An International Journal for Reviews and Communications in Heterocyclic ChemistryWeb Edition ISSN: 1881-0942
Published online by The Japan Institute of Heterocyclic Chemistry
e-Journal
Full Text HTML
Received, 25th June, 2010, Accepted, 19th August, 2010, Published online, 20th August, 2010.
DOI: 10.3987/COM-10-S(E)70
■ Unexpected Formation of Dihydrobenzosilole Derivative via the Intramolecular Cyclization in the Reaction of Overcrowded Dichloromethylsilane with Aryllithium
Takahiro Sasamori,* Eiko Mieda, and Norihiro Tokitoh*
Institute for Chemical Research, Kyoto University, Uji, Kyoto 611-0011, Japan
Abstract
The reaction of overcrowded aryldichloromethylsilane 1 bearing a 2,6-bis[bis(trimethylsilyl)methyl]-4-[tris(trimethylsilyl)methyl]phenyl (Bbt) group with an excess amount of XylLi or MesLi (Xyl = 2,6-xylyl, Mes = mesityl) unexpectedly afforded the corresponding dihydrobenzosilole derivative 2 together with silyl-migrated olefin 3. The generation of 2 in this reaction is most likely interpreted in terms of intermediacy of 1,2-dihydro-2-chlorosilene 4.There has been much interest in low-coordinated species of heavier group 14 elements such as silylenes (R2Si:), silenes (R2Si=CR2), and disilenes (R2Si=SiR2).1 Generally, such species are too reactive to be synthesized and isolated as stable, monomeric compounds, but they can be isolated as stable species when they are well kinetically stabilized with using bulky substituents. Since these species are highly active toward addition reaction even in the case that they are well protected by the bulky substituents, they are good building blocks of heterocyclic compounds containing heavier group 14 elements. From this point of view, low-coordinated organosilicon compounds bearing a reactive site such as an Si–H bond or a halogen atom should be a potentially good precursor of heterocyclic compounds bearing further reactive site. Thus, we have focused our interest on silenes (Si=C) bearing a reactive site such as an Si–H, Si–Cl, or C–Cl bond. From this point of view, we have reported the synthesis of 1-hydrosilene derivative 5, which underwent slow dimerization giving the corresponding [6+6] dimer 6.2 In this paper, we present the attempted synthesis of 1,2-dihydro-2-chlorosilene derivative 4 bearing 2,6-bis[bis(trimethylsilyl)methyl]-4-[tris(trimethylsilyl)methyl]phenyl (Bbt) group as a steric protection group by the expected intramolecular migration of a hydrogen atom of chlorodihydrosilylcarbene 7. As a result, the reactions of Bbt-substituted dichloromethyldihydrosilane 1 with ArLi (Ar = Xyl, Mes; Xyl = 2,6-xylyl, Mes = mesityl) resulted in the unexpected formation of cyclic products, dihydrobenzosiloles 2.
When BbtSiH3 (657 mg, 1.00 mmol)3 was treated with Cl3COONa (1.85 g, 10 mmol) in toluene in the presence of 18-crown-6 (27.4 mg, 0.100 mmol) and heated under the reflux conditions, dichloromethyldihydrosilane 1 was obtained as colorless crystals in 87% yield (641 mg, 0.868 mmol).4 The structure of 1 was definitively characterized by the spectroscopic and X-ray crystallographic analyses as shown in Figure 1(a).5 It was shown that it has a space around the Si–C moiety enough to react with external reagents, even though the Bbt group is very bulky and may suppress the self dimerization of the resulting molecules. Compound 1 was treated with an excess amount (2.5 eq.) of XylLi as a base in THF at –80 ºC in the expectation of generating chlorosilylcarbene 7, which further undergoes intramolecular 1,2-hydrogen shift giving 2-chloro-1,2-dihydrosilene 4.6,7 However, instead of expected silene 4, dihydrobenzosilole 2a (20%) and olefin 3a (20%) were isolated as stable crystalline compounds.8 In the case of using MesLi instead of XylLi under the same conditions, similar products of 2b and 3b were obtained in 32 and 16% yields, respectively (estimated by 1H NMR spectrum).9 The obtained products, 2a,b and 3a,b, were characterized by the spectroscopic analysis, and the molecular structures of 2a and 3a were revealed by the X-ray crystallographic analysis (Figure 1).5 Unexpected product 2a is a unique, overcrowded dihydrobenzosilole derivative bearing trimethylsilyl groups.
The formation of compounds 2a,b and 3a,b is interesting and important from the viewpoints of the reactivity of extremely hindered chlorosilylcarbene 7 and a unique synthetic method for a dihydrobenzosilole derivative, although the detailed reaction mechanism leading to the formation of 2 and 3 is unclear at present. The plausible reaction mechanism for the formation of 2 and 3 is shown in Scheme 4. At the first stage of the reaction of 1 with ArLi (Ar = Mes or Xyl), deprotonation reaction would possibly occur at the CH moiety of the CHCl2 unit of 1 to afford chlorosilylcarbene 7.6,7 Attempted intermolecular trapping reaction of 7 using an excess amount of 2,3-dimethyl-1,3-butadiene was unsuccessful, indicating the intramolecular rearrangement reaction of 7 would proceed faster than the intermolecular reaction. Simply thinking, two types of intramolecular rearrangement may be possible for carbene 7, i. e., the 1,2-hydrogen shift to afford expected silene 4 and the C–H insertion at the benzyl position of the Bbt group giving 8.6,7 Since the obtained products, 2 and 3, were found to have an additional aryl group (Mes or Xyl) on the ring silicon atom, excess ArLi would react with the reactive intermediate 4 or 8. In the path through silene 4, ArLi would attack the silicon atom of the Si=C moiety giving carbenoid 9 due to the following reason.7 The LUMO of silene 4 exhibits large contribution of the p-orbital of the Si atom on the basis of the results of theoretical calculations on model compound 4’ (Figure 2).10 In addition, the resulting anionic species of 9 would be stabilized by the α-effect of a silicon atom. Carbenoid 9 thus generated would readily afford silylcarbene 10 along with the elimination of LiCl, and then silylcarbene 10 would undergo intramolecular cyclization via the C–H insertion at the benzyl position of the Bbt group to give compound 2. Thus, the formation of compound 2 is interpreted in terms of the intermediacy of silene 4. Although the formation of compound 3 cannot be reasonably explained by the reaction mechanism via 4, detailed theoretical calculations on the model molecules10 having 2,6-[(CH(SiMe3)2]2-C6H3 group instead of the Bbt group suggested another reaction mechanism for the formation of both 2 and 3. Since the LUMO of dihydrobenzosilole 8, which consists of a π orbital containing an Si moiety with Si–H σ* orbital, would lie in relatively low energy (that of model compound 8’ is computed as –0.59 eV), ArLi can attack 8 at the Si atom to give the corresponding silicate (Figure 2). In the theoretical calculations, silicate 11’– (model compound of 11–) was optimized as a local minimum, indicating that silicate 11– can exist as a meta-stable species (Figure 3). Two pseudo-rotational isomers of silicate 11’ – were found as the optimized structures, 11’A– (trigonal bipyramidal structure; H, C(Cl): axial, aryl groups and H: equatrial) and 11’B– (pseudo pyramidal), which would be transformed to each other via pseudo-rotation due to the small energy difference (0.57 kcal/mol). When a Li+ was located around each Cl atom (2.20 Å from the Cl atom) of 11’A– and 11’B–, their structural optimization resulted in the structures of products 2’ and 3’, respectively, along with the elimination of LiCl without energy-barrier (Figure 4).11 That is, Li+[11–] generated by the reaction of 8 with ArLi would undergo elimination of LiCl followed by the Si–H insertion and 1,2-migration of the SiMe3 group to give 2 and 3, respectively. Although the reaction mechanism for the formation of 2 and 3 is unclear at present, these theoretical investigations indicated that silene 4 and/or dihydrobenzosilole 8 generated from chlorosilylcarbene 7 would be a key intermediate in this reaction. Especially, it is of great interest that the possible reaction pathway via the intermediacy of silicate species of Li+[11–] giving 2 and 3 was suggested by theoretical calculations.
In the expectation of obtaining 4 and/or 8, the reaction of 1 (50.3 mg, 0.068 mmol) with an equimolar amount of MesLi was examined. As a result, a complicated mixture containing a small amount of 1 was obtained, indicating the possible intermediates would be highly reactive under these conditions. The crude mixture was subjected to column chromatography (SiO2, n-hexane) to give starting material 1 (11.5 mg, 15.5 µmol, 22%) together with some unidentifiable products.12
In summary, a unique heterocyclic compound, dihydrobenzosilole 2, was unexpectedly obtained together with olefin 3 by the reaction of 1 with an excess amount of ArLi (Ar = Mes or Xyl). Although we have examined the reaction of 1 with an equimolar amount of MesLi in the expectation of obtaining possible intermediates, it was unsuccessful and no useful information was obtained for the formation of 2 and 3. Theoretical calculations using model compounds indicated that the possible key intermediates would be silene 4 and/or silicate 11–. This unique reactivity of 1 is of great importance in view of the construction of novel Si-containing heterocyclic compounds.
ACKNOWLEDGEMENTS
This work was partially supported by Grants-in-Aid for Creative Scientific Research (No. 17GS0207), Science Research on Priority Areas (No. 19027024, “Synergy of Elements”), and the Global COE Program B09 (International Center for Integrated Research and Advanced Education in Materials Science) from Ministry of Education, Culture, Sports, Science and Technology, Japan. E. M. is grateful for JSPS fellowship for young scientists.
References
1. For reviews, see: A. G. Brook and M. A. Brook, Adv. Organomet. Chem., 1996, 39, 71; CrossRef J. Escudié, C. Couret, and H. Ranaivonjatovo, Coordin Chem Rev., 1998, 180, 565; CrossRef T. L. Morkin and W. J. Leigh, Acc. Chem. Res., 2001, 34, 129; CrossRef L. E. Gusel'nikov, Coord. Chem. Rev., 2003, 244, 149; CrossRef H. Ottosson and P. G. Steel, Chem.-Eur. J., 2006, 12, 1576; CrossRef M. Kira and T. Iwamoto, Adv. Organomet. Chem., 2006, 54, 73; CrossRef P. Jutzi, Angew. Chem. Int. Ed., 2000, 39, 3797; CrossRef R. Okazaki and R. West, Adv. Organomet. Chem., 1996, 39, 231; CrossRef Y. Mizuhata, T. Sasamori, and N. Tokitoh, Chem. Rev., 2009, 109, 3479. CrossRef
2. S. Ozaki, T. Sasamori, and N. Tokitoh, Organometallics, 2008, 27, 2163. CrossRef
3. N. Tokitoh, K. Wakita, T. Matsumoto, T. Sasamori, R. Okazaki, N. Takagi, M. Kimura, and S. Nagase, J. Chin. Chem. Soc., 2008, 55, 487.
4. Experimental procedures and chemical data: To a toluene solution (3 ml) of BbtSiH3 (1.00 mmol, 655.6 mg) and 18-crown-6 ether (27.4 mg, 0.104 mmol) was added Cl3COONa (1.85 g, 10.0 mmol) and the mixture was refluxed for 6 h. After cooling at room temperature, benzene was added to the reaction mixture. The resulting suspension was filtered through Celite® and the solvent was removed. The residue was separated by wet column chromatography (n-hexane) to afford 1 (641 mg, 0.868 mmol, 87%). 1: mp 154.9 °C (decomp.), IR (neat), ν (Si-H) 2181 cm–1. 1H NMR (300 MHz, CDCl3) δ 6.76 (s, 2H), 5.49 (t, J = 3 Hz, 1H), 4.84 (d, J = 3 Hz, 2H), 2.07 (s, 2H), 0.25 (s, 27H), 0.07 (s, 36H). 13C NMR (75 MHz, CDCl3) δ 152.60 (s), 148.98 (s), 126.22 (d), 123.80 (s), 59.26 (d), 32.09 (d), 22.99 (s), 5.48 (q), 1.38 (q). 29Si NMR (59 MHz, CDCl3) δ 2.00, 0.85, –30.37. HRMS(FAB) m/z calcd for C31H70Si835Cl2 (M+): 736.3009, found: 736.3009. Anal. Calcd for C31H70Si8Cl2: C, 50.42; H, 9.55. Found: C, 50.17; H, 9.57.
5. X-Ray crystallographic data for 1 (C31H70Cl2Si8): M = 738.49, T = 103(2) K, monoclinic, P21/n (no.14), a = 9.579(3) Å, b = 36.546(11) Å, c = 12.922(4) Å, β = 102.943(3)º, V = 4409(2) Å3, Z = 4, Dcalc = 1.113 g cm-3, μ = 0.384 mm–1, λ = 0.71070 Å, 2θmax = 51.0, 35196 measured reflections, 8166 independent reflections (Rint = 0.0499), 399 refined parameters, GOF = 1.140, R1 = 0.0455 and wR2 = 0.0969 [I>2σ(I)], R1 = 0.0574 and wR2 = 0.1029 [for all data], largest diff. peak and hole 0.443 and -0.327 e.Å-3. X-Ray crystallographic data for 2a (C39H78Si8): M = 771.73, T = 103(2) K, monoclinic, P–1 (no.2), a = 12.6047(5) Å, b = 13.5218(6) Å, c = 16.5215(13) Å, α = 96.538(2)º, β = 102.503(3)º, γ = 117.019(3)º, V = 2375.0(2) Å3, Z = 2, Dcalc = 1.079 g cm-3, μ = 0.251 mm–1, λ = 0.71069 Å, 2θmax = 51.0, 20766 measured reflections, 8786 independent reflections (Rint = 0.0583), 451 refined parameters, GOF = 1.022, R1 = 0.0556 and wR2 = 0.1317 [I>2σ(I)], R1 = 0.0893 and wR2 = 0.1506 [for all data], largest diff. peak and hole 0.516 and -0.361 e.Å-3. X-Ray crystallographic data for 3a (C39H78Si8): M = 771.73, T = 103(2) K, monoclinic, P21/n (no.14), a = 12.3778(2) Å, b = 16.9921(3) Å, c = 22.7508(4) Å, β = 92.7957(9)º, V = 4779.36(14) Å3, Z = 4, Dcalc = 1.073 g cm-3, μ = 0.249 mm–1, λ = 0.71069 Å, 2θmax = 50.0, 40306 measured reflections, 8411 independent reflections (Rint = 0.0684), 474 refined parameters (36 restraints), GOF = 1.078, R1 = 0.0569 and wR2 = 0.1249 [I>2σ(I)], R1 = 0.0824 and wR2 = 0.1405 [for all data], largest diff. peak and hole 0.969 and -0.328 e.Å–3. Crystallographic data (excluding structure factors) for the structures of 1, 2a, and 3a reported in this paper have been deposited with the Cambridge Crystallographic Data Centre as supplementary publication nos. CCDC 780078-780080. Copies of the data can be obtained free of charge on application to CCDC, 12 Union Road, Cambridge CB21EZ, UK (fax: (+44)1223-336-033; E-mail: deposit@ccdc.cam.ac.uk.). The intensity data were collected on a Rigaku/MSC Mercury CCD diffractometer. The structure was solved by direct methods (SHELXS-97) and refined by full-matrix least-squares procedures on F2 for all reflections (SHELXL-97).
6. K. Schmohl, T. Gross, H. Reinke, and H. Oehme, Z. Anorg. Allg. Chem., 2000, 626, 1100; CrossRef K. Schmohl, H. Reinke, and H. Oehme, Z. Anorg. Allg. Chem., 2001, 627, 2619. CrossRef
7. M. Mickoleit, K. Schmohl, R. Kempe, and H. Oehme, Angew. Chem. Int. Ed. 2000, 39, 1610; CrossRef U. Bäumer, H. Reinke, and H. Oehme, J. Organomet. Chem., 2006, 691, 229. CrossRef
8. Experimental procedures and chemical data: To a THF solution (1 mL) of 1 (73.4 mg, 0.10 mmol) was added 2,6-dimethylphenyllithium (0.30M in THF, 0.845 mL, 0.25 mmol) at –80 °C. After stirring at the same temperature for 1 h, the reaction mixture was allowed to warm up to room temperature. The solvent was evaporated under reduced pressure and hexane was added to the residue. Insoluble inorganic salts were removed by filtration through Celite®. Purification by GPLC and PTLC afforded 2a (15.2 mg, 0.020 mmol, 20%), 3a (15.6 mg, 0.020 mmol, 20 %). 2a: mp 159.0 °C (decomp.) 1H NMR (300MHz, CDCl3) δ 7.16 (t, J = 6 Hz, 1H), 7.04 (d, J = 6 Hz, 1H), 6.88 (d, J = 6 Hz, 1H), 6.80 (s, 1H), 6.68 (s, 1H), 5.64 (dd, 1J = 6 Hz, 3J = 3 Hz, 1H, SiH), 2.66 (s, 3H), 2.13 (s, 3H), 1.53(dd, 2J = 15 Hz, 3J = 6 Hz, 1H), 1.42 (s, 1H), 1.30 (dd, 2J = 15 Hz, 3J = 3 Hz, 1H), 0.24 (s, 27H), 0.18 (s, 9H), 0.06 (s, 9H), 0.01 (s, 9H), –0.33 (s, 9H). 13C NMR (75MHz, CDCl3) δ 159.25 (s), 149.55 (s), 147.43 (s), 145.92 (s), 145.72 (s), 132.67 (s), 132.52 (s), 130.33 (d), 128.77 (d), 128.29 (d), 126.66 (d), 124.45 (d), 33.50 (d), 32.41 (s), 26.66 (q), 25.55 (q), 22.46 (s), 13.96 (t), 5.78 (q), 1.80 (q), 1.77 (q), 1.30 (q), 0.82(q). 29Si NMR (60 MHz, CDCl3) δ 8.15, 4.35, 2.66, 0.95, 0.72, –16.9. HRMS (FAB) m/z: Calcd for C39H78Si8 ([M+]) 770.4258, found 770.4265. 3a: mp 217.6 °C (decomp.) 1H NMR (300MHz, CDCl3) δ 7.17 (t, J = 7.5 Hz, 1H), 6.98 (d, J = 7.5 Hz, 2H), 6.80 (d, J = 2.1 Hz, 1H), 6.68 (d, J = 2.1 Hz, 1H), 6.26 (s, 1H), 4.97 (d, J = 3 Hz, 1H, SiH), 4.90 (d, J = 3 Hz, 1H, SiH), 2.46 (s, 6H), 1.97 (s, 1H), 0.23 (s, 27H), 0.05 (s, 9H), –0.07 (s, 9H), –0.11 (s, 9H), –0.12 (s, 9H). 13C NMR (75MHz, CDCl3) δ 167.47 (s), 154.10 (s), 151.45 (s), 144.85 (s), 144.49 (s), 144.27 (d), 133.20 (s), 129.67 (d), 127.89 (d), 127.65 (d), 126.45 (d), 122.14 (s), 29.02 (d), 25.24 (q), 4.95 (q), 1.53 (q), 1.31 (q), 0.00 (q), –0.49 (q). 29Si NMR (60 MHz, CDCl3) δ 1.96, 1.86, 0.80, –5.32, –9.04, –56.7. HRMS (APPI/TOF) m/z: Calcd for C36H69Si7 ([M+–SiMe3]) 697.3779, found 695.3766.
9. Experimental procedures and chemical data: To a THF solution (1 mL) of 1 (74.0 mg, 0.10 mmol) was added MesLi (0.125M in THF, 2.0 mL, 0.25 mmol) at -80 °C. After stirring at the same temperature for 1 h, the reaction mixture was allowed to warm up to room temperature. The solvent was evaporated under reduced pressure and hexane was added to the residue. Insoluble inorganic salts were removed by filtration through Celite®. Purification by GPLC and PTLC afforded mixture of 2b and 3b. (37.7 mg, 32 % of 2b and 16 % of 3b as judged by 1H NMR spectrum). 2b: mp 228.0 °C (decomp.) 1H NMR (300MHz, CDCl3) δ 6.87 (s, 1H), 6.79 (d, J = 1.6 Hz, 1H), 6.72 (s, 1H), 6.67 (d, J = 1.6 Hz, 1H), 5.61 (dd, 1J = 6 Hz, 3J = 3.5 Hz, 1H, SiH), 2.62 (s, 3H), 2.23 (s, 3H), 2.09 (s, 3H), 1.51 (dd, 2J = 15 Hz, 3J = 6.9 Hz, 1H), 1.44 (s, 1H), 1.27 (dd, 2J = 15 Hz, 3J = 3.5 Hz, 1H), 0.23 (s, 27H), 0.17 (s, 9H), 0.05 (s, 9H), 0.01 (s, 9H), –0.33 (s, 9H). HRMS(FAB) m/z: Calcd for C40H80Si8 ([M+]) 784.4414, found 784.4415. 29Si NMR (60 MHz, CDCl3) δ 8.07, 4.29, 2.60, 0.95, 0.70, –16.7. 3b: mp 192.8 °C (decomp.) 1H NMR (300MHz, CDCl3) δ 6.87 (s, 1H), 6.79 (d, J = 1.6 Hz, 1H), 6.72 (s, 1H), 6.67 (d, J = 1.6 Hz, 1H), 4.95 (d, J = 2.4 Hz, 1H, SiH), 4.87 (d, J = 2.4 Hz, 1H, SiH), 2.42 (s, 6H), 2.24 (s, 3H), 1.99 (s, 1H), 0.23 (s, 27H), 0.05 (s, 9H), –0.08 (s, 9H), –0.12 (s, 9H), –0.13 (s, 9H). 29Si NMR (60 MHz, CDCl3) δ 1.91, 1.84, 0.78, –5.39, –9.10, −56.6. HRMS(FAB) m/z: Calcd for C40H80Si8 ([M+]) 784.4414, found 784.4427.
10. All of the theoretical calculations described in this paper were performed at the B3LYP/6-31G(d) level using Gaussian 03 program. Since the calculations using real molecules related to our work using extremely large substituents cost extremely long time, model compounds bearing 2,6-[(CH(SiMe3)2]2-C6H3 group instead of the Bbt group were used and Xyl group was chosen as Ar group for the calculations.
11. At present, the transition state was not found in the calculations of IRC and detailed structural optimization. Accordingly, it can be thought that these isomerization reactions would proceed without energy-barrier. However, the possibility of the existence of the transition state with very small energy-barrier cannot be completely excluded at this stage.
12. It was found that one of the unidentified products is silene-dimer 12 on the basis of the preliminary results of the X-ray crystallographic analysis of a single crystal obtained by the recrystallization of the mixture of unidentified products. Accordingly, another reaction pathway giving 2 and 3 via silene 13 may be taken into account in the reaction of 1 with ArLi. The data set of 12 was collected at beamline BL38B1 (Spring-8 Japan)