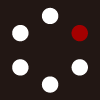
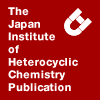
HETEROCYCLES
An International Journal for Reviews and Communications in Heterocyclic ChemistryWeb Edition ISSN: 1881-0942
Published online by The Japan Institute of Heterocyclic Chemistry
e-Journal
Full Text HTML
Received, 11th November, 2010, Accepted, 24th December, 2010, Published online, 20th January, 2011.
DOI: 10.3987/COM-10-S(E)127
■ Oxidative Coupling of Indoles with 3-Oxindoles
Mikkel Jessing and Phil S. Baran*
Department of Chemistry, The Scripps Research Institute, 10550 N. Torrey Pines Road,
BCC-169, La Jolla, CA 92037, U.S.A.
Abstract
A mild procedure for the union of 3-oxindoles with indoles is reported using oxidative coupling.In 2004, we reported the direct oxidative coupling of enolates with indoles1 and later expanded these findings to pyrroles,2 and the heterocoupling of different carbonyl enolate species.3 As a simple extension to this methodology, a mild and versatile oxidative coupling procedure for union of 3-oxindoles with indoles and pyrroles is presented in this short Note. The procedure is simple, the scope is broad for 3-oxindoles bearing a carboxy group at C-2, and the reaction can be conducted on a gram scale using CAN as the stoichiometric oxidant.
Reports have recently appeared in the literature regarding the construction of 3-oxindoles substituted with indoles at the 2-position.4,5 The chemistry used for the key C-C bond formation was that of Wasserman6 and Ke-Qing7 where a 2-hydroxy-3-oxindole is generated as the key intermediate. In this two-step procedure, a hemiaminal is in equilibrium with an iminium ion which is subsequently attacked by a nucleophile (indole7 or pyrrole6). Previously, one-step oxidative couplings with indoles that occur at C–2 have been reported using manganese triacetate.8 C-3 substitution was only observed when C-2 was already substituted.9 Other oxidative couplings for C-3 substitution require prefunctionalization.10 By analogy to the oxidative couplings that we previously had developed,1-3 a one step oxidative coupling of indoles to 3-oxindoles that could be conducted under mild conditions was pursued. For this oxidative coupling, 2-carboxymethyl-3-oxindole (2) was chosen, as this ester group could, in principle, be transformed to an aromatic group4 or a tetrahydrofuran ring5 as seen in the total syntheses of Hinckdentine A4 and Isatisine A5 respectively.
3-Oxindole 2 can be synthesized easily in large scale similar to the procedure developed by Dropinski,12 where an initial N-alkylation is followed by a Dieckmann condensation13 to produce 2-carboxymethyl-3-oxindole 2 (Scheme 1).
The coupling of 2 to indoles proved to be both simple and general (Table 1). The procedure for this mild oxidative coupling of indole is as follows: to a solution of the indole (2 eq), NaHCO3 (3 eq) and 3-oxindole in MeCN, at zero degrees, is added CAN (2 eq), the reaction is allowed to stir at ambient temperature overnight followed by aqueous work-up.
The reaction proved very robust with regards to both electronic factors and substitution patterns. With unsubstituted indole the reaction was performed on gram scale and similar yields were obtained. N-methylated indoles (see 4 in Table 1) reacted in good yield under these conditions, while N-substituted indoles did not give any coupling products for our earlier oxidative couplings.1b 2- and 3-substituted indoles produced the expected products, 5 and 6, respectively, where 6 is the 2-substituted product as the 3-position is already occupied. Using electron withdrawing groups (7, 8) high yields are observed whereas for electron donating groups (9) a low yield was observed. A similar trend is seen for the electron rich pyrrole (11). For halogen substituents (10) a good yield is observed.
As shown in Scheme 2, when either the nitrogen or the oxygen of the 3-oxindole is acetylated, 13 and 14 respectively, no reaction is observed. On the other hand, alkylation of the nitrogen in the 3-oxindole (12) is tolerated and a high yield of the ensuing product is observed, which is similar to the yield observed for the 3-oxindole 2.
We must therefore assume that the lone pair on nitrogen in the 3-oxindole 2 must play an important role in this mild oxidative coupling, when neither simple malonates nor N-acylated 3-oxindoles participate in this reaction. Other oxidants where tried for this oxidative coupling, including Mn(OAc)3, DDQ and K3Fe(CN)6 – none of which produced any product. Only from the reaction with Cu(II)-2-ethylhexanoate as the oxidant could product be observed, but only in very low yield (8%).
We must therefore assume that the lone pair on nitrogen in the 3-oxindole 2 must play an important role in this mild oxidative coupling, when neither simple malonates nor N-acylated 3-oxindoles participate in this reaction. Other oxidants where tried for this oxidative coupling, including Mn(OAc)3, DDQ and K3Fe(CN)6 – none of which produced any product. Only from the reaction with Cu(II)-2-ethylhexanoate as the oxidant could product be observed, but only in very low yield (8%).
In summary, an extension of our existing oxidative couplings of indoles has been developed. The reaction tolerates a variety of functional groups, proceeds readily at ambient conditions, and no prefunctionalisation is required. The oxidant is readily available and cheap, and the reaction can easily be performed on large scale.
EXPERIMENTAL
Methyl 2-(2-methoxy-2-oxoethylamino)benzoate 1, colorless oil: Methyl antranilate (5.00 mL, 38.6 mmol) and anhydrous Na2CO3 (4.20 g, 39.6 mmol) was dissolved in anhydrous DMF (40 ml). Methyl α-bromoacetate (5.00 mL, 52.6 mmol) was added and the reaction mixture was heated to 80oC for 20 hours. The mixture was then poured into H2O and extracted with Et2O. The organic phase was washed with Brine and dried with MgSO4, filtered and concentrated to yield pure diester 1 (7.2 g) in 83% yield.
1H NMR (400 MHz, CDCl3): δ 8.18 (s, 1 H), 7.93 (dd, 1 H, J = 1.7Hz, 8.0Hz), 7.36 (m, 1 H), 6.66 (ddd, 1H, J = 1.1Hz, 7.2Hz, 8.2Hz), 6.53 (d, 1 H, J = 8.5Hz), 4.02 (d, 2 H, J = 5.5Hz), 3.87 (s, 3 H), 3.79 (s, 3 H), which corresponds to the litterature.12
Methyl 3-hydroxy-1H-indole-2-carboxylate 2, off-white solid: Diester 1 (5.6 g, 25.1 mmol) was dissolved in anhydrous THF (79 mL) and cooled to 0 oC. Potasium tert-butoxide (3.0 g, 26.7 mmol, 1.1 eq) was dissolved in anhydrous THF (25 mL) and added dropwise to the cold solution via canula, the reaction mixture turned dark red. The reaction was monitored by TLC and after 3 hours the mixture was concentrated to remove most of the THF. H2O/AcOH (4:1) (50 mL) was added and the product was extracted with CH2Cl2. The organic phase was washed with Brine and dried over MgSO4, before being filtered and concentrated. Flash column chromatography Et2O /hexanes (1:1) yielded pure 3-oxindole 2 (4.0 g) in 82% yield.
1H NMR (400 MHz, CDCl3): δ 7.77 (bs, 1 H), 7.75 (dd, 1H, J = 1.1Hz, 8.1Hz), 7.35 (m, 1 H), 7.27 (m, 1 H), 7.10 (m, 1 H), 3.97 (s, 3 H), which corresponds to the litterature.12
Methyl 3-oxo-2,3'-biindoline-2-carboxylate 3, yellow solid: 1H NMR (600 MHz, CDCl3): δ 8.22 (s, 1 H), 7.70 (dd, 1 H, J = 0.8Hz, 7.5Hz), 7.59 (d, 1 H, J = 8.0Hz), 7.53 (ddd, 1 H, J = 1.3Hz, 7.2Hz, 8.3Hz), 7.40 (d, 1 H, J = 2.6Hz), 7.37 (d, 1 H, J = 8.2Hz), 7.21 (t, 1 H, J = 7.6Hz), 7.11 (t, 1 H, J = 7.6Hz), 7.01 (d, 1 H, J = 8.2Hz), 6.94 (t, 1 H, J = 7.4Hz), 5.73 (s, 1 H), 3.81 (s, 3 H) ppm; 13C NMR (150 MHz, CDCl3): δ 194.8, 169.1, 161.2, 138.1, 136.6, 125.6, 123.6, 122.8, 120.0, 119.6, 113.7, 111.8, 111.7, 72.5, 53.9 ppm; IR (neat) 3367, 1698, 1614, 1467, 1235 cm-1; mp 108 oC; HRMS (EI), calcd for C18H14N2O3 +H+ 307.1079, found: 307.1077.
Methyl 1'-methyl-3-oxo-2,3'-biindoline-2-carboxylate 4, yellow solid: 1H NMR (600 MHz, CDCl3): δ 7.70 (d, 1 H, J = 7.8Hz), 7.57 (d, 1 H, J = 8.0Hz), 7.52 (t, 1 H, J = 7.7Hz), 7.31 (d, 1 H, J = 8.2Hz), 7.27 (d, 1 H, J = 6.2Hz), 7.24 (t, 1 H, J = 7.6Hz), 7.11 (t, 1 H, J = 7.5Hz), 6.99 (d, 1 H, J = 8.3Hz), 6.93 (t, 1 H, J = 7.4Hz), 5.76 (s, 1 H), 3.81 (s, 3 H), 3.74 (s, 3 H); 13C NMR (150 MHz, CDCl3): δ 194.9, 169.1, 161.1, 138.0, 137.4, 128.1, 126.0, 125.5, 122.3, 120.4, 120.1, 199.9, 119.5, 113.7, 109.9, 109.9, 72.5, 53.9, 33.0 ppm; IR (neat) 3363, 2951, 1739, 1698, 1614, 1485, 1467, 1325, 1238 cm-1; mp 82 oC; HRMS (EI), calcd for C19H16N2O3 +H+ 321.1234, found: 321.1230.
Methyl 2'-methyl-3-oxo-2,3'-biindoline-2-carboxylate 5, yellow solid: 1H NMR (600 MHz, CDCl3): δ 8.01 (s, 1 H), 7.72 (d, 1 H, J = 7.7Hz), 7.53 (t, 1 H, J = 7.3Hz), 7.23 (d, 1 H, J = 8.1Hz), 7.15 (d, 1 H, J = 8.1Hz), 7.09 (t, 1 H, J = 7.6Hz), 6.98 (m, 2 H), 6.94 (t, 1 H, J = 7.5Hz), 5.55 (s, 1 H), 3.86 (s, 3 H), 2.25 (s, 3 H) ppm; 13C NMR (150 MHz, CDCl3): δ 195.5, 169.8, 161.3, 138.1, 135.0, 133.5, 127.2, 125.4, 121.8, 120.4, 120.3, 119.9, 118.2, 113.4, 110.8, 107.2, 73.3, 54.1, 13.6 ppm; IR (neat) 3353, 2923, 1696, 1615, 1487, 1461, 1432, 1325, 1236 cm-1; mp 168 oC; HRMS (EI), calcd for C19H16N2O3 +H+ 321.1234, found: 321.1231.
Methyl 3'-methyl-3-oxo-2,2'-biindoline-2-carboxylate 6, orange solid: 1H NMR (600 MHz, CDCl3): δ 9.38 (s, 1 H), 7.67 (d, 1 H, J = 7.8Hz), 7.55 (m, 2 H), 7.36 (d, 1 H, J = 8.1Hz), 7.19 (ddd, 1 H, J = 1.1Hz, 7.1Hz, 8.1Hz), 7.10 (m, 2 H), 6.95 (t, 1 H, J = 7.4Hz), 5.85 (s, 1 H), 3.79 (s, 3 H), 2.41 (s, 3 H); 13C NMR (150 MHz, CDCl3): δ 194.1, 167.4, 161.7, 138.5, 135.01, 129.0, 126.2, 125.7, 122.6, 121.0, 119.4, 119.4, 118.7, 113.9, 111.3, 109.8, 72.1, 54.3, 9.5 ppm; IR (neat) 3385, 2923, 2360, 2342, 1736, 1698, 1614, 1487, 1468, 1329, 1240 cm-1; mp 144 oC; HRMS (EI), calcd for C19H16N2O3 +H+ 321.1234, found: 321.1233.
Dimethyl 3-oxo-2,3'-biindoline-2,4'-dicarboxylate 7, yellow solid: 1H NMR (500 MHz, CDCl3): δ 8.91 (s, 1 H), 7.76 (dd, 1 H, J = 0.7Hz, 7.5Hz), 7.71 (d, 1 H, J = 7.8Hz), 7.46 (t, 1 H, J = 7.6Hz), 7.29 (dd, 1 H, J = 0.7Hz, 8.0Hz), 7.03 (m, 2 H), 6.88 (d, 1 H, J = 8.3Hz), 6.83 (m, 2 H), 3.92 (s, 3 H), 3.66 (s, 3 H) ppm; 13C NMR (125 MHz, CDCl3): δ 196.5, 169.9, 161.3, 138.3, 138.1, 128.1, 125.5, 124.2, 124.2, 122.5, 121.2, 119.1, 118.8, 117.3, 113.0, 112.0, 77.4, 74.1, 53.2, 52.4 ppm; IR (neat) 3376, 2951, 1733, 1696, 1617, 1488, 1437, 1330, 1267 cm-1; mp 235 oC; HRMS (EI), calcd for C20H16N2O5 +H+ 365.1132, found: 365.1133.
Methyl 5'-cyano-3-oxo-2,3'-biindoline-2-carboxylate 8, yellow solid: 1H NMR (600 MHz, CDCl3): δ 8.65 (s, 1 H), 8.11 (s, 1 H), 7.68 (d, 1 H, J = 7.7Hz), 7.59 (t, 1 H, J = 7.7Hz), 7.57 (d, 1 H, J = 2.6Hz), 7.39 (m, 2 H), 7.09 (d, 1 H, J = 8.2Hz), 6.99 (t, 1 H, J = 7.4Hz), 5.72 (s, 1 H), 3.84 (s, 3 H) ppm; 13C NMR (150 MHz, CDCl3): δ 194.3, 168.5, 161.3, 138.4, 138.3, 126.4, 126.0, 125.7, 125.6, 125.3, 121.2, 120.7, 119.8, 114.0, 112.7, 112.6, 103.7, 72.4, 54.2 ppm; IR (neat) 3277, 2221, 1736, 1689, 1615, 1590, 1491, 1467, 1431, 1332, 1293, 1241 cm-1; mp 146 oC; HRMS (EI), calcd for C19H13N3O3 +H+ 332.1035, found: 332.1022.
Methyl 6'-methoxy-3-oxo-2,3'-biindoline-2-carboxylate 9, yellow solid: 1H NMR (600 MHz, CDCl3): δ 8.09 (s, 1 H), 7.69 (d, 1 H, J = 7.8Hz), 7.53 (ddd, 1 H, J = 1.3Hz, 7.2Hz, 8.3Hz), 7.45 (d, 1 H, J = 8.8Hz), 7.00 (d, 1 H, J = 8.2Hz), 6.93 (t, 1 H, J = 7.4Hz), 6.83 (d, 1 H, J = 2.2Hz), 6.77 (dd, 1 H, J = 2.3Hz, 8.8Hz), 5.69 (s, 1 H), 3.81 (s, 3 H), 3.80 (s, 3 H) ppm; 13C NMR (150 MHz, CDCl3): δ 194.8, 169.1, 161.1, 156.9, 138.0, 137.5, 125.5, 122.4, 120.5, 120.3, 120.0, 119.8, 113.7, 111.8, 110.6, 95.0, 72.5, 55.8, 53.9 ppm; IR (neat) 3377, 2922, 1737, 1618, 1488, 1466, 1250 cm-1; mp 85 oC; HRMS (EI), calcd for C19H16N2O4 +H+ 337.1183, found: 337.1180.
Methyl 7'-bromo-3-oxo-2,3'-biindoline-2-carboxylate 10, yellow solid: 1H NMR (600 MHz, CDCl3): δ 8.43 (s, 1 H), 7.69 (d, 1 H, J = 7.7Hz), 7.59 (d, 1 H, J = 8.1Hz), 7.54 (ddd, 1 H, J = 1.3Hz, 7.2Hz, 8.3Hz), 7.48 (d, 1 H, J = 2.6Hz), 7.35 (d, 1 H, J = 7.6Hz), 7.02 (d, 1 H, J = 8.3Hz), 6.99 (t, 1 H, J = 7.8Hz), 6.94 (t, 1 H, J = 7.4Hz), 5.73 (s, 3 H) ppm; 13C NMR (150 MHz, CDCl3): δ 194.5, 168.8, 161.2, 138.1, 135.3, 126.7, 125.6, 125.1, 124.2, 121.6, 120.7, 119.9, 119.2, 113.7, 113.0, 105.2, 72.5, 54.0 ppm; IR (neat) 3354, 2974, 1738, 1696, 1614, 1488, 1467, 1433, 1323, 1231 cm-1; mp 101 oC; HRMS (EI), calcd for C18H13BrN2O3 +H+ 385.0182, found: 385.0187.
Methyl 2-(3,5-dimethyl-1H-pyrrol-2-yl)-3-oxoindoline-2-carboxylate 11, yellow solid: 1H NMR (600 MHz, CDCl3): δ 8.92 (s, 1 H), 7.62 (d, 1 H, J = 7.7Hz), 7.52 (t, 1 H, J = 7.7Hz), 7.02 (d, 1 H, J = 8.2Hz), 6.91 (t, 1 H, J = 7.4Hz), 5.66 (d, 1 H, J = 2.8Hz), 5.64 (s, 1 H), 3.78 (s, 3 H), 2.21 (s, 3 H), 2.09 (s, 3 H) ppm; 13C NMR (150 MHz, CDCl3): δ 194.8, 168.1, 161.6, 138.3, 127.3, 125.6, 120.6, 119.5, 117.6, 117.6, 113.7, 109.7, 71.9, 54.1, 13.1, 12.2 ppm; IR (neat) 3375, 2921, 1698, 1616, 1487, 1468, 1326, 1231 cm-1; mp 165 oC; HRMS (EI), calcd for C16H16N2O3 +H+ 285.1234, found: 285.1236.
ACKNOWLEDGEMENTS
We thank Dr. D. H. Huang and Dr. L. Pasternack for NMR spectroscopic and Dr. G. Siuzdak for mass spectrometric assistance. Financial support for this work was provided by the NSF and Bristol-Myers Squibb. We are grateful to the Danish Research Council for a postdoctoral stipend (09-064968/FNU) (M.J.).
References
1. P. S. Baran and J. M. Richter, J. Am. Chem. Soc., 2004, 126, 7450; CrossRef J. M. Richter, B. W. Whitefield, T. J. Maimone, D. W. Lin, M. P. Castroviejo, and P. S. Baran, J. Am. Chem. Soc., 2007, 129, 12857. CrossRef
2. P. S. Baran, J. M. Richter, and D. W. Lin, Angew. Chem. Int. Ed., 2005, 44, 609. CrossRef
3. P. S. Baran and M. P. DeMartino, Angew. Chem. Int. Ed., 2006, 45, 7083; CrossRef M. P. DeMartino, K. Chen, and P. S. Baran, J. Am. Chem. Soc., 2008, 130, 11546. CrossRef
4. K. Higuchi, Y. Sato, M. Tsuchimochi, K. Sugiura, M. Hatori, and T. Kawasaki, Org. Lett., 2009, 11, 197; CrossRef K. Higuchi, Y. Sato, S. Kojima, M. Tsuchimochi, K. Sugiura, M. Hatori, and T. Kawasaki, Tetrahedron, 2010, 66, 1236. CrossRef
5. A. Karadeolian and M. A. Kerr, Angew. Chem. Int. Ed., 2010, 49, 1133. CrossRef
6. H. H. Wasserman, J. D. Cook, and C. B. Vu, J. Org. Chem., 1990, 55, 1701. CrossRef
7. L. Ke-Qing, Synth. Commun., 1996, 26, 149. CrossRef
8. S.-F. Wang and C.-P. Chuang, Heterocycles, 1997, 45, 347; CrossRef C.-P. Chuang and S.-F. Wang, Tetrahedron Lett., 1994, 35, 1283; CrossRef J. Magolan and M. A. Kerr, Org. Lett., 2006, 8, 4561; CrossRef J. Magolan, C. A. Carson, and M. A. Kerr, Org. Lett., 2008, 10, 1437; CrossRef A.-I. Tsai, C.-H. Lin, and C.-P. Chuang, Heterocycles, 2005, 65, 2381. CrossRef
9. E. Baciocchi and E. Muraglia, J. Org. Chem., 1993, 58, 7610. CrossRef
10. R. Gibe and M. A. Kerr, J. Org. Chem., 2002, 67, 6247. CrossRef
11. L. M. Weinstock, E. Corley, N. L. Abramson, A. O. King, and S. Karady, Heterocycles, 1988, 27, 2627. CrossRef
12. J. F. Dropinski, T. Akiyama, M. Einstein, B. Habulihaz, T. Doebber, J. P. Berger, P. T. Meinke, and G. Q. Shi, Bioorg. Med. Chem. Lett., 2005, 15, 5035. CrossRef
13. W. Dieckmann, Chem. Ber., 1894, 27, 102; CrossRef W. Dieckmann, Ann., 1901, 317, 27.