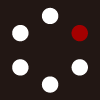
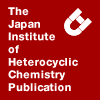
HETEROCYCLES
An International Journal for Reviews and Communications in Heterocyclic ChemistryWeb Edition ISSN: 1881-0942
Published online by The Japan Institute of Heterocyclic Chemistry
e-Journal
Full Text HTML
Received, 20th August, 2010, Accepted, 3rd September, 2010, Published online, 6th September, 2010.
DOI: 10.3987/COM-10-S(E)109
■ Convenient Synthesis of the Key Intermediate for Dihydrocorynantheol and Protoemetinol from the Monoacetate of 4-Cyclopentene-1,3-diol
Yuichi Kobayashi,* Kaori Yagi, and Yuki Kaneko
Graduate School of Bioscience and Biotechnology, Tokyo Institute of Technology, 4259 Nagatsuta-cho, Midori-ku, Yokohama 226-8501, Japan
Abstract
We invented an efficient method to obtain the key δ-lactone possessing the (CH2)2OTBDPS and Et groups at C3 and C4, respectively, starting with the acetate of 4-cyclopentene-1,3-diol, which was subjected to Pd-catalyzed allylation with malonate anion to attach the (CH2)2OTBDPS group. The Et group was then installed by 1,4-addition to the derived enone. Finally, the resulting enol TMS ether was oxidized to afford the lactone. Furthermore, the lactone was converted to dihydrocorynantheol and protoemetinol, both of which are typical examples of indoloquinolizidine and benzo[a]quinolizine alkaloids.INTRODUCTION
Dihydrocorynantheol and protoemetinol are typical alkaloids possessing the indoloquinolizidine and benzo[a]quinolizine structures, respectively (Figure 1).1 In addition to the interesting biological activities such as antiparasitic, antiviral, and analgesic activities, the structures have attracted much attention as synthetic targets, and thus various synthetic methods have been developed. Among them, δ-lactone 1a (R = Bn) introduced by Ihara and Fukumoto2 as the key intermediate for synthesis of these alkaloids attracted our attention because of its synthetic potency. The lactone 1a was constructed by using intramolecular radical cyclization of the bromo cis-olefin. Although the cyclization is stereoselective, the preparation of the cis-olefin suffers from somewhat low stereoselectivity. As an alternative synthesis of lactone 1 we envisioned a synthetic method starting with the monoacetate 2 through stereoselective installation of the two side chains and subsequent
lactone ring formation as illustrated in Scheme 1. For installation of the (CH2)2OR group, a reaction taking place with retention of the configuration requires the (S)-acetate as shown in Scheme 1, while that with inversion does the (R)-enantiomer. Since both of the enantiomers can be available easily by using the enzyme-assisted asymmetric hydrolysis of the corresponding diacetate,3,4 both approaches are feasible. Consequently, we investigated the synthesis using palladium-catalyzed allylation with malonate anion, which proceeds with retention of the configuration. Herein, we report preliminary results along this line using racemic monoacetate 2, which furnished lactone 1b (R = TBDPS). Furthermore, 1b was transformed to dihydrocorynantheol2a,b,5 and protoemetinol2c,6 with modification.
RESULTS AND DISCUSSION
Palladium-catalyzed reaction of the racemic monoacetate 2 with malonate in the presence of t-BuOK at room temperature proceeded regioselectively with retention of stereochemsitry.7 Next, decarboxylation of the product under the standard conditions afforded 4 in 96% yield (Scheme 2). Hydride reduction produced the corresponding diol, and the primary hydroxyl group was protected with TBDPSCl under carefully controlled conditions to prevent participation of the secondary hydroxyl group in the protection reaction (slow addition of TBDPSCl in DMF, –30 °C). Jones oxidation of the remaining hydroxyl group in the mono-TBDPS ether 5 afforded enone 6 in 91% yield. To install the Et group, EtMgBr and CuI was mixed to prepare Et2CuMgBr, which was subjected to conjugate addition to enone 6 in the presence of DMPU and Et3N at –78 to –40 °C. The enolate thus generated was quenched with TMSCl to afford TMS ether 7. Without purification by chromatography, enol ether 7 was subjected to ozonolysis and the resulting peroxide was reduced with NaBH4 in one-pot. Finally, the product was treated with 1 N HCl at room temperature to afford lactone 1b in high yield. Total yield was 60% from the monoacetate 2.
Transformation of 1b to dihydrocorynatheol is summarized in Scheme 3, in which reactions being different from the original method2a,b are involved. First, a mixture of 1b and tryptamine (8) in xylene was heated under reflux to give amide alcohol 9 in 94% yield, which was converted to mesylate 10. For construction of the lactam ring, KN(TMS)2 in THF was selected due to operational convenience (cf. KH with 18-crown-6 by Ihara and Fukumoto2a,b). The reaction proceeded at room temperature smoothly to afford lactam 3A in 88% yield, which was treated with POCl3 at 110 °C to produce iminium salt 11. During the conversion, the TBDPS group was removed. Finally, reduction of 11 with NaBH42a,b,5h furnished dihydrocorynantheol stereoselectively in 93% yield from the lactam 3A. The 1H NMR spectrum of the product was identical with that reported.5b,e–g
In a similar manner, reaction of lactone 1b with homoveratrylamine (12) in xylene under reflux afforded hydroxyamide 13, which upon reaction with MsCl followed by cyclization with KN(TMS)2 produced lactam 14 quantitatively as shown in Scheme 4. Finally, reaction with POCl3 followed by hydride reduction of the iminium salt 15 with NaBH48 produced protoemetinol stereoselectively. The product was identified by 1H NMR spectroscopy.6a
CONCLUSION
The key lactone 1b was synthesized from the easily available monoacetate 2 by using the stereoselective installation of the two side chains (Scheme 2), and transformed to dihydrocorynantheol (Scheme 3) and protoemetinol (Scheme 4) with modification of reaction conditions. Since the present method has no restriction on using oxygenated indol and aryl groups, the method would be applicable to a wide variety of alkaloids possessing the indoloquinolizidine and benzo[a]quinolizine structures.
EXPERIMENTAL
General. Infrared (IR) spectra are reported in wave numbers (cm–1). The 1H NMR (300 and 500 MHz) and 13C NMR (75 MHz) spectra were measured in CDCl3 using SiMe4 (δ = 0 ppm) and the center line of CDCl3 triplet (δ = 77.1 ppm) as internal standards, respectively. The following solvents were distilled before use: THF (from Na/benzophenone), Et2O (from Na/benzophenone), and CH2Cl2 (from CaH2).
(4S*,5R*)-4-(2-((tert-Butyldiphenylsilyl)oxy)ethyl)-5-ethyltetrahydro-2H-pyran-2-one (1b). To a slurry of CuI (302 mg, 1.59 mmol) in THF (0.6 mL) was added EtMgBr (3.90 mL, 0.75 M in THF, 2.93 mmol) slowly at –78 °C. After 1 h of stirring at –30 °C, a solution of cyclopentenone 6 (463 mg, 1.32 mmol), DMPU (0.33 mL, 2.73 mmol), and Et3N (0.33 mL, 2.37 mmol) in THF (6 mL) was added dropwise at –78 °C. The mixture was stirred at –78 °C for 45 min and then at –40 °C for 5 min. TMSCl (0.50 mL, 3.96 mmol) was added at –40 °C. The mixture was gradually warmed to 0 °C over 1 h, and diluted with saturated NaHCO3. The resulting mixture was extracted with hexane five times. The combined extracts were dried over Na2SO4 and concentrated to leave the enol ether 7. A steam of O3 in O2 was gently bubbled into a solution of the enol ether 7 in MeOH (6.6 mL) and CH2Cl2 (0.66 mL) at –78 °C for 5 min. Excess O3 remaining in the solution was purged by bubbling argon at –78 °C. The solution was warmed to 0 °C and NaBH4 (250 mg, 6.61 mmol) was added. The solution was stirred at 0 °C for 1 h and diluted with EtOAc and 1 N HCl (6 mL). The mixture was stirred at room temperature for 2 h and extracted with EtOAc three times. The combined extracts were dried over MgSO4 and concentrated to leave a residue, which was purified by chromatography on silica gel (hexane/EtOAc) to provide lactone 1b (522 mg, 96%): 1H NMR (300 MHz, CDCl3) δ 0.93 (t, J = 7.5 Hz, 3 H), 1.05 (s, 9 H), 1.20–1.56 (m, 4 H), 1.68–1.82 (m, 1 H), 1.89–2.03 (m, 1 H), 2.19 (dd, J = 16, 7.5 Hz, 1 H), 2.56 (dd, J = 16, 6.5 Hz, 1 H), 3.60–3.76 (m, 2 H), 3.99 (dd, J = 11, 7 Hz, 1 H), 4.27 (dd, J = 11, 4 Hz, 1 H), 7.34–7.46 (m, 6H), 7.61–7.67 (m, 4 H); 13C NMR (75 MHz, CDCl3) δ 11.2, 19.2, 24.2, 26.9, 32.5, 34.0, 38.0, 39.9, 60.8, 70.4, 127.7, 127.8, 129.77, 129.80, 133.5, 135.5, 172.7. HRMS (FAB) calcd for C25H35O3Si [(M+H)+] 411.2355, found 411.2558.
(3S*,4R*)-N-(2-(1H-Indol-3-yl)ethyl)-3-(2-((tert-butyldiphenylsilyl)oxy)ethyl)-4-(hydroxymethyl)hexanamide (9). A mixture of lactone 1b (312 mg, 0.760 mmol) and tryptamine 8 (304 mg, 1.90 mmol) in p-xylene (3.5 mL) was heated under reflux overnight. Most of the volatile materials were removed under reduced pressure to afford an oil, which was purified by chromatography on silica gel (CH2Cl2/MeOH) to furnish 9 (408 mg, 94%): 1H NMR (300 MHz, CDCl3) δ 0.88 (t, J = 7 Hz, 3 H), 1.05 (s, 9 H), 1.15–1.49 (m, 5 H), 1.60–1.79 (m, 2 H), 1.94–2.21 (m, 2 H), 2.71 (br s, 1 H), 2.91 (t, J = 7 Hz, 2 H), 3.35–3.74 (m, 6 H), 5.56 (t, J = 5.5 Hz, 1 H), 6.94 (d, J = 2 Hz, 1 H), 7.11 (t, J = 7 Hz, 1 H), 7.19 (t, J = 7.5 Hz, 1 H), 7.23–7.50 (m, 7 H), 7.50–7.72 (m, 5 H), 7.98 (br s, 1 H).
(4S*,5R*)-1-(2-(1H-Indol-3-yl)ethyl)-4-(2-((tert-butyldiphenylsilyl)oxy)ethyl)-5-ethylpiperidin-2-one (3A). To an ice-cold solution of alcohol 9 (32 mg, 0.056 mmol) and Et3N (0.020 mL, 0.14 mmol) in CH2Cl2 (0.6 mL) was added MsCl (0.009 mL, 0.12 mmol). After being stirred at room temperature for 1 h, the solution was diluted with CH2Cl2 and washed successively with saturated NaHCO3 and H2O. The organic layer was dried over Na2SO4 and concentrated to give mesylate 10, which was subjected to the next reaction without further purification. To an ice-cold solution of the above mesylate 10 in THF (0.6 mL) was added KN(TMS)2 (0.13 mL, 0.50 M in toluene, 0.065 mmol). After being stirred at room temperature for 1 h, the reaction was quenched by addition of saturated NH4Cl. The resulting mixture was extracted with EtOAc three times. The combined extracts were dried over Na2SO4 and concentrated to leave a residue, which was purified by chromatography on silica gel (CH2Cl2/MeOH) to provide 3A (27 mg, 88%): 1H NMR (300 MHz, CDCl3) δ 0.74 (t, J = 7.5 Hz, 3 H), 1.04 (s, 9 H), 1.05–1.53 (m, 4 H), 1.63–1.84 (m, 2 H), 2.00 (dd, J = 17, 8 Hz, 1 H), 2.47 (dd, J = 17, 5.5 Hz, 1 H), 2.85 (dd, J = 13, 7 Hz, 1 H), 3.02 (t, J = 7.5 Hz, 2 H), 3.12 (dd, J = 13, 5 Hz, 1 H), 3.50–3.75 (m, 4 H), 7.03 (d, J = 2 Hz, 1 H), 7.11 (t, J = 7.5 Hz, 1 H), 7.17 (t, J = 7 Hz, 1 H), 7.29–7.47 (m, 7 H), 7.58–7.69 (m, 5 H), 7.96 (br s, 1 H); 13C NMR (75 MHz, CDCl3) δ 11.0, 19.3, 23.2, 23.8, 27.0, 33.0, 36.1, 36.5, 39.3, 48.1, 51.4, 61.3, 111.2, 113.5, 118.9, 119.5, 122.0, 122.1, 127.77, 127.79, 129.75, 129.78, 133.9, 135.6, 169.6.
(–)-Dihydrocorynanteol. A mixture of lactam 3A (20 mg, 0.036 mmol) and POCl3 (0.2 mL) was refluxed for 3 h. Most of the volatile materials were removed, and the resulting residue was dried up by azeotropic distillation with THF three times for the next reaction. To an ice-cold solution of the crude iminium salt 11 in MeOH (0.4 mL) was added NaBH4 (7 mg, 0.18 mmol). The solution was stirred at 0 °C for 1 h and diluted with 1 N NaOH, brine, and EtOAc. The layers were separated, and the aqueous layer was extracted with CH2Cl2 three times. The combined extracts were dried over K2CO3 and concentrated to leave a residue, which was purified by chromatography (CH2Cl2/MeOH) to afford (–)-dihydorocorynanteol (10 mg, 93%): 1H NMR (300 MHz, CDCl3) δ 0.94 (t, J = 7.5 Hz, 3 H), 1.1–1.8 (m, 8 H), 2.08–2.24 (m, 2 H), 2.55–2.80 (m, 2 H), 2.93–3.17 (m, 3H), 3.23 (d, J = 11 Hz, 1 H), 3.54–3.76 (m, 2 H), 7.04–7.19 (m, 2 H), 7.33 (d, J = 7.5 Hz, 1 H), 7.47 (d, J = 7.5 Hz, 1 H), 7.88 (br s, 1 H). The spectrum was identical with the data reported for (–)-dihydorocorynanteol in the literature.5b,e–g
(3S*,4R*)-3-(2-((tert-Butyldiphenylsilyl)oxy)ethyl)-N-(3,4-dimethoxyphenethyl)-4-(hydroxymethyl)hexanamide (13). A mixture of the lactone 1b (165 mg, 0.402 mmol) and 3,4-dimethoxyphenyethylamine 12 (0.47 mL, 2.82 mmol) was heated under reflux overnight. Most of the volatile materials were removed under reduced pressure to afford an oil, which was purified by chromatography on silica gel (CH2Cl2/MeOH) to furnish 13 (232 mg, 98%): 1H NMR (300 MHz, CDCl3) δ 0.89 (t, J = 7.5 Hz, 3 H), 1.05 (s, 9 H), 1.14–1.80 (m, 6 H), 2.15 (s, 2 H), 2.55 (br s, 1 H), 2.69 (t, J = 7 Hz, 2 H), 3.35–3.75 (m, 6 H), 3.84 (s, 3 H), 3.85 (s, 3 H), 5.56–5.66 (m, 1 H), 6.65–6.72 (m, 2 H), 6.77 (d, J = 9 Hz, 1 H), 7.33–7.46 (m, 6 H), 7.62–7.68 (m, 4 H).
(4S*,5R*)-4-(2-((tert-Butyldiphenylsilyl)oxy)ethyl)-1-(3,4-dimethoxyphenethyl)-5-ethylpiperidin-2-one (14). To an ice-cold solution of alcohol 13 (30 mg, 0.0507 mmol) and Et3N (0.036 mL, 0.256 mmol) in THF (0.5 mL) was added MsCl (0.020 mL, 0.26 mmol). The solution was stirred at room temperature for 10 min and cooled to 0 °C. To this solutions was added KN(TMS)2 (0.72 mL, 0.70 M in toluene, 0.504 mmol). The solution was stirred at room temperature overnight and diluted with brine. The resulting mixture was extracted with CH2Cl2 three times. The combined extracts were dried over Na2SO4 and concentrated to leave a residue, which was purified by chromatography on silica gel (CH2Cl2/MeOH) to provide 14 (28 mg, 99%): 1H NMR (300 MHz, CDCl3) δ 0.81 (t, J = 7.5 Hz, 3 H), 1.04 (s, 9 H), 1.1–1.9 (m, 6 H), 1.99 (dd, J = 17, 8 Hz, 1H), 2.47 (dd, J = 17, 5 Hz, 1 H), 2.80 (t, J = 7.5 Hz, 2 H), 2.75–2.90 (m, 1 H), 3.12 (dd, J = 12, 5 Hz, 1 H), 3.34–3.76 (m, 4 H), 3.84 (s, 3 H), 3.86 (s, 3 H), 6.71–6.81 (m, 3 H), 7.34–7.45 (m, 6 H), 7.64 (ddm, J = 7.5, 1.5 Hz, 4 H).
(–)-Protoemetiol. A mixture of lactam 14 (17 mg, 0.0296 mmol) and POCl3 (0.14 mL) was refluxed at 110 °C for 3 h. Most of the volatile materials were removed and the resulting residue was dried up by azeotropic distillation with THF three times to give the iminium salt 15, which was used for the next reaction without further purification. To an ice-cold solution of the above salt 15 in MeOH (0.3 mL) was added NaBH4 (6 mg, 0.16 mmol). The solution was stirred at 0 °C for 1 h, and diluted with 1 N NaOH, brine, and EtOAc. The layers were separated and the aqueous layer was extracted with CH2Cl2 three times. The combined extracts were dried (K2CO3) and concentrated. The residue was purified by chromatography on silica gel (CH2Cl2/MeOH) to afford (–)-protoemetinol (9 mg, 95%): 1H NMR (300 MHz, CDCl3) δ 0.93 (t, J = 7.5 Hz, 3 H), 1.06–1.31 (m, 3 H), 1.35–1.77 (m, 5 H), 2.05 (t, J = 11 Hz, 1 H), 1.95–2.23 (m, 1 H), 2.32 (dt, J = 13, 3 Hz, 1 H), 2.48 (dt, J = 4, 11 Hz, 1 H), 2.62 (dm, J = 16 Hz, 1 H), 2.96 (dd, J = 11, 6 Hz, 1 H), 3.06 (dd, J = 11, 4 Hz, 2 H), 3.52–3.76 (m, 2 H), 3.85 (s, 3 H), 3.87 (s, 3 H), 6.58 (s, 1 H), 6.68 (s, 1 H). The spectrum was identical with the data reported for (–)-protoemetinol in the literature.6a
ACKNOWLEDGEMENT
This work was supported by a Grant-in-Aid for Scientific Research from the Ministry of Education, Science, Sports, and Culture, Japan.
References
1. (a) M. Hesse, Alkalids, Wiley, New York, 2002; (b) T. Fujii and M. Ohba, Heterocycles, 1988, 27, 1009. CrossRef
2. (a) M. Ihara, N. Taniguchi, K. Fukumoto, and T. Kametani, J. Chem. Soc., Chem. Commun., 1987, 1438; CrossRef (b) M. Ihara, N. Taniguchi, K. Yasui, and K. Fukumoto, J. Chem. Soc., Perkin Trans. 1, 1990, 2771; CrossRef (c) M. Ihara, K. Yasui, N. Taniguchi, and K. Fukumoto, J. Chem. Soc., Perkin Trans. 1, 1990, 1469. CrossRef
3. (R)-Acetate: (a) T. Sugai and K. Mori, Synthesis, 1988, 19; CrossRef (b) K. Laumen and M. P. Schneider, J. Chem. Soc., Chem. Commun., 1986, 1298. CrossRef
4. (S)-Acetate: (a) C. R. Johnson and M. P. Braun, J. Am. Chem. Soc., 1993, 115, 11014; CrossRef (b) K. Laumen and M. Schneider, Tetrahedron Lett., 1984, 25, 5875; CrossRef (c) Y.-F. Wang, C.-S. Chen, G. Girdaukas, and C. J. Sih, J. Am. Chem. Soc., 1984, 106, 3695; CrossRef (d) S. Takano, K. Tanigawa, and K. Ogasawara, J. Chem. Soc., Chem. Commun., 1976, 189. CrossRef
5. Recent and selected syntheses of dihydrocorynantheol: (a) M. Amat, A. Gómez-Esqué, C. Escolano, M. M. M. Santos, E. Molins, and J. Bosch, J. Org. Chem., 2009, 74, 1205; CrossRef (b) T. Itoh, M. Yokoya, K. Miyauchi, K. Nagata, and A. Ohsawa, Org. Lett., 2006, 8, 1533; CrossRef (c) A. Deiters, M. Pettersson, and S. F. Martin, J. Org. Chem., 2006, 71, 6547; CrossRef (d) A. Tosaka, S. Ito, N. Miyazawa, M. Shibuya, K. Ogasawara, and Y. Iwabuchi, Heterocycles, 2006, 70, 153; CrossRef (e) A. Deiters and S. F. Martin, Org. Lett., 2002, 4, 3243; CrossRef (f) M. Ohba, T. Ohashi, and T. Fjii, Heterocycles, 1991, 32, 319; CrossRef (g) R. L. Beard and A. I. Meyers, J. Org. Chem., 1991, 56, 2091; CrossRef (h) S. Takano, K. Masuda, and K. Ogasawara, J. Chem. Soc., Chem. Commun., 1980, 887. CrossRef
6. Recent and selected syntheses of protoemetinol: (a) J.-K. Chang, B.-R. Chang, Y.-H. Chuang, and N.-C. Chang, Tetrahedron, 2008, 64, 9685; CrossRef (b) C.-G. Huang, B.-R. Chang, and N.-C. Chang, Tetrahedron Lett., 2002, 43, 2721; CrossRef (c) T. Fujii, M. Ohba, K. Yoneyama, and H. Kizu, Chem. Pharm. Bull., 1985, 33, 358; (d) Y. Hirai, A. Hagiwara, and T. Yamazaki, Heterocycles, 1986, 24, 571. CrossRef
7. (a) H. P. Acharya and Y. Kobayashi, Tetrahedron, 2006, 62, 3329; CrossRef (b) J. Igarashi, M. Katsukawa, Y.-G. Wang, H. P. Acharya, Y. Kobayashi, Tetrahedron Lett., 2004, 45, 3783. CrossRef
8. S. Takano, S. Hatakeyama, and K. Ogasawara, Tetrahedron Lett., 1978, 19, 2519. CrossRef