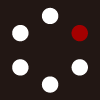
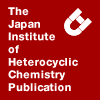
HETEROCYCLES
An International Journal for Reviews and Communications in Heterocyclic ChemistryWeb Edition ISSN: 1881-0942
Published online by The Japan Institute of Heterocyclic Chemistry
e-Journal
Full Text HTML
Received, 11th July, 2010, Accepted, 7th September, 2010, Published online, 8th September, 2010.
DOI: 10.3987/COM-10-S(E)95
■ Preparation of β-Amino Esters and β-Lactams from Nitriles via Aldimine-Borane Complexes
P. Veeraraghavan Ramachandran,* Debanjan Biswas, and Guang-Ming Chen
Herbert C. Brown Center for Borane Research, Department of Chemistry, Purdue University, 560 Oval Dr.; BRWN 5131
West Lafayette, IN 47907-2084, U.S.A.
Abstract
one-pot synthesis of β-amino esters has been achieved in 59-75% yield from aromatic and aliphatic nitriles via the condensation of the corresponding non-enolizable and enolizable aldimine-triethylborane complexes, respectively with methyl trimethylsilyl ketene acetals. Grignard-mediated lactamization of the intermediate β-amino esters provides the corresponding β-lactams in the same pot in 58-74% overall yield.The first synthesis of a β-lactam (azetidin-2-one) was reported a century ago by Staudinger via the [2+2]-cycloaddition of diphenylketene with benzylidene aniline.1 The interest in β-lactam molecules arose when the antibiotic properties of the first semi-synthetic penicillin, Penicillin G, were identified nearly seven decades ago.2 Since then other families of β-lactam antibiotics have been extensively developed and immensely used throughout the world to fight infectious pathogens.3 β-Lactams also serve as important building blocks for the preparation of various natural and synthetic antibiotics.4 In addition, they also serve as versatile intermediates for the synthesis of a variety of protein and non-protein amino acids, peptides, peptidomimetics, taxoid antitumor agents, heterocycles, and other types of compounds.5 Accordingly, the preparation of α-substituted β-lactams and β-amino acids is a topic of interest to organic and medicinal chemists.6
Diastereoselective alkylation to introduce substitution at the α-carbon has been an efficient method for the preparation of 3,3-dialkyl substituted β-amino acid derivatives.7 One of the general methods for the preparation of α-alkyl substituted β-lactams is the Staudinger cycloaddition reaction between Schiff bases8 and ketenes obtained from enantiomerically pure β-silyloxy- or β-(silyl)butyric acid chlorides.9
However, there have been very few reports on the successful preparation of α-substituted β-lactams starting from enolizable species.10 Birkofer and co-workers described the reaction between diphenylketene and N-trimethylsilylbenzaldimine, albeit in low yield, to produce the corresponding 2,2-diphenyl β-lactam.11 Condensation of N-trimethylsilylaldimines with the lithium ester enolates has also been reported to afford the corresponding β-lactams with syn-selectivity.12 The stereochemical outcome of these reactions has been reported to be dependent on the choice of imines and appropriate reaction conditions.
Colvin and coworkers reported the condensation of N-trimethylsilylaldimines with methyl trimethylsilyl dimethylketene acetal in presence of a molar equivalent of ZnI2 and t-BuOH to obtain β-amino ester intermediates.13 Lactamization of these intermediates mediated by methyl Grignard reagent provided 3,3-dimethyl β-lactams in moderate to good yield (Scheme 1). Unfortunately, only non-enolizable aldimines can be applied for such synthesis, due to the inaccessibility of enolizable N-trimethylsilylaldimine species. Yamamoto and coworkers have described the application of tris(pentafluorophenyl)boron as an efficient catalyst for the reaction between silyl ketene acetals and N-benzyl and N-silyl aldimines. The product β-amino esters were converted to the corresponding lactams.14
Suginome and coworkers reported a three-component Mannich-type reaction of silyl ketene acetals with aldehydes in the presence of aminoboranes or other amine sources for the preparation of β-amino esters.15 The reaction proceeds via the iminium salts derived from the aminoboranes, which undergo further reaction with the ketene acetals. A selective three-component reaction of aldimines and ethylbromoacetate via a modified rhodium-catalyzed Reformatsky-type reaction to form β-amino esters and lactams has been described by Honda and coworkers.16 They applied this reaction for the diasteroselective synthesis of chiral β-lactams.17 Ando and coworkers applied the Honda-Reformatsky reaction for accessing chiral difluoro-β-lactams by the treatment of ethyl bromodifluoroacetate with chiral 2-hydroxy-1-phenethyl group substituted imines (Scheme 2).18
Recently, we had reported the preparation of a series of stable enolizable and non-enolizable aldimine-trialkylborane complexes from the corresponding nitriles and utilized them for the preparation of asymmetric homoallylic amines, γ-substituted GABA analogs and γ-lactams.19,20 We envisaged a general methodology for the preparation of β-amino esters and β-lactams from these aldimine-borane complexes. The results of our study are presented herein.
A room temperature reaction of benzaldimine-triethylborane complex (2a), prepared by the partial reduction of benzonitrile (1a) with LiEt3BH (Super-Hydride™) in THF, followed by the addition of stoichiometric amount of methanol, with a molar equivalent of ZnI2 and methyl trimethylsilyl dimethylketene acetal resulted in an extremely slow reaction, requiring ~5 d for 40% completion, as determined by 11B NMR spectroscopy. Aqueous ammonium chloride workup, followed by chromatographic purification afforded the desired β-amino ester, methyl 3-amino-2,2-dimethyl-3-phenylpropanoate (3a), in a dismally low (15%) yield. The slow rate was presumed to be due to the presence LiOMe, generated during the preparation of the aldimine-borane complex, which renders the ZnI2 inactive. Filtration of LiOMe and carrying out the reaction in THF did not improve the rate. Replacing the solvent THF with diethyl ether enhanced the rate of the reaction considerably. Accordingly, preparation of 2a in THF was followed by the filtration of LiOMe, removal of THF and addition of Et2O. A molar equivalent of ZnI2 was added, followed by an equivalent of methyl trimethylsilyl dimethylketene acetal dissolved in Et2O. The condensation was complete within an hour. Aqueous workup with saturated NH4Cl solution provided, after chromatography, 71% yield of 3a (Scheme 3).
Encouraged by this result, we undertook a detailed study to optimize the stoichiometry of ZnI2 needed for the condensation of the ketene acetal with the aldimine-borane complex. Accordingly, the in-situ generated benzaldimine-triethylborane complex (2a) was treated with the ketene acetal in the presence of different molar ratios of the Lewis acid after filtering off LiOMe and replacing the solvent with ether. 20 mol% of ZnI2 achieved the condensation in 12 h. In the presence of 50 mol% of ZnI2 the condensation was completed in ~ 2 h and with stoichiometric quantity of the Lewis acid, the reaction was completed in less than 1 h at room temperature (Table 1). In all of the cases, the desired β-amino ester was isolated in comparable yields of 70-73%. Thus, under optimized conditions we utilized 0.5 equiv of ZnI2 for the condensation reaction.
Having standardized the reaction conditions, we extended this protocol for the one-pot preparation of a series of β-amino esters from both enolizable and non-enolizable aldimines-borane complexes. Aromatic nitriles (1a-d) were converted to the corresponding aldimine-triethylborane complexes (2a-d) via reduction with Super-Hydride™, followed by methanolysis. Since the reduction of aliphatic nitriles with Super-Hydride™ is not efficient,19 an alternate procedure involving the reduction of these nitriles (1e-f) with DIBAL-H, followed by methanolysis and addition of triethylborane was employed to prepare the aldimine complexes 2e-f.21 Condensation of these enolizable and non-enolizable aldimine-triethylborane complexes with trimethylsilyl ketene acetal in presence of 0.5 equiv. of ZnI2 after replacing the solvent (THF for 2a-d and hexanes for 2e-f) with ether provided the β-amino ester was (3a-e) within 2 h at room temperature in 59-75% isolated overall yields from the nitriles (Schemes 4 and 5). The results are summarized in Table 2.
Having achieved the successful preparation of the β-amino esters, we extended this protocol for a one-pot preparation of the corresponding β-lactams. As described earlier, THF was replaced with Et2O after the removal of LiOMe from the in-situ generated benzaldimine-triethylborane complex (2a), followed by the addition of 0.5 equivalent of ZnI2 and the ketene acetal. The reaction mixture was now treated with methylmagnesium bromide to achieve lactamization. Refluxing the reaction mixture for 48 h, followed by workup with saturated ammonium chloride solution afforded the desired 3,3-dimethyl-4-phenylazetidin-2-one (4a) in 69% yield. The reaction was then extended to the same series of representative enolizable and non-enolizable aldimine-triethylborane complexes (2b-f) obtained from the corresponding nitriles (1b-f) and the corresponding α,α-dimethyl β-lactams (4b-f) were obtained in 58-74% yield (Scheme 6, Table 3).
In conclusion, we have developed an efficient one-pot synthesis of β-amino esters from aromatic and aliphatic nitriles via the condensation of non-enolizable and enolizable aldimine-triethylborane complexes with methyl trimethylsilyl dimethylketene acetal in the presence of 0.5 equiv. of ZnI2. The cyclization of the intermediate β-amino esters to the corresponding β-lactams were achieved in the same pot via a methyl Grignard mediated lactamization. We are currently investigating the diastereoselective and enantioselective approaches of this synthesis. The results on this study will be reported subsequently.
EXPERIMENTAL
General: Unless otherwise noted, reagents were purchased from Aldrich Chemical Co. Tetrahydrofuran (THF) was distilled from sodium and sodium benzophenone ketyl under nitrogen. Thin layer chromatography was performed on Sorbent Technologies precoated silica gel w/UV254 glass backed (250 μm) plates. Silica gel (Sorbent Technologies 230-400 mesh) was used for flash column chromatography. 1H NMR spectra was recorded on a Gemini (300 MHz) or Bruker (200 MHz) spectrometer. Spectra was referenced internally to the residual proton resonance in CDCl3 (δ 7.26 ppm) as the internal standard. Commercially available CDCl3 was stored under 4 Ǻ molecular sieves. Chemical shifts (δ) were reported as parts per million (ppm) in δ scale downfield from TMS. 13C NMR spectra were recorded on a Gemini 300 spectrometer and referenced to CDCl3 (δ 77.0 ppm). Coupling constants (J) were reported in Hertz. Spectroscopic data is presented for analytical samples of all compounds.
General procedure for the preparation of methyl 3-amino-2,2-dimethyl-3-phenylpropanoate (3a): Lithium triethylborohydride (1M in THF; 5.0 mL, 5.0 mmol) was added to a solution of benzonitrile (1a; 0.52 mL, 5.05 mmol) in THF (5 mL) at 0 °C and the solution was stirred for 2 h. MeOH (0.20 mL, 5.0 mmol) was then added to the reaction mixture and stirred for another 30 min. LiOMe was filtered off and the solvent was evaporated under reduced pressure and replaced with Et2O (4 mL). ZnI2 (0.85 g, 2.53 mmol) was added and stirred for 30 min, followed by the slow addition of methyl trimethylsilyldimethylketene acetal (1.02 mL, 5.05 mmol) in Et2O (2 mL). The solution was stirred for 2 h at room temperature and poured into saturated aqueous NH4Cl (15 mL) and shaken vigorously. The product was then extracted with Et2O (3x20 mL), the combined organic layer was washed with saturated aqueous NH4Cl solution and dried over anhydrous Na2SO4 The solvent was evaporated under reduced pressure, and the crude product was purified over silica gel (hexanes:EtOAc 85:15) to afford 0.74 g (3.59 mmol, 71% yield) of 3a.13 1H NMR (300 MHz, CDCl3): δ (ppm) 7.33-7.26 (m, 5H), 4.26 (s, 1H), 3.72 (m, 3H), 1.71 (br s, 2H), 1.18 (s, 3H), 1.12 (s, 3H); 13C NMR (75 MHz, CDCl3): δ (ppm) 177.8, 141.9, 128.1, 127.8, 127.4, 61.9, 51.8, 47.8, 23.6, 19.4; MS (EI): 208 [M+H]+, 191 [M+H-NH3]+, 176, 106 [Ph-CH-NH2]+; (CI): 208 [M+H]+, 106 [M+H-NH3]+.
Methyl 3-amino-2,2-dimethyl-3-p-tolylpropanoate (3b):22 Yield: 64%. 1H NMR (300 MHz, CDCl3): δ 7.22-7.15 (m, 4H), 4.23 (s, 1H), 3.74 (m, 3H), 2.37 (s, 3H), 1.89 (br s, 2H), 1.17 (s, 3H), 1.12 (s, 3H); 13C NMR (75 MHz, CDCl3): δ 177.7, 137.2, 129.8, 128.7, 128.1, 61.7, 51.9, 47.6, 23.7, 21.1, 19.7; MS (EI): 222 [M+H]+, 205 [M+H-NH3]+, 190 [M+H-HOCH3], 120; (CI): 222 [M+H]+, 205.
Methyl 3-amino-3-(4-methoxyphenyl)-2,2-dimethylpropanoate (3c):23 Yield: 69%. 1H NMR (300 MHz, CDCl3): δ 7.25-7.18 (m, 4H), 4.23 (s, 1H), 3.91 (m, 3H), 3.70 (s, 3H), 1.99 (br s, 2H), 1.19 (s, 3H), 1.10 (s, 3H); 13C NMR (75 MHz, CDCl3): δ 177.7, 141.5, 128.1, 127.7, 126.1, 63.0, 56.7, 51.9, 47.7, 23.6, 19.6; MS (EI): 238 [M+H]+, 221 [M+H-NH3]+, 136; (CI): 238 [M+H]+, 221.
Methyl 3-amino-2,2-dimethyl-3-(thiophen-2-yl)propanoate (3d): Yield: 75%. 1H NMR (300 MHz, CDCl3): δ 7.16 (d, J = 5.4 Hz, 1H), 6.94-6.87 (m, 2H), 4.51 (s, 1H), 3.68 (m, 3H), 1.94 (br s, 2H), 1.21 (s, 3H), 1.13 (s, 3H); 13C NMR (75 MHz, CDCl3): δ 177.4, 146.0, 126.3, 125.1, 124.1, 58.2, 51.9, 47.7, 23.0, 20.2; MS (EI): 214 [M+H]+, 197 [M+H-NH3]+, 182 [M+H-HOCH3]; (CI): 214 [M+H]+, 197.
General procedure for the preparation of methyl 3-amino-3-cyclohexyl-2,2-dimethylpropanoate (3e): DIBAL-H (0.91 mL, 5.05 mmol) was added to a solution of cyclohexanecarbonitrile (1e; 0.60 mL, 5.05 mmol) in hexanes (3 mL) at -78 oC and the solution was stirred for 3 h. Triethylborane (1M in hexanes; 5.05 mL, 5.05 mmol) was then added, followed by the addition of MeOH (0.20 mL, 5.0 mmol) and the reaction mixture was stirred for 30 min while warming to RT. The solvent was evaporated under reduced pressure and replaced by Et2O (4 mL). ZnI2 (0.85 g, 2.53 mmol) was added and stirred for 30 min, followed by the slow addition of methyl trimethylsilyldimethylketene acetal (1.02 mL, 5.05 mmol) in Et2O (2 mL). The solution was stirred for 2 h at RT, poured into saturated aqueous NH4Cl (15 mL), and shaken vigorously. The product was then extracted with Et2O (3x20 mL), the combined organic layer was washed with saturated aqueous NH4Cl solution and dried over anhydrous Na2SO4. The solvent was evaporated under reduced pressure, and the crude product was purified over silica gel (hexanes:EtOAc 85:15) to afford 0.66 g (3.08 mmol, 61% yield) of 3e.22 1H NMR (300 MHz, CDCl3): δ (ppm) 3.69 (s, 3H), 3.19 (dd, J = 8.5 Hz, J = 3.1 Hz, 1H), 1.77-158 (m, 5H), 1.51-1.01 (m, 9H), .081 (s, 3H); 13C NMR (75 MHz, CDCl3): δ (ppm) 179.1, 62.3, 51.8, 47.8, 31.1, 27.6, 26.9, 24.1 22.6, 19.4; MS (EI): 213 [M]+, 187 [M+H-NH3]+, 99; (CI): 214 [M+H]+, 187.
Methyl 3-amino-2,2-dimethylheptanoate (3f): Yield: 59%. 1H NMR (300 MHz, CDCl3): δ 3.54 (s, 3H), 2.72 (dd, J = 10.5 Hz, J = 2.4 Hz, 1H), 1.48 (br s, 2H), 1.32-1.07 (m, 5H), 1.04 (s, 3H), 1.02 (s, 3H), 0.79 (t, J = 6.3 Hz, 4H); 13C NMR (75 MHz, CDCl3): δ 177.9, 57.8, 51.5, 47.4, 32.1, 29.4, 22.5, 21.1, 20.8, 13.9; MS (EI): 188 [M+H]+, 177 [M+H-NH3]+, 86; (CI): 188 [M+H]+, 156.
General Procedure for the preparation of 3,3-dimethyl-4-phenylazetidin-2-one (4a): Lithium triethylborohydride (1M in THF; 5.0 mL, 5.0 mmol) was added to a solution of benzonitrile (1a; 0.52 mL, 5.05 mmol) in THF (5 ml) at 0 oC and stirred for 2 h. MeOH (0.20 mL, 5.0 mmol) was then added to the reaction mixture and stirred for another 30 min. LiOMe was filtered, the solvent was evaporated under reduced pressure and replaced with Et2O (4 mL). ZnI2 (0.85 g, 2.53 mmol) was then added and stirred for 30 min, followed by the slow addition of methyl trimethylsilyldimethylketene acetal (1.02 mL, 5.05 mmol) in Et2O (2 mL). The solution was stirred for 2 h at RT, followed by the addition of methylmagnesium bromide (3M in Et2O, 2.02 mL, 6.06 mmol). The reaction mixture was then refluxed for 48 h and poured into saturated aqueous NH4Cl (15 mL) and shaken vigorously. The product was then extracted with Et2O (3x20 mL), the combined organic layer was washed with saturated aqueous NH4Cl solution and dried over anhydrous Na2SO4. The solvent was evaporated under reduced pressure, and the crude product was purified over silica gel (hexanes:EtOAc 80:20) to afford 0.69 g (3.48 mmol, 69% yield) of 4a.13 1H NMR (300 MHz, CDCl3): δ (ppm) 7.44-7.28 (m, 5H), 6.74 (br s, 2H), 4.55 (s, 1H), 1.50 (s, 3H), 0.81 (s, 3H); 13C NMR (75 MHz, CDCl3): δ (ppm) 175.4, 137.9, 128.6, 127.8, 126.1, 63.2, 56.9, 22.6, 18.3; MS (EI): 175 [M]+, 106 [Ph-CH-NH2]+; (CI): 176 [M+H]+, 117.
3,3-Dimethyl-4-p-tolylazetidin-2-one (4b): Yield: 74%. 1H NMR (300 MHz, CDCl3): δ 7.21-7.13 (m, 4H), 7.02 (br s, 2H), 4.48 (s, 1H), 2.37 (s, 3H), 1.47 (s, 3H), 0.79 (s, 3H); 13C NMR: (75 MHz, CDCl3): δ 175.6, 137.4, 134.9, 129.2, 126.1, 63.1, 56.6, 22.5, 21.1, 18.2; MS (EI): 190 [M+H]+, 146, 120 [CH3-Ph-CH-NH2]+; (CI): 189 [M]+, 120.
4-(4-Methoxyphenyl)-3,3-dimethylazetidin-2-one (4c):24 Yield: 73%. 1H NMR (300 MHz, CDCl3): δ 7.15 (d, J = 8.1 Hz, 2H), 7.05 (br s, 2H), 6.89 (d, J = 8.7 Hz, 2H), 4.42 (s, 1H), 3.78 (s, 3H), 1.42 (s, 3H), 0.75 (s, 3H); 13C NMR (75 MHz, CDCl3): δ 175.6, 159.1, 129.9, 127.2, 113.9, 62.8, 56.5, 55.3, 55.1, 22.5, 18.1; MS (EI): 205 [M]+, 136, 162; (CI): 206 [M+H]+, 234.
3,3-Dimethyl-4-(thiophen-2-yl)azetidin-2-one (4d):25 Yield: 64%. 1H NMR (300 MHz, CDCl3): δ 7.31 (d, J = 5.9 Hz, 1H), 7.09-6.98 (m, 2H), 6.71 (br s, 2H), 4.74 (s, 1H), 3.78 (s, 3H), 1.46 (s, 3H), 0.98 (s, 3H); 13C NMR: (75 MHz, CDCl3): δ174.9, 142.0, 127.3, 124.8, 124.6, 59.6, 57.7, 22.2, 18.1; MS (EI): 182 [M+H]+, 112 [Thp-CH-NH2]+; (CI): 182 [M+H]+, 106.
General procedure for the preparation of 4-cyclohexyl-3,3-dimethylazetidin-2-one (4e): DIBAL-H (0.91 mL, 5.05 mmol) was added to a solution of cyclohexanecarbonitrile (1e; 0.60 mL, 5.05 mmol) in hexanes (3 mL) at -78 oC and the solution was stirred for 3 h. Triethylborane (1M in hexanes; 5.05 mL, 5.05 mmol) was then added, followed by the addition of MeOH (0.20 mL, 5.0 mmol) and the reaction mixture was stirred for 30 min while warming to RT. The solvent was evaporated under reduced pressure and replaced with Et2O (4 mL). ZnI2 (0.85 g, 2.53 mmol) was then added and stirred for 30 min, followed by the slow addition of methyl trimethylsilyldimethylketene acetal (1.02 mL, 5.05 mmol) in Et2O (2 mL). The solution was stirred for 2 h at RT, followed by the addition of methylmagnesium bromide (3M in Et2O, 2.02 mL, 6.06 mmol). The reaction mixture was refluxed for 48 h and poured into saturated aqueous NH4Cl (15 mL) and shaken vigorously. The product was then extracted with Et2O (3x20 mL), the combined organic layer was washed with saturated aqueous NH4Cl solution and dried over anhydrous Na2SO4. The solvent was evaporated under reduced pressure, and the crude product was purified over silica gel (hexanes:EtOAc 80:20) to afford 0.55 g (3.03 mmol, 60% yield) of 4e.24 1H NMR (300 MHz, CDCl3): δ (ppm) 6.19 (br s, 2H), 3.0 (d, J = 7.4 Hz, 1H), 1.79-1.60 (m, 5H), 1.47-1.15 (m, 9H), 1.09-0.94 (m, 3H); 13C NMR (75 MHz, CDCl3): δ (ppm) 180.4, 65.7, 38.8, 30.7, 29.7, 26.2, 25.6, 23.0, 17.1; MS (EI): 182 [M+H]+, 112 [Chx-CH-NH2]+; (CI): 181 [M]+, 112.
4-Butyl-3,3-dimethylazetidin-2-one (4f):26 Yield: 58%. 1H NMR (300 MHz, CDCl3): δ 7.01 (br s, 2H), 3.20 (dd, J = 8.4 Hz, J = 3.0 Hz, 1H), 1.29-1.18 (m, 10H), 1.09 (s, 3H), 0.85 (t, J = 6.3 Hz, 3H); 13C NMR (75 MHz, CDCl3): δ 175.6, 60.6, 53.0, 30.8, 29.6, 28.8, 22.5, 16.6, 13.7; MS (EI): 156 [M+H]+, 86 [CH3(CH2)3-CH-NH2]+; (CI): 155 [M]+, 70.
ACKNOWLEDGEMENTS
We thank the Herbert C. Brown Center for Borane Research for support of this work.
References
1. H. Staudinger, Liebigs Ann. Chem., 1907, 356, 51. CrossRef
2. H. T. Clark, J. R. Jonson, and R. Robindon, The Chemistry of Penicillins, Princeton University Press, 1949.
3. Chemistry and Biology of β-Lactam Antibiotics, R. B. Morin and M. Goldman, Eds., Academic Press, New York, 1982, Vol. 1-3; Recent Advances in the Chemistry of Anti-Infective Agents, P. H. Bentley and R. Ponsford, Eds., The Royal Society of Chemistry, Cambridge, 1993.
4. I. Ojima, Acc. Chem. Res., 1995, 28, 383; CrossRef B. Alcaide, P. Almendros, and C. Aragoncillo, Chem. Rev., 2007, 107, 4437. CrossRef
5. The Chemistry of β-Lactams, M. I. Page, Ed., Chapman and Hall, London, 1992; The Organic Chemistry of β-Lactams, G. I. George, Ed., VCH: New York, 1993; M. Kidwai, P. Sapra, and K. R. Bhushan, Curr. Med. Chem., 1999, 6, 195.
6. S. France, A. E. Weatherwax, A. Taggi, and T. Lectka, Acc. Chem. Res., 2004, 37, 592; CrossRef G. S. Singh, Tetrahedron, 2003, 59, 7631. CrossRef
7. E. Juaristi, J. D. Quintana, B. Lamatsch, and D. Seebach, J. Org. Chem., 1991, 56, 2553; CrossRef E. Juaristi, D. Quintana, M. Balderas, and E. Garcia-Perez, Tetrahedron: Asymmetry, 1996, 7, 2233. CrossRef
8. For a historic perspective see: T. T. Tidwell, Angew. Chem. Int. Ed., 2008, 47, 1016. CrossRef
9. G. Cainelli, P. Galletti, and D. Giacomoni, Tetrahedron Lett., 1998, 39, 7779; CrossRef C. Palamo, J. M. Aizpurua, M. Iturburu, and R. Urchegui, J. Org. Chem., 1994, 59, 240; CrossRef C. Palamo, J. M. Aizpurua, R. Urchegui, and M. Iturburu, J. Org. Chem., 1992, 57, 1571; CrossRef D. M. Tschaen, L. M. Fuentes, J. E. Lynch, W. L. Laswell, R. P. Volante, and I. Shinkai, Tetrahedron Lett., 1988, 29, 2779. CrossRef
10. C. Palomo, J. M. Aizpurua, I. Ganboa, and M. Oiarbide, Curr. Med. Chem., 2004, 11, 1837.
11. L. Birkofer and J. Schramm, Liebigs Ann. Chem., 1977, 760.
12. D.-C. Ha, D. J. Hart, and T.-K. Yang, J. Am. Chem. Soc., 1984, 106, 4819. CrossRef
13. E. W. Colvin, D. McGarry, and M. J. Nugent, Tetrahedron, 1988, 44, 4157; CrossRef E. W. Colvin and D. G. McGarry, J. Chem. Soc., Chem. Commun., 1985, 539. CrossRef
14. K. Ishihara, N. Hanaki, M. Funahashi, M. Miyata, and H.Yamamoto, Bull. Chem. Soc. Jpn., 1995, 68, 1721. CrossRef
15. M. Suginome, L. Uehlin, and M. Murakami, J. Am. Chem. Soc., 2004, 126, 13196. CrossRef
16. K. Kanai, H. Wakabayashi, and T. Honda, Heterocycles, 2002, 58, 47. CrossRef
17. T. Honda, H. Wakabayashi, and K. Kanai, Chem. Pharm. Bull., 2002, 50, 307. CrossRef
18. A. Tarui, K. Kondo, H. Taira, K. Sato, M. Omote, I. Kumadaki, and A. Ando, Heterocycles, 2007, 73, 203; CrossRef A. Tarui, D. Ozaki, N. Nakajima, Y. Yokota, Y. S. Sokeirik, K. Sato, M. Omote, I. Kumadaki, and A. Ando, Tetrahedron Lett., 2008, 49, 3843. CrossRef
19. P. V. Ramachandran and D. Biswas, Org. Lett., 2007, 9, 3025. CrossRef
20. P. V. Ramachandran, G. V. Reddy, and D. Biswas, J. Flour. Chem., 2009, 130, 204.
21. G. M. Chen and H. C. Brown, J. Am. Chem. Soc., 2000, 122, 4217. CrossRef
22. S. Kobayashi and Y. Aoki, Tetrahedron Lett., 1998, 39, 7345. CrossRef
23. R. Kawecki, Russ. J. Org. Chem., 1999, 64, 8724.
24. H. Nagai, T. Shiozawa, K. Achiwa, and T. Kazuo, Chem. Pharm. Bull., 1993, 41, 1933.
25. P. Andreoli, G. Cainelli, M. Contento, D. Giacomini, G. Marelli, and M. Paununzio, J. Chem. Soc., Perkin Trans. 1, 1988, 945. CrossRef
26. T. Uyehara, I. Suzuki, and Y. Yamamoto, Tetrahedron Lett., 1989, 30, 4275. CrossRef