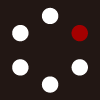
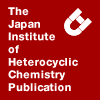
HETEROCYCLES
An International Journal for Reviews and Communications in Heterocyclic ChemistryWeb Edition ISSN: 1881-0942
Published online by The Japan Institute of Heterocyclic Chemistry
e-Journal
Full Text HTML
Received, 1st July, 2010, Accepted, 13th August, 2010, Published online, 16th August, 2010.
DOI: 10.3987/COM-10-S(E)90
■ Reversible Dehydrogenation-Hydrogenation of Tetrahydroquinoline-Quinoline Using a Supported Cooper Nanoparticle Catalyst
Yusuke Mikami, Kaori Ebata, Takato Mitsudome, Tomoo Mizugaki, Koichiro Jitsukawa, and Kiyotomi Kaneda*
Research Center for Solar Energy Chemistry, Graduate School of Engineering Science, Osaka University, 1-3 Machikaneyama, Toyonaka, Osaka 560-8531, Japan
Abstract
Copper nanoparticles synthesized on a titania surface (Cu/TiO2) act as an efficient heterogeneous catalyst for the reversible dehydrogenation and hydrogenation of tetrahydroquinoline-quinoline under an atmospheric pressure of H2.INTRODUCTION
Hydrogen has been proposed as a clean energy source and an alternative to fossil fuels for next-generation automobile and fuel cells, because only water is formed as a by-product after the consumption of hydrogen.1 For a hydrogen-based energy infrastructure, efficient and safe storage, transportation, and supply of hydrogen are crucial issues.2 Among the several approaches to accomplish this, the organic hydride strategy has attracted much attention as a promising method, wherein H2 is stored or released from organic molecules through reversible hydrogenation and dehydrogenation using catalysts.3 The organic hydride method has significant advantages such as the safe use of recyclable organic hydrides, good handling, and no emission of CO, CO2 or other by-products. Although reversible hydrogenation and dehydrogenation using cyclohexane/benzene and decalin/naphthalene pair systems have been reported, severe conditions of high temperature (> 200 oC) and high H2 pressure (30-80 atm) are required in these systems.3a-e
Recently, Pez and Crabtree discovered that the incorporation of nitrogen atoms into the aromatic rings facilitated the dehydrogenation process.4 Heteroaromatics such as carbazole and 1,2,3,4-tetrahydroquinoline were easily dehydrogenated under a milder temperature (120 oC) using Pd/C and Rh/C catalysts. These catalyst systems, however, still required a high H2 pressure (50 atm) in the hydrogenation process. While successful dehydrogenation systems of nitrogen heterocycles have been reported,4,5 few catalytic systems for reversible dehydrogenations are known and these systems still suffer from the need for severe reaction conditions and the use of expensive metals such as Pt, Pd or Ir.6 Thus, the development of reusable and less expensive base-metal catalysts for efficient reversible dehydrogenation and hydrogenation systems is highly desirable from the ecological and practical points of view.
Herein, we report that copper nanoparticles synthesized on a titania surface act as an efficient heterogeneous catalyst for the reversible dehydrogenation and hydrogenation of tetrahydroquinoline/quinoline. This hydrogen storage system can be operated under mild conditions (Scheme 1). This is the first example of a reversible organic hydride system using a heterogeneous catalyst with an atmospheric pressure of H2.
RESULTS AND DISCUSSIONS
TiO2-supported copper nanoparticles (Cu/TiO2) were prepared as follows. TiO2 (JRC-TIO-4) (1.0 g) was added to an aqueous solution of copper (II) trifluoromethanesulfonate (16 M), and then the mixture was stirred at 25 oC for 2 min. Next, a 25 wt% aqueous NH3 solution was slowly dropped into the mixture to adjust the pH to 8.0 while stirring. After stirring for 1 h, the slurry was filtered, washed with deionized water, and dried at room temperature in vacuo, providing divalent copper species on TiO2 (CuII/TiO2) as a light grey powder. The resulting CuII/TiO2 was reduced with 1 atm of H2 to afford Cu/TiO2 as a dark powder (Cu: 4.91 wt%). UV spectra of Cu/TiO2 showed an absorption around 572-575 nm, which is characteristic of the plasmon band of a zero valent copper species.7
Initially, Cu/TiO2 was examined in the dehydrogenation of 1,2,3,4-tetrahydroquinoline (1) at 150 oC under Ar flow for 16 h. 1 was dehydrogenated to give quinoline (2) in 99% yield and H2 was generated in two equimolar amounts to 2. Other inorganic materials-supported copper nanoparticles of Cu/Al2O3, Cu/SiO2, Cu/MgO, and Cu/Hydroxyapatite (Cu/HAP) were prepared in similar ways and tested in the dehydrogenation of 1. As summarized in Table 1, Cu/Al2O3 was also found to be an effective catalyst, whereas Cu/SiO2, Cu/HAP, and Cu/MgO showed lower activities.8 The present dehydrogenation system using Cu/TiO2 was applicable to scale-up conditions (20-mmol scale of 1) and an identical yield of 2 could be obtained (entry 2). In heterogeneous catalysts in the liquid-phase reaction, leaching of the active metal species from the support into the reaction solvent is a significant issue. In the course of the dehydrogenation of 1 using Cu/TiO2, the solid Cu/TiO2 was filtered off at a 50% conversion of 1. Further stirring of the filtrate under similar reaction conditions did not afford any products, showing that the dehydrogenation took place on the surface of Cu/TiO2. In addition, no copper ions in the filtrate were confirmed by ICP analysis (detection limit: 0.054 ppm ).
Next, the hydrogenation of 2 into 1 was carried out using Cu/TiO2. We found that 2 could be successfully hydrogenated with Cu/TiO2 at 150 oC under 5 atm of H2 in mesitylene for 3 h, affording 1 in 92% yield. It is noted that the hydrogenation quantitatively proceeded even under an atmospheric pressure of H2 (Scheme 2). The previously reported systems using copper catalysts required high pressure (> 30 atm) and/or temperature to achieve a high yield of 1.9 From the above results, Cu/TiO2 was found to be an effective catalyst for both the dehydrogenation of 1 and hydrogenation of 2.
To further investigate the reversibility of the present tetrahydroquinoline/quinoline system, successive dehydrogenation-hydrogenation of 1/2 using Cu/TiO2 was repeated (Table 2). After the quantitative dehydrogenation of 1 into 2 (entry 1), the Ar atmosphere was replaced with 1 atm of H2 and the reaction mixture was heated again and stirred at 150 oC. The above treatment gave the product 1 in 95% yield for 6 h, which is shorter reaction time than that of the dehydrogenation (entry 2). Molecular hydrogen could be released and stored using the present tetrahydroquinoline/quinoline catalyst system. These reversible dehydrogenation and hydrogenation reactions could be repeated with retention of high efficiency (entries 3 and 4).
CONCLUSION
In conclusion, we prepared TiO2-supported copper nanoparticles that acted as a heterogeneous catalyst for the reversible dehydrogenation and hydrogenation of tetrahydroquinoline/quinoline. The Cu/TiO2-catalyzed organic hydride system could be conducted under mild reaction conditions and repeated. This is the first report of a reversible organic hydride system using a heterogeneous catalyst with 1 atm of H2. This strategy will give a clue to the development of a highly efficient, practical organic hydride system.
EXPERIMENTAL
1) General
All organic reagents were purified before use. Copper (II) trifluoromethanesulfonate was purchased from Tokyo Kasei Kogyo Co., Ltd. TiO2 (JRC-TIO-4), Al2O3 (JRC-ALO-3), SiO2 (JRC-SIO-4), MgO (JRC-MGO-1) were obtained from the Catalysis Society of Japan as reference catalysts. Hydroxyapatite (HAP) was purchased from Wako Pure Chemical Industries, Ltd. Inductively coupled plasma measurement was performed by SII NanoTechnology SPS7800. 1H and 13C nuclear magnetic resonance (NMR) spectra were recorded on JEOL JNM-AL400 and JNM-GSX270 spectrometers, respectively. Gas chromatography (GC-FID) was carried out a Shimadzu GC-2014 equipped with a KOCL-3000T column (2 m).
2) Characterization of Cu/TiO2
Elemental analysis showed that the copper loading was 4.91 wt%. UV-vis spectra were recorded on a JASCO V-550 spectrometer equipped with integration spheres at room temperature. BaSO4 was used as a standard reflection sample.
3) Typical reaction procedure
3-1) Dehydrogenation of 1,2,3,4-Tetrahydroquinoline (1)
A typical procedure for the dehydrogenation of 1 by Cu/TiO2: CuII/TiO2 (0.13 g, 0.01 mmol of Cu) was placed in a Schlenk tube equipped with a reflux condenser. The vessel was evacuated and filled with 1 atm of molecular hydrogen (H2) and the solid catalyst was stirred at 180 oC for 0.5 h. After replacing the H2 with Ar, a mineral oil bubbler was attached to the top of the condenser, mesitylene (5 mL) and 1 (0.5 mmol) were added, and the reaction mixture was vigorously stirred at 150 oC under a stream of Ar for 16 h. The solid catalyst was removed by centrifugation and the yield was determined by GC analysis with naphthalene as an internal standard [Shimadzu GC-8A; carrier gases: N2 (50 kPa), H2 (50 kPa), and air (50 kPa); column: 5% KOCL-3000T (5.0 m); oven temperature program: from 100 oC, 10 oC/min up to 220 oC, hold for 10 min; injection and detection temperature: 270 oC; retention time: 1 (10.9 min), 2 (9.3 min), naphthalene (7.16 min)].
3-2) Hydrogenation of quinoline (2)
A typical procedure for the hydrogenation of 2 by Cu/TiO2: CuII/TiO2 (0.13 g, 0.01 mmol of Cu) was placed in a Schlenk tube equipped with a reflux condenser. The vessel was evacuated and filled with 1 atm of molecular hydrogen (H2) and the solid catalyst was stirred at 180 oC for 0.5 h. A balloon filled with hydrogen was connected to the top of the condenser. Mesitylene (5 mL) and 2 (0.5 mmol) were added, and the reaction mixture was vigorously stirred at 150 oC for 6 h. The solid catalyst was removed by centrifugation and the yield was determined by GC analysis with naphthalene as an internal standard.
3-3) Reversible dehydrogenation and hydrogenation between 1 and 2
A typical procedure for the dehydrogenation of 1 by Cu/TiO2: CuII/TiO2 (0.13 g, 0.01 mmol of Cu) was placed in a Schlenk tube equipped with a reflux condenser. The vessel was evacuated and filled with 1 atm of molecular hydrogen (H2) and the solid catalyst was stirred at 180 oC for 0.5 h. After replacing the H2 with Ar, a mineral oil bubbler was attached to the top of the condenser, mesitylene (5 mL) and 1 (0.5 mmol) were added, and the reaction mixture was vigorously stirred at 150 oC under a stream of Ar for 16 h. The yield of 2 was determined by GC analysis. Next, the atmosphere of the flask was replaced with 1 atm of H2. The mixture was magnetically stirred for 6 h at 150 oC. The yield of 1 was determined by GC analysis. The above procedures were repeated.
3-4) Measurement of H2
The dehydrogenation was carried out in a closed vessel under standard reaction conditions. After the reaction, the gas phase was subjected to GC-TCD for qualitative and quantitative analysis of H2. GC conditions were as follows: GC using thermal conductivity detector (Shimadzu GC-8A); column: molecular sieves 13X (4.0 m); oven temperature: 40 oC; injection and detection temperature: 70 oC; carrier gas: Ar (100 kPa); current: 60 mA; retention time: H2 (3.0 min). Evolution of H2 was also confirmed volumetrically using a gas buret attached to the reactors.
ACKNOWLEDGEMENTS
This investigation was supported by a Grant-in-Aid for Scientific Research from the Ministry of Education, Culture, Sports, Science, and Technology of Japan. This work was also supported by a Grant-in-Aid for Scientific Research on Priority Areas (No.18065016, "Chemistry of Concerto Catalysis") from the Ministry of Education, Culture, Sports, Science, and Technology, Japan. One of the authors Y.M. thanks the JSPS Research Fellowships for Young Scientists. Y. M. expresses his special thanks for The Global COE (center of excellence) Program “Global Education and Research Center for Bio-Environmental Chemistry” of Osaka University.
References
1. A. Sartbaeva, V. L. Kuznetsov, S. A. Wellsb, and P. P. Edwards, Energy Environ. Sci., 2008, 1, 79.
2. U. Eberle, M. Felderhoff, and F. Schuth, Angew. Chem. Int. Ed., 2009, 48, 6608. CrossRef
3. (a) N. Kariya, A. Fukuoka, and M. Ichikawa, Appl. Catal., A, 2002, 233, 91; (b) S. Hodoshima, S. Takaiwa, A. Shono, K. Satoh, and Y. Saito, Appl. Catal., A, 2005, 283, 235; (c) S. Hodoshima, H. Nagata, and Y. Saito, Appl. Catal., A, 2005, 292, 90; (d) Y. Okada, E. Sasaki, E. Watanabe, S. Hyodo, and H. Nishijima, Int. J. Hydrogen Energy, 2008, 31, 1348; CrossRef (e) R. B. Biniwale, S. Rayalu, S. Devotta, and M. Ichikawa, Int. J. Hydrogen Energy, 2008, 33, 360; CrossRef (f) D. Sebastián, E. G. Bordejé, L. Calvillo, M. J. Lázaro, and R. Moliner, Int. J. Hydrogen Energy, 2008, 33, 1329. CrossRef
4. (a) G. P. Pez, A. R. Scott, A. C. Cooper, H. Cheng, U.S. Pat. Appl. US2004/0223907 A1, 2004; (b) G. P. Pez, A. R. Scott, A. C. Cooper, H. Cheng, L. D. Bagzis, and J. B. Appleby, WO Appl. Pat. WO 2005/000457 A2, 2005; (c) G. P. Pez, A. R. Scott, A. C. Cooper, and H. Cheng, U.S. Patent 7,101,530, 2006; (d) E. Clot, O. Eisenstein, and R. H. Crabtree, Chem. Commun., 2007, 2231; CrossRef (e) R. H. Crabtree, Energy Environ. Sci., 2008, 1, 134.
5. (a) E. Balogh-Hergovich and Gabor Speier, J. Mol. Catal., 1986, 37, 309; CrossRef (b) S. L. Vorobeva, V. N. Buyanov, and N. N. Suvorov, Chem. Heterocycl. Comp., 1991, 27, 636; CrossRef (c) U. Tilstam, M. Harre, T. Heckrodt, and H. Weinmann, Tetrahedron Lett., 2001, 42, 5385. CrossRef
6. (a) A. Moores, M. Poyatos, Y. Luo, and R. H. Crabtree, New J. Chem., 2006, 30, 1675; CrossRef (b) Y. Cui, S. Kwok, A. Bucholz, B. Davis, R. A. Whitney, and P. G. Jessop, New J. Chem., 2008, 32, 1027; CrossRef (c) R. Yamaguchi, C. Ikeda, Y. Takahashi, and K. Fujita, J. Am. Chem. Soc., 2009, 131, 8410. CrossRef
7. See experimental section (Figure 1S).
8. The solid support of TiO2 might contribute to the formation of highly-dispersed Cu nanoparticles on Cu/TiO2. See; J. A. Rodríguez, J. Evans, J. Graciani, J. Park, P. Liu, J. Hrbek, and J. F. Sanz, J. Phys. Chem. C., 2009, 113, 7364. CrossRef
9. H. Adkins and R. Connor, J. Am. Chem. Soc., 1931, 53, 1091. CrossRef