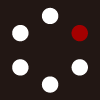
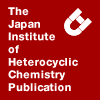
HETEROCYCLES
An International Journal for Reviews and Communications in Heterocyclic ChemistryWeb Edition ISSN: 1881-0942
Published online by The Japan Institute of Heterocyclic Chemistry
e-Journal
Full Text HTML
Received, 27th May, 2010, Accepted, 21st June, 2010, Published online, 22nd June, 2010.
DOI: 10.3987/COM-10-S(E)39
■ A Flexible Route to 4-Substituted β-Lactams
Béatrice Quiclet-Sire* and Samir Z. Zard*
CNRS UMR 7652, Laboratoire de Synthèse Organique Associé, Ecole Polytechnique, F-91128 Palaiseau, France
Abstract
A variety of 4-substituted β-lactams can be readily prepared by the radical addition-transfer of 4-S-xanthyl-azetidinones to unactivated olefins. For this radical chain process to operate efficiently it is necessary to place an acyl group on the nitrogen of the azetidinone ring.INTRODUCTION
The enormous importance of β-lactam antibiotics and the rich chemistry associated with the strained lactam ring, especially in its capacity to act as an activated, latent β-aminoacid, have continued to stimulate the interest of organic chemists.1,2 In addition to the development of methods for constructing the β-lactam ring itself, much effort has been expanded for the modification of existing structures. The introduction of a substituent at position 4 of β-lactams has often been realised by replacement of a potential leaving group X in structure 1 by various nucleophilic reagents to give derivatives 2 (Scheme 1).3 Thus, carbanions, organometallics, enol silyl ethers, ketene silyl acetals and allylsilanes can be used for the substitution reaction, the last three families of nucleophiles usually requiring the mediation of a Lewis acid. In stark contrast, the introduction of substituents by reactions proceeding by way of radical 3 and leading to structures 4, has very seldom been exploited, despite its obvious potential. One rare example reported a quarter of a century ago by Fliri and Mak and portrayed in Scheme 1 is the radical allylation of azetidinone 5 with allyltributyltin to provide compound 6 in good yield.4 This approach is however limited to very simple allylations and requires the use of organotin derivatives, which are not acceptable in a pharmaceutical context because of the acute toxicity of organotin derivatives and the extreme practical difficulty in completely removing tin residues from the products.
RESULTS AND DISCUSSION
Over the past years, we have demonstrated the unique ability of xanthates for creating new carbon-carbon bonds by intermolecular radical addition to unactivated alkenes.5 More generally, relatively slow and otherwise difficult radical transformations can often be accomplished because the xanthate transfer process imparts a comparatively long lifetime to the intermediate radicals, even under concentrated conditions. We exploited this property to construct the β-lactam ring itself, but it seemed interesting to expand the scope to the modification of existing β-lactam structures. However, while the addition of S-cyanomethyl xanthate 8 to N-allyl β-lactam 7 could indeed be readily accomplished, the addition of β-lactam xanthate 10 to simple unactivated alkenes 11 proceeded in very poor yield (Scheme 1).
The most likely reason underlying this disappointing failure may be traced to the relative stabilities of the various radical intermediates involved in the xanthate addition-transfer, which can be readily understood by a brief examination of the simplified mechanism displayed in Scheme 2. One of the key requirements of the process is that, in the absence of special polar factors, the initial radical, in this case 14, must be more stable than the adduct radical 15 in order for the equilibrium involving intermediate 16 to favour the forward pathway and thus sustain the desired chain reaction. In other words, the fragmentation of intermediate 16 has to preferentially lead to addition product 17 and starting radical 14, rather than back to adduct radical 15. The failure of the desired addition indicated that this is probably not the case, and that the stabilisation of radical 14 by the lone pair of the nitrogen is not sufficient, presumably for reasons related to the strained geometry of the azetidinone structure.
With the aim of enhancing the stability of radical 14 sufficiently to tilt the equilibrium along the forward direction, we contemplated starting with the N-acyl derivatives 13 (R’ = MeCO-), where we hoped the radical would acquire a certain degree of allylic character through canonical structures 18a-c of the imide, as pictured in the lower part of Scheme 2. A similar spin delocalization appears to operate in the case of phthalimidomethyl radicals, which we exploited to accomplish a very efficient and broadly applicable radical aminomethylation of alkenes.6 Because of the strain involved, the extra stabilisation provided by canonical structures 18b and 18c would be expected to be less effective than in the case of the much less strained phthalimido analogues, but even a small stabilisation could prove decisive in ensuring success.
We therefore prepared N-acetyl derivative 21a from known xanthate 10,7 itself readily obtained from commercial 4-acetoxyazetidinone 19 (Scheme 3). In the same manner, we prepared the neopentyl analogue 21b (neoPn = neopentyl). We have observed over the years that S-neopentyl xanthates tended to be more crystalline than their ethyl analogues, and this can be advantageous when there is a need to purify delicate structures.8 Neopentyl derivatives were also much less susceptible to undesired ionic transformations. When a concentrated solution of xanthate 21a and methyl 10-undecenoate 11a was heated at reflux in ethyl acetate in the presence of a small amount of lauroyl peroxide, we were gratified to find that a relatively smooth reaction took place to give the desired product 22a in 65% yield. To simplify the characterization of the product, the xanthate group was removed to give 23a in near quantitative yield by reduction with tris-(trimethylsilyl)silane and a small amount of AIBN in refluxing 1:1 toluene:cyclohexane (Scheme 3).9
The success of this preliminary reaction opens up numerous possibilities for rapidly constructing highly functional and indeed quite complex azetidinones. One such example, also pictured in Scheme 3, is the synthesis of di-β-lactam 23b by the addition of S-azetidinyl xanthate 21b to 3-allyl azetidinone 11b and reductive removal of the xanthate group from the intermediate adduct 22b. One of the main features of the present radical process is its tolerance for numerous functional groups because of the extreme mildness of the experimental conditions. This is illustrated by the addition reactions of 3-substituted xanthate 26, derived from commercially available 4-acetoxyazetidinone derivative 24 (Scheme 4). Thus, the addition reaction of 26 with methyl 10-undecenoate 11a proceeded as effectively as with the simpler xanthate 21a leading finally to compound 28 following the removal of the xanthate group. Only one isomer is obtained since the bulky protected hydroxyethyl substituent on C-3 of the azetidinone forces the new carbon-carbon bond to form from the opposite side of the ring. The addition of 26 to the dimethyl ester 11c of Feist’s acid is another example of the degree of complexity that can be swiftly attained.
We had previously reported the use of allyl and vinyl ethylsulfones as tin-free allylating and vinylating reagent for both aliphatic iodides and xanthates.10 An example of the latter transformation is shown in Scheme 5, whereby S-azetidinyl xanthate 26 is converted in high yield into nicely crystalline (mp: 85-86 °C) dichlorovinyl derivative 30 upon reaction with sulfone 29. Very recently, we discovered that allylic alcohols become highly versatile radical allylating agents when they are converted into their 6-allyloxy-2-fluoropyridinyl derivatives.11 This process has an enormous potential for synthesis in view of the central position played by alkenes in organic chemistry. An illustration in the present case is the formation of protected enal 32, as a 5:1 mixture of geometrical isomers by addition-fragmentation of xanthate 26 to derivative 31. It is worth noting that in this case, the lauroyl peroxide must be used in stoichiometric amounts since the departing fluoropyridinyloxy radical is unable to propagate the chain. Another process, which also requires the use of stoichiometric peroxide, is the intermolecular addition of xanthates to certain heteroaromatic derivatives, as illustrated by the formation in moderate yield of compound 34 by reaction of xanthate 26 with 3-cyanoindole 33. While this approach is somewhat limited in scope as far as the heteroaromatic component is concerned, it can nevertheless furnish in one step some very interesting structures.12
In all the preceding examples, the N-acetyl group serves to stabilize the radical and, even if this stabilization is presumably relatively small in absolute terms, it has proved essential for implementing the chain process, which does not operate in its absence. The drawback is that it is very difficult to remove this acetyl group without destroying the azetidinone ring, and this obliterates to a large extent the utility of this approach. In order to circumvent this rather serious problem, we examined the replacement of the acetyl by an oxalyl group. It was known by researchers in the β-lactam field that an N-oxalyl group could be removed under extremely mild conditions that did not affect the fragile azetidinone ring.13 We therefore prepared xanthate 35 from β-lactam xanthate 10 and, indeed, found that the addition and removal of the oxalyl group could be readily accomplished, as demonstrated by the synthesis of compounds 39a,b possessing a free N-H group on the β-lactam ring (Scheme 6).
The intermediate adduct 38a was not purified in this sequence but immediately converted into 39a by exposure to triethylamine in methanol. In fact, the purification of N-oxalyl azetidinones proved rather tricky and some decomposition was observed upon chromatographic separation. We attempted to overcome this hurdle by replacing the O-ethyl xanthate group in 35 with an O-neopentyl xanthate and the methyl oxalate with the much bulkier 9-fluorenyl ester.14 However, the resulting derivative 36 did not prove superior in relation to the synthetic effort need to prepare it, as shown by the formation of analogous product 39b in similar overall yield (59% vs 65%). The remainder of the study was therefore performed using azetidinyl xanthate 37, which was readily obtained and proved easy to purify by crystallisation.
Xanthate 37 was reacted with a selection of five olefins, 11d-h, to give the corresponding adducts 40-44 in useful overall yield. In two instances (41 and 44), the xanthate group was reductively removed. In the case of 41, the volatility of the olefin caused a significant lowering of the yield. The attachment of a β-lactam motif to a glucose residue as in 44 is noteworthy and further demonstrates the synthetic potential of this novel chemistry.
In summary, we have developed a flexible approach to highly substituted azetidinones. While the radical additions of the β-lactam xanthates are slightly less efficient than the phthalimido substituted xanthates we described previously, many of the compounds reported in this preliminary work would be exceedingly tedious, if not impossible, to make by more traditional routes. Indeed, the xanthate transfer technology represents a quite general solution to a longstanding problem in organic synthesis, namely the creation of a carbon-carbon bond in an intermolecular fashion starting with non-activated alkenes.
References
1. The Chemistry of β-Lactams, ed. by M. I. Page, Blackie Academic & Professional: New York, 1992; The Organic Chemistry of β-Lactams, ed. by G. I. Georg, VCH: New York, 1993; Chemistry and Biology of β-lactam Antibiotics, ed. by R. B. Morin and M. Gorman, Academic Press: New York, 1982, Vols 1-3; C. I. Samarendra, N. Maiti, R. G. Micetich, M. Daneshtalab, K. Atchison, O. A. Phillips, and C. Kunugita, J. Antibiot., 1994, 47, 1030.
2. B. Alcaide, P. Almendros, and C. Aragoncillo, Chem. Rev., 2007, 107, 4437; CrossRef I. Ojima, Adv. Asym. Synth., 1995, 1, 95.
3. For some recent examples, see: M. J. Bodner, R. M. Phelan, and C. A. Townsend, Org. Lett., 2009, 11, 3606; CrossRef H. Sakaguchi, H. Tokuyama, and T. Fukuyama, Org. Lett., 2008, 10, 1711; CrossRef F. Broccolo, G. Cainelli, G. Caltabiano, C. E. A. Cocuzza, C. G. Fortuna, P. Galletti, D. Giacomini, G. Musumarra, R. Musumeci, and A. Quintavalla, J. Med. Chem., 2006, 49, 2804; CrossRef M. F. Jacobsen, M. Turks, R. Hazell, and T. Skrydstrup, J. Org. Chem., 2002, 67, 2411; CrossRef P. Maragni, M. Mattioli, R. Pachera, A. Perboni, and B. Tamburini, Org. Process Res. Dev., 2002, 6, 597. CrossRef
4. H. Fliri and C. P. Mak, J. Org. Chem., 1985, 50, 3438; CrossRef Interestingly, the use of radical reactions to construct the azetidinone ring itself are more common. For a recent review and some leading examples, see: A. Brandi, S. Cicchi, and F. M. Cordero, Chem. Rev., 2008, 108, 3988; CrossRef L. Benati, G. Bencivenni, R. Leardini, M. Minozzi, D. Nanni, R. Scialpi, P. Spagnolo, and G. Zanardi, J. Org. Chem., 2006, 71, 3192; CrossRef G. A. DiLabio, E. M. Scanlan, and J. C. Walton, Org. Lett., 2005, 7, 155; CrossRef I. Ryu, H. Miyazato, H. Kuriyama, K. Matsu, M. Tojino, T. Fukuyama, S. Minakata, and M. Komatsu, J. Am. Chem. Soc., 2003, 125, 5632; CrossRef L. Boiteau, J. Boivin, B. Quiclet-Sire, and S. Z. Zard, Tetrahedron, 1998, 54, 2087, and references cited therein. CrossRef
5. For reviews of this work, see: S. Z. Zard, Angew. Chem., Int. Ed. Engl., 1997, 36, 672; CrossRef B. Quiclet-Sire and S. Z. Zard, Phosphorus, Sulfur, Silicon, Related Elem., 1999, 137; S. Z. Zard, in Radicals in Organic Synthesis, ed. by P. Renaud, M. P. Sibi, Wiley-VCH: Weinheim, 2001, Vol. 1, 90; CrossRef B. Quiclet-Sire and S. Z. Zard, Chem. Eur. J., 2006, 12, 6002; CrossRef B. Quiclet-Sire and S. Z. Zard, Top. Curr. Chem., 2006, 264, 201; CrossRef S. Z. Zard, Org. Biomol. Chem., 2007, 5, 205. CrossRef
6. B. Quiclet-Sire and S. Z. Zard, Org. Lett., 2008, 10, 3279; CrossRef F. Lebreux, B. Quiclet-Sire, and S. Z. Zard, Org. Lett., 2009, 11, 2844; CrossRef B. Quiclet-Sire, G. Revol, and S. Z. Zard, Org. Lett., 2009, 11, 3554; CrossRef B. Quiclet-Sire, G. Revol, and S. Z. Zard, Tetrahedron, in press.
7. K. Clauss, D. Grimm, and J. Prossel, Justus Liebigs Ann. Chem., 1974, 539.
8. J. Boivin, J. Camara, and S. Z. Zard, J. Am. Chem. Soc., 1992, 114, 7909; CrossRef B. Quiclet-Sire and S. Z. Zard, J. Am. Chem. Soc., 1996, 118, 9190; CrossRef M.-P. Denieul, B. Quiclet-Sire, S. Z. Zard, Chem. Commun., 1996, 2511. CrossRef
9. C. Chatgilialoglu, Chem. Rev., 1995, 95, 1229. CrossRef
10. F. Bertrand, F. Leguyader, L. Liguori, G. Ouvry, B. Quiclet-Sire, S. Seguin, and S. Z. Zard, C. R. Acad. Sci. Paris, 2001, II4, 547; B. Quiclet-Sire and S. Z. Zard, J. Am. Chem. Soc., 1996, 118, 1209; CrossRef F. Leguyader, B. Quiclet-Sire, S. Seguin, and S. Z. Zard, J. Am. Chem. Soc., 1997, 119, 7410; CrossRef B. Quiclet-Sire, S. Seguin, and S. Z. Zard, Angew. Chem., Int. Ed., 1998, 37, 2864; CrossRef F. Bertrand, B. Quiclet-Sire, and S. Z. Zard, Angew. Chem. Int. Ed., 1999, 38, 1943; CrossRef G. Ouvry, S. Z. Zard, Synlett, 2003, 1627; CrossRef N. Charrier and S. Z. Zard, Angew. Chem. Int. Ed., 2008, 47, 9443. CrossRef
11. N. Charrier, B. Quiclet-Sire, and S. Z. Zard, J. Am. Chem. Soc., 2008, 130, 8898. CrossRef
12. P. E. Reyes-Gutiérrez, R. O. Torres-Ochoa, R. Martinez, L. D. Miranda, Org. Biomol. Chem., 2009, 7, 1388; CrossRef M. A. Guerrero, L. D. Miranda, Tetrahedron Lett., 2006, 47, 2517; CrossRef Y. M. Osornio, R. Cruz-Almanza, V. Jimenez-Montano, L. D. Miranda, Chem. Commun., 2003, 2316; CrossRef F. Coppa, F. Fontana, 13. R. D. G. Cooper, F. L. José, J. Am. Chem. Soc., 1972, 94, 1021F. Minisci, G. Pianese, P. Tortoreto, L. Zhao, Tetrahedron Lett., 1992, 33, 687. CrossRef
13. R. D. G. Cooper and F. L. José, J. Am. Chem. Soc., 1972, 94, 1021; CrossRef I. Ernest, J. Gosteli, C. W. Greengrass, W. Holick, D. E. Jackman, H. R. Pfaendler, and R. B. Woodward, J. Am. Chem. Soc., 1978, 100, 8214; CrossRef I. Ernest, Helv. Chim. Acta, 1980, 63, 201; CrossRef M. D. Bachi and A. Gross, J. Chem. Soc., Perkin Trans. 1, 1983, 1157; CrossRef D. P. Sahu, P. Mashawa, M. S. Manhas, A. K. Bose, J. Org. Chem., 1983, 48, 1142; CrossRef M. Foglio, C. Battistini, F. Zarini, C. Scarafile, and G. Franceschi, Heterocycles, 1983, 20, 1491; CrossRef M. Alpegiani, A. Badeshi, E. Perrone, F. Zarini, and G. Franceschi, Heterocycles, 1985, 23, 2255; CrossRef H. R. Pfaendler and H. Hoppe, Heterocycles, 1985, 23, 265; CrossRef H. Ludescher, C. P. Mak, G. Schulz, and H. Fliri, Heterocycles, 1987, 26, 885; CrossRef M. Endo, Can. J. Chem., 1987, 65, 2140; CrossRef H. Ceric, M. Kovacevic, and M. Sindler-Kulyk, Tetrahedron, 2000, 56, 3985. CrossRef
14. S. Biondi, A. Pecunioso, F. Busi, S. A. Contini, D. Donati, M. Maffeis, D. A. Pizzi, L. Rossi, T. Rossi, and F. M. Sabbatini, Tetrahedron, 2000, 56, 5649 CrossRef