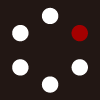
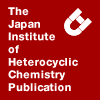
HETEROCYCLES
An International Journal for Reviews and Communications in Heterocyclic ChemistryWeb Edition ISSN: 1881-0942
Published online by The Japan Institute of Heterocyclic Chemistry
e-Journal
Full Text HTML
Received, 14th June, 2010, Accepted, 3rd August, 2010, Published online, 5th August, 2010.
DOI: 10.3987/COM-10-S(E)58
■ Synthesis of a Chiral C3-Symmetric Bowl-Shaped Cyclohexapeptide Composed of Anthranilic Acid and Leucine
Motohiro Akazome,* Masashi Enzu, Yohei Goto, and Shoji Matsumoto
Faculty of Engineering, Chiba University, 1-33 Yayoi-cho, Inage-ku, Chiba 263-8522, Japan
Abstract
A chiral C3-symmetric bowl-shaped cyclohexapeptide (1), composed of anthranilic acid (2-aminobenzoic acid) and α-amino acid in an alternating sequence, was synthesized from 2-nitrobenzoic acid and leucine. The 1H and 13C NMR spectra of 1 suggest a chiral C3-symmetric bowl-shaped structure. Molecular mechanic calculation revealed that the cyclohexapeptide becomes a bowl-shaped structure when all three α-amino acid components have homochiral side chains.Molecular platforms with C3 symmetry are often used for asymmetric catalysis and molecular recognition.1 In host-guest chemistry, C3 symmetric bowl-shaped compounds are potential candidates for use as highly designed synthetic receptors. Simple cyclic triamides,2 chiral cyclotriveratrylene,3 cyclic tripeptides,4 and cyclic pseudopeptides5 have been reported as the typical C3 symmetric bowl-shaped structures. Among them, cyclic hexapeptides composed of 3-aminobenzoic acid and α-amino acid in an alternating sequence were demonstrated as potential host molecules (Figure 1, structure I).6 About two decades ago, an achiral bowl structure of cyclic hexapeptide, which was made of anthranilic acid (2-aminobenzoic acid) and glycine (α-amino acid), was reported by Feigel and Lugert (Figure 1, structure II ),7 but other α-amino acids were never applied to this bowl-shaped structure.
We report synthesis of a chiral hexapeptide 1 composed of alternating anthranilic acid and leucine in Figure 1. Although N-benzoyl amino acid is easily racemized in general coupling conditions, we suppressed racemization by the choice of a coupling reagent, 1-ethoxycarbonyl-2-ethoxy-1,2- dihydroquinoline (EEDQ), to synthesize cyclic hexapeptide having the enantiopure C3-symmetric bowl-shaped structure. The chiral C3-symmetric bowl-shaped structure of the compound (1) was supported by NMR spectra and molecular force field calculations.
The synthetic route of 1 is shown in Schemes 1 and 2. Instead of anthranilic acid, 2-nitrobenzolic acid was used because protection of the amino group is necessary prior to the coupling process of amino acids. Cyclic hexapeptide (1) was constructed from a common dipeptide intermediate, enantionpure methyl (S)-N-(2-nitrobenzoyl)leucinate (2), which was obtained by coupling of 2-nitrobenzoic acid and methyl leaucinate using DCC and 1-hydroxybenzotriazole (HOBt)8 (Scheme 1). Saponification and hydrogenation of 2 respectively afforded (S)-N-(2-nitrobenzoyl)leucine (3)9 and methyl (S)-N-(2-aminobenzoyl)leucinate (4) in high yield.
The cyclic hexapeptide (1) was synthesized by a coupling reaction of three dipeptide intermediates according to Scheme 2. When general coupling reagents such as 1-ethyl-3-(3-dimethylaminopropyl)carbodiimide hydrochloride (EDC) with HOBt10 or N,N’-carbonyldiimidazole (CDI)11 were used, severe racemization of N-terminal leucine occurred to give a diastereomeric mixture of 5. It is well-known that N-benzoyl amino acids are often racemized by formation of a 5(4H)-oxazolone intermediate under coupling conditions.12 Fortunately 1-ethoxycarbonyl-2-ethoxy-1,2-dihydroquinoline (EEDQ)13 afforded the corresponding peptide 5 without racemization. After reduction of the nitro group of 5, the amino compound 6 was coupled with 3 by the same conditions using EEDQ. The linear hexapeptide 7 was transformed to the cyclization precursor 8 by reduction and saponification. Cyclization was performed under high dilution conditions using a syringe pump. With EEDQ as a coupling reagent, trace amounts of 1 were obtained. Instead of EEDQ, cyclization by propylphosphonic acid anhydride (T3P)14 afforded the desirable cyclohexapeptide 1 in 69%. However direct synthesis of 1 from (S)-N-(2-aminobenzoyl)leucine with T3P was unsuccessful and the corresponding cyclic compound, namely benzodiazepine-2,5-dione, was mainly obtained.
In CDCl3, the 1H and 13C NMR spectra of 1 show only one-third of the peaks of the whole structure, which indicates that 1 has a high symmetric structure such as C3-symmetry. The variable temperature (VT) NMR study showed high symmetry peaks even at -55 oC in CDCl3. Two peaks (11.37 and 6.84 ppm in Figure 2a) of 1 were assigned to the amide protons of two kinds because both peaks disappeared by the H–D exchange in methanol-d4. Figure 2 shows that both chemical shifts of these amide protons of 1 are very close to those of the linear compound 7. Therefore, almost no breakage of hydrogen bonds resulted from conformational restriction along with the cyclization. In DMSO-d6, chemical shifts of these amides of 1 change to 11.56 and 8.98 ppm (not shown). These values are also close to the chemical shifts (10.92 and 8.88 ppm) of the achiral compound II in Figure 1.7 In comparison with CHCl3 solvent, DMSO as a strong hydrogen-bonding acceptor would form hydrogen bonds with amide protons of the leucine moiety to move the chemical shift to the downfield (Δδ = 2.14 ppm downfield shift). On the other hand, only 0.19 ppm downfield-shift of anthranilic amide protons was observed in DMSO-d6 because the protons would be strongly shielded by the prearranged six-membered hydrogen-bonding with the anthranilic amide oxygen atom.
We were not able to obtain suitable crystals for single-crystal X-ray analysis. To estimate the preferred conformation in solution, we performed a conformational search for 1 using Monte Carlo minimization procedures with the MMFF94s force field15 and GB/SA solvation treatment (CHCl3)16 as implemented in the MacroModel program.17 Three isobutyl groups of 1 generate many conformational isomers. Therefore, we replaced three isobutyl groups into three methyl groups on the molecular mechanics calculations of 1. Three conformers were found within 10 kJ/mol (Figure 3). The lowest-energy conformation is a perfect C3-symmetric bowl-shaped structure (conformer A). Judging from the sum of the van der Waals radii (2.72 Å; H, 1.20 Å; O, 1.52 Å),18 the conformer A has three weak five-membered hydrogen bonds in addition to three strong six-membered hydrogen bonds. The second lowest-energy conformation (conformer B), which is the slightly distorted structure of conformer A, loses two weak five-membered hydrogen bonds (+3.0 kJ/mol). Both conformers A and B project three methyl groups outside the bowl structure to prevent steric repulsion. However, the third lowest-energy conformation (conformer C) is not C3-symmetric. Conformer C without a five-membered hydrogen bond has higher energy (+9.8 kJ/mol).
To confirm the effect of alkyl side chains, we prepared two cyclohexapeptides 9 and 10, which are partially replaced leucine by glycine. These CD spectra of these compounds are summarized in Figure 4. The Cotton effect of 1 was notably stronger than those of two compounds 9 and 10. As these spectra show, the loss of only one side chain allowed greater flexibility of conformation to change of the population of the lower-energy conformers.
The CD spectra of 9 and 10 were mutually similar. Therefore, we performed additional molecular mechanics calculation of methyl analogs of 9 and 10 in Figure 5. It is particularly interesting that the major lower-energy conformers are common among 1, 9 and 10. The lowest-energy conformers of methyl analogs of 9 and 10 twisted conformer C in the opposite direction (conformer C’ in Figure 5). The next lowest-energy conformers resemble conformer C of 1 (+4.0 and +6.3 kJ/mol, respectively). But, conformational energies of the pseudo-C3-symmetric bowl are higher than those of the lowest conformations (C’) by +7.8 (conformers B) and +10.8 kJ/mol (conformers A), respectively. Consequently, the similarity of CD spectra of 9 and 10 would be attributed to common features and similar distribution of lower-energy conformers. These results also suggest that three side chains of 1 evenly enforce the cyclic hexapeptide ring to form a C3-symmetric bowl-shaped structure. In other words, the major conformer was not C3-symmetric if it were not for even one side chain of 1.
In conclusion, we synthesized a chiral C3 bowl-shaped hexapeptide composed of anthranilic acid and leucine in an alternating sequence. Their conformers in a solution state were estimated using NMR, CD, and molecular mechanic calculation. All three side chains of leucine evenly enforce the cyclic hexapeptide ring to form a chiral C3 bowl-shaped structure. This hexapeptide and the related analogue would become candidates having a chiral C3-symmetric cavity in the field of supramolecular chemistry.
EXPERIMENTAL
General Procedures. Melting points were determined with Yanaco MP-J3 and values were uncorrected. 1H (300 MHz) and 13C (75 MHz) NMR spectra were recorded on Varian GEMINI 2000 spectrometer with TMS as an internal standard. J-Values were given in Hz. IR spectra were measured on a JASCO FT/IR-350 spectrometer. Circular dichroism (CD) spectra were measured on a JASCO-J-820 spectrophotometer. Elemental analyses (EA) and high-resolution mass spectroscopy (HRMS) were carried out by the Chemical Analysis Center of Chiba University.
Methyl (S)-2-nitrobenzoylleucine (2). To a solution of (S)-methyl leucinate (18.2 g, 100 mmol), N,N-diidopropylethylamine (DIEA, 14.2 g, 110 mmol), and 2-nitrobenzoic acid (18.4 g, 110 mmol) in THF (200 mL) were added DCC (22.7 g, 110 mmol) and HOBt (14.9 g, 110 mmol) at 0 °C, and the mixture was stirred at 0 °C for 1 h and at rt for 25 h. The reaction mixture was concentrated in vacuo and added EtOAc (100 mL). The organic layer was washed with saturated aqueous NaHCO3 (100 mL), 1 M HCl (100 mL × 3), brine (100 mL) and dried over MgSO4. The solvent was evaporated to obtain 2 (22.3 g, 75.6 mmol, 76%) as yellow solid: mp 81.9-82.4 °C; [α]D25 -80.3 (c 1.00, MeOH); 1H NMR (CDCl3) δ 0.99 (d, J= 6.0 Hz, 3H), 1.04 (d, J= 6.3 Hz, 3H), 1.65-1.90 (m, 3H), 3.79 (s, 3H), 4.83-4.93 (m, 1H), 6.26 (d, J= 7.9 Hz, 1H), 7.57 (d, J= 7.3 Hz, 1H), 7.60 (dd, J= 6.2 and 7.9 Hz, 1H), 7.68 (dd, J= 7.3 and 7.0 Hz 1H), 8.07 (d, J= 8.1 Hz, 1H); IR (KBr) 3286, 3082, 2958, 2871, 1747, 1645, 1529, 1352, 744 (cm-1). MS (FAB): m/z = 295 [M + H]+, 317 [M + Na]+. HRMS Calcd for C14H18N2NaO5: [M + Na]+ 317.1104, found: m/z 317.1108. Anal. Calcd for C14H18N2O5: C, 57.13; H, 6.16; N, 9.52. Found: C, 57.10; H, 5.98; N, 9.92.
(S)-N-(2-Nitrobenzoyl)leucine (3).9 To a solution of 2 (14.1 g, 48.0 mmol) in THF (58 mL) was added 1 M LiOH aq. (58 mL), and the mixture was stirred at rt for 1 h. The reaction mixture was concentrated in vacuo. The solution was acidified (pH = 2) by using 1 M HCl, which was extracted by CHCl3 and dried over MgSO4. After drying in vacuo, 3 (12.2 g, 43.4 mmol, 90%) was obtained as a pale yellow solid. Crystallization form CHCl3/hexane afforded colorless needle crystals: mp 114.0-115.0 °C; [α]D25 -97.7 (c 1.00, MeOH); 1H NMR (DMSO-d6) δ 0.92 (d, J= 6.3 Hz, 6H), 1.50-1.80 (m, 3H), 4.30-4.60 (m, 1H), 7.57 (d, J= 7.0 Hz, 1H), 7.69 (dd, J= 7.7 and 7.7 Hz, 1H), 7.80 (dd, J= 7.7 and 7.0 Hz, 1H), 8.03 (d, J= 7.7 Hz, 1H), 8.94 (d, J= 7.7 Hz, 1H); IR (KBr) 3273, 3074, 2960, 2871, 1730, 1653, 1541, 1350, 741 (cm-1). HRMS Calcd for C13H16N2NaO5: [M + Na]+ 303.0950, found: m/z 303.0951.
Methyl (S)-(2-aminobenzoyl)leucinate (4). A mixture of 2 (7.36 g, 25.0 mmol) and 10% Pd-C (0.737 g) in THF (100 mL) was stirred at rt for 20 h under hydrogen atmosphere. After filtration, the reaction mixture was concentrated in vacuo to give 4 (6.55 g, 24.8 mmol, 99%) as colorless needle solid: mp 51.0-51.8 °C; [α]D25 -48.6 (c 1.00, MeOH); 1H NMR (CDCl3) δ 0.98 (d, J= 4.4 Hz, 3H), 0.99 (d, J= 5.0 Hz, 3H), 1.60-1.80 (m, 3H), 3.76 (s, 3H), 4.75-4.85 (m, 1H), 5.50 (br, 2H), 6.42 (d, J= 7.0 Hz, 1H), 6.67 (dd, J= 7.0 and 7.0 Hz, 1H), 6.68 (d, J= 7.0 Hz, 1H), 7.21 (dd, J= 7.6 and 8.2 Hz, 1H), 7.40 (d, J= 7.0 Hz, 1H); IR (KBr) 3473, 3367, 3033, 2956, 2870, 1736, 1633, 1583, 1531, 750 (cm-1). HRMS Calcd for C14H20N2NaO3: [M + Na]+ 287.1363, found: m/z 287.1366.
Methyl (S)-4-methyl-2-[2-[(S)-4-methyl-2-(2-nitrobenzamido)pentanamido]benzamido]-
pentanoate (5). To a solution of 3 (6.17 g, 22.0 mmol) and 4 (5.82 g, 22.0 mmol) in dry CHCl3 (30 mL) were added a solution of EEDQ (8.16 g, 33 mmol) in dry CHCl3 (20 mL) at 0 °C, and the mixture was stirred at 0 °C for 1 h and at rt for 16 h. The reaction mixture was concentrated in vacuo and added CHCl3 (20 mL). The organic layer was washed with 0.5 M HCl (30 mL × 4), saturated aqueous NaHCO3 (20 mL × 4), brine (30 mL) and dried over MgSO4. Evaporation in vacuo and column chromatography (silica gel; hexane : EtOAc = 1 : 1) provided 5 (5.40 g, 10.3 mmol, 47%) as pale yellow solid: mp 80.9-81.6 °C; [α]D25 -138.6 (c 0.10, MeOH); 1H NMR (CDCl3) δ 0.94-1.05 (m, 9H), 1.08 (d, J= 5.7 Hz, 3H), 1.68-1.90 (m, 6H), 3.77 (s, 3H), 4.79-4.89 (m, 2H), 6.51 (d, J= 7.5 Hz, 1H), 6.66 (d, J= 7.5 Hz, 1H), 7.14 (dd, J= 7.5 and 7.5 Hz, 1H), 7.48-7.61 (m, 3H), 7.68 (dd, J= 7.5 and 7.5 Hz, 1H), 7.77 (d, J= 7.5 Hz, 1H), 8.06 (d, J= 8.1 Hz, 1H), 8.56 (d, J= 8.4 Hz, 1H), 11.31 (s, 1H); IR (KBr) 3300, 3074, 2958, 2871, 1651, 1601, 1587, 1533, 1448, 1350, 758 (cm-1). MS (FAB): m/z = 527 [M + H] +, 549 [M + Na] +. HRMS Calcd for C27H34N4NaO7: [M + Na]+ 549.2313, found: m/z 549.2320. Anal. Calcd for C27H34N4O7 : C, 61.58; H, 6.51; N, 10.64. Found: C, 61.45; H, 6.53; N, 10.67.
Methyl (S)-2-[2-[(S)-2-(2-aminobenzamido)-4-methylpentanamido]benzamido]-4-methylpentanoate (6). A mixture of 5 (5.04 g, 9.57 mmol) and 10% Pd-C (0.504 g) in THF (50 mL) was stirred at rt for 21 h under hydrogen atmosphere. After filtration, the reaction mixture was concentrated in vacuo to give 6 (4.67 g, 94.1 mmol, 98%) as orange solid: mp 66.8-67.8 °C; [α]D25 –71.9 (c 1.00, MeOH); 1H NMR (CDCl3) δ 0.91-1.02 (m, 12H), 1.64-1.88 (m, 6H), 3.79 (s, 3H), 4.79-4.80 (m, 2H), 5.40 (br, 2H), 6.66-6.72 (m, 4H), 7.12 (dd, J= 7.8 and 7.2 Hz, 1H), 7.22 (dd, J= 7.2 and 8.1 Hz, 1H), 7.49 (dd, J= 7.8 and 7.2 Hz, 1H), 7.56 (dd, J= 8.1 and 8.4 Hz, 2H), 8.59 (d, J= 8.7 Hz, 1H), 11.36 (s, 1H); IR (KBr) 3461, 3350, 2956, 2870, 1734, 1652, 1522, 1448, 1260, 1160, 753 (cm-1). HRMS Calcd for C27H36N4NaO5: [M + Na]+ 519.2570, found: m/z 519.2578.
Methyl (S)-4-methyl-2-[2-[(S)-4-methyl-2-[2-[(S)-4-methyl-2-(2-nitrobenzamido)-
pentanamido]benzamido]pentanamido]benzamido]pentanoate (7). This compound was prepared as described above for compound 5 under general coupling conditions using EEDQ. The product 7 was isolated by column chromatography (silica gel; hexane : EtOAc = 1 : 1) in 43% yield as pale yellow solid: mp 116.0-117.2 °C, 1H NMR (CDCl3) δ 0.89-1.05 (m, 18H), 1.66-1.88 (m, 9H), 3.74 (s, 3H), 4.58-4.65 (m, 1H), 4.73-4.80 (m, 1H), 4.82-4.90 (m, 1H), 6.63 (d, J= 8.1 Hz, 1H), 6.75 (d, J= 8.1 Hz, 1H), 6.78 (d, J= 8.1 Hz, 1H), 7.12-7.19 (m, 2H), 7.43-7.73 (m, 6H), 7.92 (d, J= 6.3 Hz, 1H), 8.01 (d, J= 8.4 Hz, 1H), 8.49 (d, J= 8.4 Hz, 1H), 8.55 (d, J= 8.4 Hz, 1H), 11.26 (s, 1H), 11.32 (s, 1H); 13C NMR (CDCl3) δ 21.80, 21.96, 22.11, 22.77, 22.93, 23.12, 24.88, 24.97, 25.08, 41.35, 41.51, 41.88, 51.12, 52.62, 53.59, 53.75, 120.62, 121.22, 121.54, 122.00, 123.46, 123.48, 124.37, 126.76, 127.00, 129.28, 130.40, 132.66, 132.77, 132.87, 133.70, 138.66, 138.73, 146.55, 166.46, 168.54, 168.89, 170.60, 170.82 , 173.17; IR (KBr) 3299, 3068, 2958, 2871, 1741, 1650, 1600, 1587, 1533, 1448, 1348, 1288, 755 (cm-1). MS (FAB): m/z = 759 [M + H] +, 781 [M + Na] +. HRMS Calcd for C40H50N6NaO9: [M + Na]+ 781.3537, found: m/z 781.3575.
(S)-2-[2-[(S)-2-[2-[(S)-2-(2-Aminobenzamido)-4-methylpentanamido]benzamido]-4-methylpentanamido]benzamido]-4-methylpentanoic acid (8). A mixture of 7 (1.51 g, 1.99 mmol) and 10% Pd-C (0.152 g) in THF (30 mL) was stirred at rt for 18 h under hydrogen atmosphere. After filtration, the reaction mixture was concentrated in vacuo to give the corresponding reduced compound (1.35 g, 1.86 mmol, 93%) as pale yellow solid. To a solution of the resulted compound (0.499 g, 0.686 mmol) in THF (10 mL) was added 0.082 M LiOH aq. (10 mL), and the mixture was stirred at rt for 2.5 h. The reaction mixture was concentrated in vacuo. After neutralization by using 1M HCl, the deposited white solid was collected by filtration and dried in vacuo to give 8 as white solid (0.436 g, 0.610 mmol, 89%): mp 139.2-140.7 °C; 1H NMR (DMSO-d6) δ 0.70 (d, J= 4.8 Hz, 6H), 0.80-0.90 (m, 12H), 1.53-1.79 (m, 9H), 4.25-4.51 (m, 3H), 6.30 (br, 3H), 6.59 (dd, J= 7.2 and 7.2 Hz, 1H), 6.66 (d, J= 8.1 Hz, 1H), 7.11-7.18 (m, 3H), 7.45-7.52 (m, 2H), 7.75 (br, 1H), 7.90 (d, J= 8.4 Hz, 1H), 8.20 (d, J= 8.1 Hz, 1H), 8.51-8.58 (m, 3H), 8.63 (br, 1H), 9.29 (br, 1H), 11.68 (s, 1H), 11.84 (s, 1H). MS (FAB): m/z = 715 [M + H]+, 737 [M + Na]+.
(7S,16S,25S)-7,16,25-Triisobutyl-7,8,16,17,25,26-hexahydro-5H-tribenzo[e,l,s]-
[1,4,8,11,15,18]hexaazacyclohenicosine-6,9,15,18,24,27(14H,23H)-hexaone (1). To a solution of NMM (0.190 g, 1.88 mmol) and propylphosphonic acid anhydride (T3P in 50% EtOAc, 0.255 g, 0.800 mmol) in THF (130 mL) were added a solution of 8 (0.301 g, 0.400 mmol) in THF (20 mL) at rt over 6.5 h by a syringe pump, and the mixture was stirred for 40 h. The reaction mixture was concentrated in vacuo. The product 1 was isolated by column flash chromatography (silica gel; CHCl3 : hexane = 5 : 1) as white solid (0.191 g, 0.263 mmol, 69%): mp 190.8-191.7 °C; 1H NMR (CDCl3) δ 0.96-1.09 (m, 18H), 1.74-1.87 (m, 9H), 4.89-4.97 (m, 3H), 6.84 (d, J= 8.1 Hz, 3H), 7.12 (dd, J= 7.2 and 7.5 Hz, 3H), 7.45 (dd, J= 7.5 and 7.5 Hz, 3H), 7.52 (d, J= 7.8 Hz, 3H), 8.30 (d, J= 8.7 Hz, 3H), 11.37 (s, 3H); 13C NMR (CDCl3) δ 22.87, 22.98, 25.14, 43.39, 53.58, 121.69, 122.35, 123.81, 126.39, 132.41, 138.15, 167.88, 170.36; IR (KBr) 3314, 2957, 2870, 1647, 1599, 1529, 1448, 1369, 1337, 1287, 1168 (cm-1). MS (FAB): m/z = 697 [M + H]+, 719 [M + Na]+. HRMS Calcd for C39H49N6O6: [M + H]+ 697.3714, found: m/z 697.3736. Anal. Calcd for C39H48N6O6・0.45H2O: C, 66.45; H, 6.99; N, 11.92. Found: C, 66.23; H, 7.00; N, 11.74.
(7S,16S)-7,16-Diisobutyl-7,8,16,17,25,26-hexahydro-5H-tribenzo[e,l,s]-
[1,4,8,11,15,18]hexaazacyclohenicosine-6,9,15,18,24,27(14H,23H)-hexaone (9). According to the similar procedure mentioned above, the cyclic hexapeptide 9 was prepared from 6 and N-(2-nitrobenzoyl)glycine. The final cyclization using T3P afforded 9 as white solid (32%): mp 261.9-262.8 °C; 1H NMR (CDCl3) δ 0.92-1.05 (m, 12H), 1.65-1.89 (m, 6H), 4.58 (dd, J= 6.9 and 6.9 Hz, 2H), 4.83-4.94 (m, 2H), 6.49 (d, J= 9.0 Hz, 1H), 6.75 (d, J= 8.7 Hz, 1H), 6.86 (m, 1H), 7.09-7.17 (m, 3H), 7.45-7.57 (m, 6H), 8.24-8.32 (m, 3H), 11.05 (s, 1H), 11.25 (s, 1H), 11.35 (s, 1H); 13C NMR (CDCl3) δ 22.25, 22.43, 22.84, 22.91, 25.02, 25.19, 42.09, 42.77, 44.82, 53.28, 53.26, 121.55, 122.40, 122.42, 122.45, 122.49, 122.69, 123.18, 123.90, 124.05, 126.47, 126.52, 127.16, 132.30, 132.41, 132.63, 137.66, 137.90, 138.13, 167.25, 167.99, 168.19, 168.64, 170.66, 170.66; IR (KBr) 3294, 2958, 2870, 1647, 1601, 1588, 1521, 1449, 1288, 1167 (cm-1). MS (FAB): m/z = 641 [M + H]+, 663 [M + Na]+. HRMS Calcd for C35H40N6NaO6: [M + Na]+ 663.2907, found: m/z 663.2935. Anal. Calcd for C35H40N6O6・0.9H2O: C, 63.99; H, 6.41; N, 12.79. Found C, 63.89; H, 6.25; N, 12.51.
(S)-7-Isobutyl-7,8,16,17,25,26-hexahydro-5H-tribenzo[e,l,s][1,4,8,11,15,18]-
hexaazacyclohenicosine-6,9,15,18,24,27(14H,23H)-hexaone (10). According to the similar procedure mentioned above, the cyclic hexapeptide 10 was prepared from the achiral analog of 6 and 3. The final cyclization using T3P afforded 10 as white solid (20%): mp 272.0-274.0 °C; 1H NMR (CDCl3) δ 0.86 (d, J= 6.3 Hz, 6H), 1.48-1.67 (m, 3H), 3.89-3.99 (m, 2H), 4.69-4.85 (m, 3H), 6.17-6.21 (m, 1H), 6.60 (d, J= 9.9 Hz, 1H), 6.66-6.70 (m, 1H), 7.09 (dd, J= 7.5 and 7.8 Hz, 1H), 7.20 (dd, J= 7.5 and 7.5 Hz, 2H), 7.46-7.55 (m, 5H), 7.62 (d, J= 6.6 Hz, 1H), 8.09 (d, J= 7.8 Hz, 1H), 8.14 (d, J= 8.1 Hz, 1H), 8.56 (d, J= 8.7 Hz, 1H), 10.57 (s, 1H), 10.64 (s, 1H), 11.21 (s, 1H); IR (KBr) 3292, 2956, 2930, 1647, 1602, 1587, 1521, 1449, 1288 cm-1. MS (FAB): m/z = 585 [M + H]+, 607 [M + Na]+. HRMS Calcd for C31H33N6O6: [M + H]+ 585.2462, found: m/z 585.2450.
ACKNOWLEDGEMENTS
This work was supported by a Grant-in-Aid for Scientific Research (C) (No. 21550129) from the Japan Society for the Promotion Science.
References
1. S. E. Gibson and M. P. Castaldi, Angew. Chem. Int. Ed., 2006, 45, 4718; CrossRef C. Moberg, Angew. Chem. Int. Ed., 2006, 45, 4721; CrossRef C. Moberg, Angew. Chem. Int. Ed., 1998, 37, 248. CrossRef
2. K. Katagiri, T. Kato, H. Masu, M. Tominaga, and I. Azumaya, Cryst. Growth Des., 2009, 9, 1519; CrossRef F. Imabeppu, K. Katagiri, H. Masu, T. Kato, M. Tominaga, B. Therrien, H. Takayanagi, E. Kaji, K. Yamaguchi, H. Kagechika, and I. Azumaya, Tetrahedron Lett., 2006, 47, 413. CrossRef
3. A. Collet and J. Jacques, Tetrahedron Lett., 1978, 15, 1265; CrossRef A. Collet, J. Gabard, J. Jacques, M. Cesario, J. Guilhem, and C. Pascard, J. Chem. Soc., Perkin Trans. 1, 1981, 1630; CrossRef J. Canceill, A. Collet, J. Gabard, G. Gottarelli, and G. P. Spada, J. Am. Chem. Soc., 1985, 107, 1299. CrossRef
4. M. Akazome, J. Sukegawa, Y. Goto, and S. Matsumoto, Tetrahedron Lett., 2009, 50, 5382; CrossRef T. K. Chakraborty, S. Tapadar, T. V. Raju, J. Annapurna, and H. Singh, Synlett, 2004, 2484; CrossRef L.-S. Sonntag, S. Ivan, M. Langer, M. M. Conza, and H. Wennemers, Synlett, 2004, 1270; CrossRef G. Haberhauser, Synlett, 2004, 1003; CrossRef G. Haberhauer, T. Oeser, and F. Rominger, Chem. Commun. 2004, 2044; CrossRef G. Haberhauer and F. Rominger, Tetrahedron Lett., 2002, 43, 6335; CrossRef G. Haberhauer, L. Somogyi, and J. Rebek, Jr. Tetrahedron Lett., 2000, 41, 5013. CrossRef
5. K. H. Lee, D. H. Lee, S. Hwang, O. S. Lee, D. S. Chung, and J. -I. Hong, Org. Lett., 2003, 5, 1431; CrossRef W. C. Still, Acc. Chem. Res., 1996, 29, 155; CrossRef D. Q. McDonald and W. C. Still, J. Am. Chem. Soc., 1996, 118, 2073; CrossRef A. Borchardt and W. C. Still, J. Am. Chem. Soc., 1994, 116, 7467; CrossRef A. Borchardt and W. C. Still, J. Am. Chem. Soc., 1994, 116, 373; CrossRef J.-I. Hong, S. K. Namgoong, A. Bernardi, and W. C. Still, J. Am. Chem. Soc., 1991, 113, 5111. CrossRef
6. S. Kubik, J. Am. Chem. Soc., 1999, 121, 5846; CrossRef S. Kubik and R. Goddard, J. Org. Chem., 1999, 64, 9475; CrossRef H. Ishida, M. Suga, K. Donowaki, and K. Ohkubo, J. Org. Chem., 1999, 64, 9475. CrossRef
7. M. Feigel and G. Lugert, Liebigs Ann. Chem., 1989, 1089. CrossRef
8. W. König and R. Geiger, Chem. Ber., 1970, 103, 788. CrossRef
9. U. Nagai and M. Kurumi, Chem. Pharm. Bull., 1970, 18, 831.
10. S. Nozaki and I. Muramatsu, Bull. Chem. Soc. Jpn., 1982, 55, 2165. CrossRef
11. R. Paul and G. W. Anderson, J. Am. Chem. Soc., 1960, 82, 4596; CrossRef G. W. Anderson and R. Paul, J. Am. Chem. Soc., 1958, 80, 4423. CrossRef
12. M. Bodanszky, 'Principles of Peptide Synthesis,' Springer-Verlag, Berlin Heidelberg, 1993, pp. 170-185.
13. B. Belleau and G. Malek, J. Am. Chem. Soc., 1966, 90, 1651. CrossRef
14. P. Lewer, P. R. Graupner, D. R. Hahn, L. L. Karr, D. O. Duebelbeis, J. M. Lira, P.B. Anzeveno, S. C. Fields, J. R. Gilbert, and C. Pearce, J. Nat. Prod., 2006, 69, 1506; CrossRef J. Klose, M. Beinert, C. M. Mollenkopf, D. Wehle, C.-w. Zhang, L. A. Carpino, and P. Henklein, Chem. Commun., 1999, 1847; CrossRef H. Wissmann and H.-J. Kleiner, Angew. Chem., Int. Ed. Engl., 1980, 19, 133. CrossRef
15. T. A. Halgren, J. Comput. Chem., 1999, 20, 720; CrossRef T. A. Halgren, J. Comput. Chem., 1999, 20, 730. CrossRef
16. W. C. Still, A. Tempczyk, R. C. Hawley, and T. Hendrickson, J. Am. Chem. Soc., 1990, 112, 6127. CrossRef
17. F. Mohamadi, N. G. J. Richards, W. C. Guida, R. Liskamp, M. Lipton, C. Caufield, G. Chang, T. Hendrickson, and W. C. Still, J. Comput. Chem., 1990, 11, 440; CrossRef G. Chang, W. C. Guida, and W. C. Still, J. Am. Chem. Soc., 1989, 111, 4379. CrossRef
18. A. Bondi, J. Phys. Chem., 1964, 68, 441. CrossRef