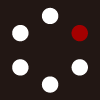
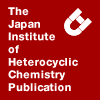
HETEROCYCLES
An International Journal for Reviews and Communications in Heterocyclic ChemistryWeb Edition ISSN: 1881-0942
Published online by The Japan Institute of Heterocyclic Chemistry
e-Journal
Full Text HTML
Received, 9th May, 2011, Accepted, 27th June, 2011, Published online, 1st July, 2011.
DOI: 10.3987/COM-11-S(P)19
■ Chemo-Enzymatic Synthesis of 1’-Photoreactive Sucrose Derivatives via Ether Linkage
Yuka Tsunekawa, Katsuyoshi Masuda, Miho Muto, Yasuyuki Muto, Yuta Murai, Yasuyuki Hashidoko, Yoshitake Orikasa, Yuji Oda, Yasumaru Hatanaka, and Makoto Hashimoto*
Graduate School of Agriculture, Hokkaido University, Kita 9 Nishi 9, Kita-ku, Sapporo, Hokkaido 060-8589, Japan
Abstract
As the 1’-hydroxyl group of sucrose is well known to be less reactive than other primary alcohols, there are no reports on the substitution of a phenoxy group at this position. Chemo-enzymatic synthesis of photoreactive 1’-phenoxy-substituted sucrose was examined to elucidate the functional analysis of sweet receptors.Humans can discriminate five basic tastes: saltiness, sourness, sweetness, bitterness, and umami.1 Sweetness is one of the most important tastes because it is related to food acceptance.2 Sweet taste qualities are generally regarded as appetit sensations of an energy source. Sucrose and fructose are recognized as sweet but not all sucrose and fructose derivatives are sweet, even if a substitution is introduced into one hydroxyl group. The detailed relationship of the structure and biological activity has not been completely clarified. Photoaffinity labeling is one of the methods used in the study of the interactions of low molecular bioactive compounds with biomolecules.3 Various photophores, such as benzophenone, arylazide and 3-(trifluoromethyl) phenyldiazirine, are used to elucidate the ligand-receptor or substrate-enzyme interactions. Chemical designs are important for applications of photoaffinity labeling to elucidate the functional analysis of sweetness. We have reported that diazirinyl photophore was appropriate for the analysis of sweet receptors.4 It was found that several sucrose-based oligosaccharides have sweet activities and 1-kestose (GF2), which has an additional fructose, was linked at 1’-position of the fructose unit of sucrose and acted as a sweetener.5 The results indicated that the 1’-position of the sucrose will be acceptable for substitutions. Although selective esterification of 1’- position was achieved by biotransformation,6 the ester linkage seems easily hydrolyzed under physiological conditions and metabolism during analysis. On the other hand, ether (phenoxy) linkages seem more stable than esters for functional analysis.7 The Mitsunobu reaction of one of the most popular reactions for the formation of ether linkages between different alcohols.8 Etherification9 and azidation10 of sucrose have been reported at 6- and 6’-positions, but to the best of our knowledge, there is no report on substitutions at sucrose 1’-position via ether linkage. We here present the novel synthesis of 1’-phenoxy derivatives of sucrose, including trifluoromethyldiazirinyl moiety as the photophore for photoaffinity labeling.
Selective deprotection of 1’-position has to be achieved to prevent the reaction of other primary alcohols at 6- and 6’-positions. Several synthetic routes that have been reported for protected sucrose derivatives exclude 1’-position, but their routes needed several steps.11 It has been reported that selective enzymatic hydrolysis of octaacetylsucrose 1 with Alcalase at the 1’-acetyl group seems suitable for the purpose.12 The previous reports presented only the results of the main product after 24 and 72 hours. Our detailed investigations of the time course of the reaction mixture revealed that maximum selective hydrolysis of 1 at 1’-position afforded 2 by incubation at 37 °C for 24 hours with Alcalase 2.4L. The hydrolyzed 2 also became the substrate for further hydrolysis at 6’-position, affording 4. Although 4 was detected after 12-hour incubations, monohydrolyzation at 6’-position (3) was less observed (Scheme 1).13
Compound 2 was subjected to the Mitsunobu reaction with p-nitrophenol 5. To use minimum reagents, equivalent amount of p-nitrophenol was reacted under diisopropyl azodicarboxylate (DIAD) and triphenylphosphine (Ph3P) (each 1.5 equivalents) at room temperature, but no product was detected after 4 days. The products were detected when the reaction mixture was warmed at 50 °C and the yield was moderate (45%) after 40 hours. We found that the Mitsunobu reaction rate of 2 was dependent on the reaction temperature. Isolation yield became maximum when the reaction was subjected with 2 equivalents of DIAD and Ph3P at 60 °C for 20 hours (Scheme 2, entries 1-5).14
o-Nitrophenol 6 was same reactivity for the Mitsunobu reaction of 2 (Scheme 2, entries 6, 7). Compared with previous results of the Mitsunobu reaction at 6’-position of sucrose,9 the reactivity of 1’ hydroxyl group in sucrose was lower than other sucrose primary alcohols and heating was required to react with compound 2, despite using o- or p- nitrophenol, which are good reactants for the Mitsunobu reaction.
m-Diazirinyl phenol 915 was subjected to the Mitsunobu reaction with compound 2 to synthesize photoaffinity label reagents. The reaction under established conditions of previous experiments for nitrophenols (equivalent phenol and 1.5 equivalents of DIAD and Ph3P at 60 °C) was applied for 9, but no condensation product was detected after 24 hours. Various proportions of the phenol and reactants were tested and the results indicated that excess m-diazirinyl phenol 9 was needed to obtain the condensation product 1116 (Scheme 3, entries 1-6). The m-diazirinyl phenol 9 was less reactive than p- or o- nitrophenols for the Mitsunobu reaction, because there was no electron-withdrawing group at the o- or p-position against the hydroxyl group. Furthermore, the 1’-hydroxyl group was less reactive than the other hydroxyl groups, and excess reactant and reagents were needed for the reaction to proceed under heating. The Tsunoda reagent (cyanomethylenetributylphosphorane)17 were also subjected to the reaction, but no improvement was observed in the reaction with compound 9.
p-Diazirinyl phenol 1015 was also subjected to the Mitsunobu reaction. The triflluoromethyldiazirinyl moiety at p-position against the hydroxyl group acts as a relative electron-withdrawing characteristic in the alkyl substituent due to electron negativity at the tertially carbon atom, but no reaction was observed at room temperature and the diazirinyl group was decomposed when the reaction was subjected at 60 °C. The p-diazirinyl phenol 10 has been utilized to form ether linkage in the Mitsunobu reaction in previous reports.18 The reported reaction was set at room temperature for all compounds with good yields. Our results indicated that Mitsunobu reaction of sucrose derivative 2 and diazirinyl phenols 9 or 10 were regulated by two factors. One is low reactivity of the 1’-hydroxyl group of sucrose. The other is thermal stability of the diazirinyl group under the condition (Scheme 3).
1’-O-aryl heptaacetylsucroses 7, 8 and 11 were subjected to deacetylation with NH3-methanol at room temperature to afford 1’-substituted sucroses 13, 14 and 15, respectively, with good yields without decomposition of the substituent.19
The establishment of chemoenzymatic modification at the less reactive 1’-position of sucrose will be useful to elucidate the functional analysis of sweet receptors. Bioactivities of the synthetic compounds for the sweet receptor are now under investigation.
ACKNOWLEDGEMENTS
This research was partially supported by a Ministry of Education, Science, Sports and Culture Grant-in-Aid for Scientific Research in a Priority Area, 18032007 and for Scientific Research (C), 19510210, 21510219 (M.H.).
References
1. B. Lindemann, Nature, 2001, 413, 219. CrossRef
2. P. A. Temussi, Trends Biochem. Sci., 2009, 34, 296. CrossRef
3. J. Brunner, Annu. Rev. Biochem., 1993, 62, 483; CrossRef Y. Hatanaka, H. Nakayama, and Y. Kanaoka, Rev. Heteroatom Chem., 1996, 14, 213; T. Tomohiro, M. Hashimoto, and Y. Hatanaka, Chem. Records, 2005, 5, 385; CrossRef M. Hashimoto and Y. Hatanaka, Eur. J. Org. Chem., 2008, 2513. CrossRef
4. K. Masuda, A. Koizumi, T. Misaka, Y. Hatanaka, K. Abe, T. Tanaka, M. Ishiguro, and M. Hashimoto, Bioorg. Med. Chem. Lett., 2010, 20, 1081. CrossRef
5. T. Oku, T. Tokunaga, and N. Hosoya, J. Nutr., 1984, 114, 1574.
6. G. Carrea, S. Riva, F. Secundo, and B. Danieli, J. Chem. Soc., Perkin Trans. 1, 1989, 105.
7. R. Matsumi, H. Atomi, A. J. M. Driessen, and J. van der Oost, Res. Microbiol., 2011, 162, 3. CrossRef
8. O. Mitsunobu, Synthesis, 1981, 1. CrossRef
9. M.-C. Scherrmann, A. Boutboul, B. Estramareix, A.-S. Hoffmann, and A. Lubineau, Carbohydr. Res., 2001, 334, 295. CrossRef
10. C. Besset, S. Chambert, B. Fenet, and Y. Queneau, Tetrahedron Lett., 2009, 50, 7043. CrossRef
11. M. T. Barros, C. D. Maycock, and C. Thomassigny, Carbohydr. Res., 2000, 328, 419. CrossRef
12. K.-Y. Chang, S.-H. Wu, and K.-T. Wang, Carbohydr. Res., 1991, 222, 121; CrossRef S. Bornemann, J. M. Cassells, J. S. Dordick, and A. J. Hacking, Biocatalysis, 1992, 7, 1. CrossRef
13. The isolation yields of deacylated compounds were lower than those of previous reports. No details for specific activity of enzyme were described in the previous reports. We encouraged improvements (amounts of enzyme, incubation temperature, incubation time etc) of the yields with several lots of Alcalase 2.4L with identical manners of previous reports, but no more further improvments were archieved.
14. General procedures for Mitsunobu reaction: To 2 (500 mg, 0.785 mmol) in dry toluene (1 mL) at 0 ℃, DIAD (187.5 μL, 1.178 mmol) and Ph3P (309 mg, 1.178 mmol) were added at 0 ℃ and stirred at the same temp. for 1.5 h. To this solution, phenol derivatives (1.178 mmol) were added and the reaction mixture was subjected to appropriate conditions, as in the text. The solvent was removed in vacuo and the residue was purified by silica gel column with ethyl acetate : hexane to afford the desired product. 1’-O-p-nitrophenyl heptaacetylsucrose (7) yield 90%, 1H-NMR (CDCl3) δ: 2.03 (3H, s), 2.04 (3H, s), 2.05 (3H, s), 2.09 (3H, s), 2.10 (3H, s), 2.13 (3H, s), 2.17 (3H, s), 4.05 - 4.34 (8H, m), 4.89 (1H, dd, J = 10.6, 3.7 Hz), 5.10 (1H, t, J = 10.0 Hz), 5.46 (1H, t, J = 10.0 Hz), 5.48 (1H, t, J = 6.6 Hz), 5.67 (1H, d, J = 6.9 Hz), 5.79 (1H, d, J = 3.4 Hz), 7.01 (2H, d, J = 9.2 Hz), 8.24 (2H, d, J = 9.2 Hz). 13C-NMR (CDCl3) δ : 20.56 (2C), 20.63 (2C), 20.69 (2C), 21.91, 61.51, 63.03, 68.02, 68.53, 68.91, 69.55, 70.21, 74.06, 75.65, 78.74, 89.71, 103.62, 114.82 (2C), 125.95 (2C), 142.21, 162.64, 169.48, 169.79, 169.87, 170.06, 170.12, 170.50, 170.63, HR-FAB-MS calcd for C32H40NO20 758.2144, found 758.2158, 1’-O-o-nitrophenyl heptaacetylsucrose (8) yield 85%, 1H-NMR (CDCl3) δ: 2.03 (3H, s), 2.05 (3H, s), 2.07 (3H, s), 2.10 (3H, s), 2.12 (3H, s), 2.14 (3H, s), 2.15 (3H, s), 4.00 - 4.28 (8H, m), 4.95 (1H, dd, J = 10.3, 4.0 Hz), 5.12 (1H, t, J = 9.7 Hz), 5.46 (1H, t, J = 9.7 Hz), 5.58 (1H, t, J = 8.0 Hz), 5.82 (1H, d, J = 8.0 Hz), 5.85 (1H, d, J = 3.4 Hz), 7.05 (1H, d, J = 8.0 Hz), 7.11 (1H, t, J = 8.0 Hz), 7.58 (1H, t, J = 8.0 Hz), 7.96 (1H, d, J = 8.0 Hz). 13C-NMR (CDCl3) δ: 19.85 (2C), 19.89 (2C), 19.92 (2C), 19.99, 60.78, 61.84, 67.36, 67.71, 69.04, 69.12, 69.25, 72.03, 74.99, 77.00, 88.57, 101.99, 113.54, 120.51, 125.49, 133.88, 138.55, 150.72, 168.72, 169.03, 169.24, 169.38, 169.45, 169.77, 169.89. HR-FAB-MS calcd for C32H40NO20 758.2144, found 758.2160.
15. Y. Hatanaka, M. Hashimoto, H. Kurihara, H. Nakayama, and Y. Kanaoka, J. Org. Chem., 1994, 59, 383. CrossRef
16. 1’-O-m-trifluoromethyldiazirinylphenyl heptaacetylsucrose (11) yield 10%, 1H-NMR (CDCl3) δ: 2.02 (3H, s), 2.03 (3H, s), 2.05 (3H, s), 2.07 (3H, s), 2.11 (3H, s), 2.14 (3H, s), 2.16 (3H, s), 4.06-4.36 (8H, m), 4.92 (1H, dd, J = 10.6, 3.7 Hz), 5.08 (1H, t, J = 10.0 Hz), 5.52 (2H, m), 5.70 (1H, d, J = 6.9 Hz), 5.82 (1H, d, J = 3.4 Hz), 6.62 (1H, s), 6.68 (1H, d, J = 8.0 Hz), 6.85 (1H, d, J = 8.0 Hz), 7.21 (1H, t, J = 8.0 Hz), 13C-NMR (CDCl3) δ: 20.54 (2C), 20.63 (2C), 20.72 (2C), 20.75, 29.4 (q, 2JCF = 40 Hz), 61.51, 63.03, 68.02, 68.53, , 68.91, 69.55, 70.21, 74.06, 75.65, 78.74, 89.71, 103.62, 110.7, 111.1, 114.82, 123.6 (q, 1JCF = 274 Hz), 125.95, 130.6, 132.3, 136.0, 142.21, 161.0, 162.64, 169.87, 19F-NMR (CDCl3) δ: -66.5, HR-FAB-MS calcd for C34H40F3N2O18 821.2228, found 821.2240.
17. T. Tsunoda, M. Nagaku, C. Nagino, Y. Kawamura, F. Ozaki, H. Hioki, and S. Ito, Tetrahedron Lett., 1995, 36, 2531; CrossRef T. Tsunoda, F. Ozaki, N. Shirakata, Y. Tamaoka, H. Yamamoto, and S. Ito, Tetrahedron Lett., 1996, 37, 2463. CrossRef
18. T. Shigenari, T. Hakogi, and S. Katsumura, Chem. Lett., 2004, 33, 594. CrossRef
19. 1’-O-p-Nitrophenyl sucrose (13) yield 95%, 1H-NMR (CD3OD) δ: 3.36 (1H, t, J = 9.7 Hz), 3.43 (1H, dd, J = 9.7, 4.0 Hz), 3.65-3.90 (7H, m), 4.08 (1H, t, J = 8.6 Hz), 4.20-4.30 (3H, m), 5.47 (1H, t, J = 4.0 Hz), 7.14 (2H, d, J = 8.9 Hz), 8.22 (2H, d, J = 8.9 Hz). 13C-NMR (CD3OD) δ: 62.24, 63.28, 69.02, 71.43, 73.07, 74.41, 74.60, 74.92, 78.23, 83.81, 94.03, 104.30, 116.10 (2C), 126.80 (2C), 143.13, 165.04, HR-FAB-MS calcd for C18H26NO13 464.1404, found 464.1422. 1’-O-o-Nitrophenylsucrose (14) yield 92%, 1H-NMR (CD3OD) δ: 3.36 (1H, t, J = 9.7 Hz), 3.42 (1H, dd, J = 9.7, 4.0 Hz), 3.69-3.88 (7H, m), 4.09 (1H, t, J = 8.6 Hz), 4.23 (2H, m), 4.38 (1H, d, J = 8.6 Hz), 5.48 (1H, d, J = 4.0 Hz), 7.10 (1H, t, J = 8.0 Hz), 7.28 (1H, d, J = 8.0 Hz), 7.61 (1H, t, J = 8.0 Hz), 7.86 (1H, d, J = 8.0 Hz). 13C-NMR (CD3OD) δ : 62.17, 63.00, 69.41, 71.41, 73.03, 74.42, 74.51, 74.59, 77.91, 83.58, 93.94, 104.15, 116.09, 121.93, 126.49, 135.51, 141.16, 153.00, HR-FAB-MS calcd for C18H26NO13 464.1404, found 464.1418. 1’-O-m-Trifluoromethyldiazirinyl sucrose (15) yield 90%, 1H-NMR (CD3OD) δ: 3.34 (1H, t, J = 9.7 Hz), 3.40 (1H, d, J = 9.7, 4.0 Hz), 3.68-3.90 (7H, m), 4.14-4.20 (3H, m), 4.25 (1H, d, J = 8.6 Hz), 5.51 (1H, d, J = 4.0 Hz), 6.75 (1H, s), 6.90 (1H, d, J = 8.0 Hz), 7.13 (1H, d, J = 8.0 Hz), 7.41 (1H, t, J = 8.0 Hz), 13C-NMR (CD3OD) δ: 29.6 (q, 2JCF = 40 Hz), 62.24, 63.27, 69.01, 71.44, 73.07, 74.42, 74.61, 74.92, 78.23, 83.81, 94.03, 104.30, 110.9, 111.0, 123.4 (q, 1JCF = 274 Hz), 130.5, 132.0, 135.5, 160.0. 19F-NMR (CD3OD) δ: -66.5, HR-FAB-MS calcd for C20H26F3N2O11 527.1489, found 527.1470.