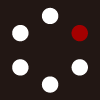
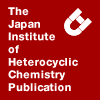
HETEROCYCLES
An International Journal for Reviews and Communications in Heterocyclic ChemistryWeb Edition ISSN: 1881-0942
Published online by The Japan Institute of Heterocyclic Chemistry
e-Journal
Full Text HTML
Received, 30th September, 2012, Accepted, 8th November, 2012, Published online, 9th November, 2012.
DOI: 10.3987/COM-12-S(N)127
■ Reaction of 1,2,3-Oxadiazole 3-Oxides
Takumichi Sugihara,* Akira Murakawa, Chiemi Sawanaka, Megumi Seki, Natsuki Iio, Ayano Iizuka, Takuya Oohora, and Shinobu Honzawa
Niigata University of Pharmacy and Applied Life Sciences, 265-1, Higashi-jima, Niigata 956-8603, Japan
Abstract
1,2,3-Oxadiazole 3-oxides with an alkoxymethyl group at the 5-position were reduced by reacting them with sodium borohydride to produce 4,5-dihydro-1,2,3-oxadiazole 3-oxides in good yields, which were further transformed into substituted diazene N-oxides by reacting with Grignard reagents.Oxadiazoles are five-membered heteroaromatic compounds consisting of two carbons, two nitrogens, and one oxygen with two double bonds; they are used as mother nuclei and/or subunits of pharmaceutical drugs, such as butalamine, fasiplon, oxolamine, pleconaril, and raltegravir. Oxadiazoles have four isomers: 1,2,3-, 1,2,4-, 1,2,5-, and 1,3,4-isomers. Although the later three are stable under ambient temperature, the first one 1 is unstable and is easily tautomerized to α-diazocarbonyl compounds 2 (Eq. 1).1
We have developed a new and convenient method of synthesizing 1,2,3-oxadiazole 3-oxides 3 by reacting alkynyllithiums with nitric oxide at atmospheric pressure and low temperatures (Eq. 2).2 The reaction is clean and fast, producing 3 in good yield when oxygen contamination is eliminated completely.
Most N-oxides of nitrogen-containing heteroaromatic compounds are unstable when heated. 1,2,5-Oxadiazole 2-oxides are the same, decomposing into nitrile oxides and isocyanates easily.3 In contrast, their isomers 1,2,3-oxadiazole 3-oxides 3 are thermally stable and are not decomposed after refluxing in xylene for 10 h; however, 1,2,3-oxadiazoles 1 are less stable than 1,2,5-isomers. If the stable and easily accessible 1,2,3-oxadiazole 3-oxides 3 are reduced, this will become a new method of producing α-diazocarbonyls, α-aminocarbonyls, and β-amino alcohols. Thus, we started to study the reaction of 1,2,3-oxadiazole 3-oxides 3 with various reducing agents.
The introduction of phenyl substituents into the starting molecules facilitates handling and reaction checking. Thus, five types of 1,2,3-oxadiazole 3-oxide with phenyl substituents, i.e., 5-phenyl-1,2,3-oxadiazole 3-oxide 3a, 5-(2-phenylethyl)-1,2,3-oxadiazole 3-oxide 3b, 5-phenoxymethyl-1,2,3-oxadiazole 3-oxide 3c, 5-(3-phenoxypropyl)-1,2,3-oxadiazole 3-oxide 3d, and 5-(3-benzyloxypropyl)-1,2,3-oxadiazole 3-oxide 3e were synthesized from the corresponding alkynes according to the previous method2 and used for studying their reactivity.
First, catalytic hydrogenation in the presence of palladium on activated carbon was applied to 3a-e, since one of their isomers, 1,2,5-oxadiazole 2-oxide, was reported to deoxygenate under the same conditions.4 The results are summarized in Table 1.
1,2,3-Oxadiazole 3-oxides 3 are slightly solved in methanol, and thus, whether a large amount of methanol or the addition of dichloromethane is required to carry out the reaction in solution. A mixture of 3a and a catalytic amount of palladium on activated carbon in methanol was stirred at 20 °C under atmospheric pressure of hydrogen for 2 h. Unfortunately, the desired 1,2,3-oxadiazole 1a (R1 = Ph, R2 = H) or their tautomers, α-diazocarbonyl compound 2a, were not obtained, and the simple carbonyl compound 4a was produced in an almost quantitative yield (Entry 1). Changing the solvent to a mixture of methanol and dichloromethane shortened the reaction time (Entry 2); however the reaction in dichloromethane lowered the yield of 4a (Entry 3). 1,2,3-Oxadiazole 3-oxides having 2-phenylethyl and 3-phenoxypropyl groups 3b and 3d were also reduced smoothly to produce 4b and 4d, respectively (Entries 4 and 6), whereas the reaction of 3c produced phenol 5 in an almost quantitative yield (Entry 5). The hydrogenation of 1-phenoxyacetone under the same conditions did not produce phenol 6, and no 5-methyl-1,2,3-oxadiazole 3-oxide was obtained after the reaction. Therefore, the hydrogenolytic removal of the 5-methylene-1,2,3-oxadiazole 3-oxide part seemed to be faster than the reduction of this part, and the resulting 5-methyl-1,2,3-oxadiazole 3-oxide might be transformed into acetone under the same conditions. Both the hydrogenation of the 1,2,3-oxadiazole 3-oxide part and the hydrogenolytic cleavage of the arylmethyl part proceeded at the same time when 3e was reacted to give 5-hydroxy-2-pentanone in a good yield (Entry 7). 1,2,3-Oxadiazole 3-oxides 3 were prepared from their parent terminal alkynes under basic conditions, and the reduction of the resulting 3 to the carbonyl compounds 4 was carried out under neutral conditions. Thus, the overall reaction from alkynes to carbonyl compounds 4 appeared to be an alternative to the Markovnikov-type ‘formal hydration’ of alkynes and could be carried out under basic to neutral conditions. The Markovnikov-type hydration of the terminal alkynes is generally carried out under acidic conditions as shown in Scheme 1. Thus, the present overall transformation becomes an alternative to the conventional method.
Catalytic hydrogenation produced neither the desired 2 nor 3, and thus we switched to conventional reducing agents having nucleophilic hydrides. The reaction of 3a-e with lithium aluminum hydride produced a number of compounds, and thus mild boron-based reducing agents were chosen and reacted with 3. The results are summarized in Table 2.
The reaction of 3a, 3b, 3d, and 3e with excess sodium borohydride in methanol did not proceed, which led to the recovery of these compounds in quantitative yields (Entries 1, 2, 7, and 8). In contrast, the reaction of 3c with excess sodium borohydride in methanol proceeded smoothly to produce 7c in a good yield (Entry 3).5,6 A comparison of Entries1-3 and 7 revealed that the position of the oxygen atom in the side chain plays an important role in the successful reduction of the carbon-carbon double bond by reaction with sodium borohydride. Changing the solvent from methanol to a mixture of methanol and dichloromethane could reduce the amount of solvent used and make the reaction clean (Entry 4). When sodium cyanoborohydride and borane-tetrahydrofuran complex were used instead of sodium borohydride, 7c was also produced in moderate yield in the former case (Entry 5), and 3c was recovered in quantitative yields in the latter case (Entry 6), respectively. Thus, sodium borohydride was important to reduce the carbon-carbon double bond in 1,2,3-oxadiazole 3-oxides. The efficient reduction of the carbon-carbon double bond also proceeded by changing the substituent on methanol from a phenyl group to 4-methoxyphenyl, benzyl, triphenylmethyl, and 3-phenylpropyl groups (Entries 9-12);5 thus, the presence of aryloxymethyl and alkoxymethyl groups at the 5-position of 1,2,3-oxadiazole 3-oxides seemed to be important for the reduction.7
Although 5-phenoxymethyl-1,2,3-oxadiazole 3-oxide 3c was reacted with neither n-butyllithium nor ethylmagnesium chloride, its reduced form 4,5-dihydro-5-phenoxymethyl-1,2,3-oxadiazole 3-oxide 7c was reacted with certain Grignard reagents to produce diazene N-oxides 8. The results of the reaction with a couple of organometallic regents are summarized in Table 3.
Although the reaction of 7c with methylmagnesium chloride was sluggish to produce 8a in moderate yield (Entry 1),5 that with ethylmagnesium chloride proceeded smoothly to produce unsymmetrical diazene N-oxide 8b in good yield (Entry 2).5,8 The isopropyl group could also be introduced into the nitrogen atom (Entry 3).5 However, nothing happened when tert-butylmagnesium chloride was used (Entry 4). The reaction with n-butyllithium gave a complex mixture (Entry 5) and that with ethylzinc chloride recovered the starting compound 7c in an almost quantitative yield (Entry 6). In addition, nothing happened when 3c was reacted with ethylmagnesium chloride. Thus, the reduction of the double bond of 1,2,3-oxadiazole 3-oxides 3c and the reaction with Grignard reagents having primary and secondary alkyl groups are important for the success of the desired substitution reaction.
We elucidated herein some reactivity of 1,2,3-oxadiazole 3-oxides 3 and developed a new route to unsymmetrical diazene N-oxides by reacting with sodium borohydride and then with Grignard reagents. The resulting 7 and 8 are considered as the precursors of substituted β-aminoalcohols, which are important mother nuclei of sympathomimetic drugs. Further development is now under way, and the dream that ‘medicines can be made from a pollutant (nitric oxide)’ will come true soon in the near future.
ACKNOWLEDGEMENTS
This work was supported in part by a Grant-in-Aid for Scientific Research from the Ministry of Education, Culture, Sports, Science and Technology, Japan.
References
1. J. A. Joule, K. Mills, and G. F. Smith, ‘Heterocyclic Chemistry, 3rd ed.,’ Chapman & Hall, London, 1995.
2. T. Sugihara, K. Kuwahara, A. Wakabayashi, H. Takao, H. Imagawa, and M. Nishizawa, Chem. Commun., 2004, 216. CrossRef
3. J. A. Chapman, J. Crosby, C. A. Cummings, R. A. C. Rennie, and R. M. Paton, J. Chem. Soc., Chem. Commun., 1976, 240. CrossRef
4. T. K. Kim, J. H. Choe, B. W. Lee, and K.-H. Chung, Bull. Korean Chem. Soc., 2012, 33, 2765. CrossRef
5. All new compounds were characterized by spectroscopic methods (IR, 1H- and 13C-NMR, MS).
6. The spectroscopic data of the typical compound 7c are as follows: IR (KBr) 1590, 1499, 1473, 1239, 996 cm-1; 1H NMR (400 MHz, CDCl3) δ 4.22 (dd, J = 3.6, 10.8 Hz, 1 H), 4.30 (dd, J = 4.4, 10.8 Hz, 1 H), 4.65 (d, J = 9.2 Hz, 2 H), 5.34 (ddt, J = 3.6, 4.4, 9.2 Hz, 1 H), 6.92 (ddd, J = 1.2, 2.0, 8.0 Hz, 2 H), 7.03 (dd, J = 1.2, 7.6 Hz, 1 H), 7.32 (ddd, J = 2.0, 7.6, 8.8 Hz, 2 H); 13C NMR δ 63.41, 66.39, 78.67, 114.60, 122.09, 129.68, 157.57; MS (EI) m/z 194 (M+), 134 (100%), 119, 94, 77, 65. HR-MS (EI) calcd. for C9H10N2O3 (M+) 194.0692, found 194.0694.
7. The investigation to clarify the reaction mechanism is now under way.
8. The spectroscopic data of the typical compound 8b are follows: IR (KBr) 3442, 297, 2937, 1598, 1588, 1497, 1458, 1424, 1317, 1244, 1078, 1044, 754, 692 cm-1; 1H NMR (400 MHz, CDCl3) δ 1.31 (t, J = 7.4 Hz, 3 H), 3.52 (tq, J = 1.1, 7.4 Hz, 2 H), 3.76 (d, J = 4.7 Hz, 1 H), 4.02 (dd, J = 6.1, 9.6 Hz, 1 H), 4.12 (d, J = 4.6, 9.6 Hz, 1 H), 4.41 (ddt, J = 1.1, 8.1, 13.2 Hz, 1 H), 4.49 (ddt, J = 1.1, 2.9, 13.2 Hz, 1 H), 4.58 (m, 1 H), 6.91 (ddd, J = 1.2, 2.4, 8.8 Hz, 2 H), 6.99 (dd, J = 1.2, 7.2 Hz, 1 H), 7.30 (ddd, J = 2.4, 7.2, 8.8 Hz, 2 H); 13C NMR δ 11.74, 46.72, 67.61, 68.56, 71.25, 114.43, 121.34, 129.48, 158.07; MS (EI) m/z 224 (M+), 207, 131 (100%), 107, 94, 77, 65. HR-MS (EI) calcd. for C11H16N2O3 (M+) 224.1162, found 224.1152.