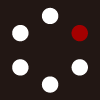
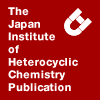
HETEROCYCLES
An International Journal for Reviews and Communications in Heterocyclic ChemistryWeb Edition ISSN: 1881-0942
Published online by The Japan Institute of Heterocyclic Chemistry
e-Journal
Full Text HTML
Received, 23rd June, 2012, Accepted, 16th August, 2012, Published online, 23rd August, 2012.
DOI: 10.3987/COM-12-S(N)52
■ THE FIRST TOTAL SYNTHESES OF SCHOLAREINS A, C, AND D EMPLOYING 2ND GENARATION PALLADIUM-CATALYZED CYCLOALKENYLATION
Yasufumi Kawai, Megumi Saeki, and Masahiro Toyota*
Department of Chemistry, Graduate School of Science, Osaka Prefecture University, 1-1 Gakuencho, Sakai, Osaka 593-8531, Japan
Abstract
(±)-Scholareins A (1), C (3), and D (4) have been synthesized for the first time by a 2nd generation palladium-catalyzed cycloalkenylation. In addition, total synthesis of (±)-boonein (5) has also been achieved by applying the same catalytic cyclization as the key step.INTRODUCTION
In 1983, boonein (5), a possible precursor in the indole alkaloid biogenesis, was isolated from the bark of Alstonia boonei.1 Structurally similar 11-noriridoids, scholareins A (1), B (2), C (3), and D (4) were also isolated in 2008 from EtOH extract of the bark of Alstonia scholaris, which is widely distributed in the tropical regions of Africa and Asia.2 While two research groups have independently achieved total syntheses of boonein (5),3 no laboratory synthesis of scholareins has been described (Figure 1).
As part of our ongoing program on the synthesis of biologically active iridolactones via the 2nd generation palladium-catalyzed cycloalkenylation developed by us,4 we undertook the synthesis of boonein (5) and scholareins A-D (1-4). Herein, we disclose the first total syntheses of 11-noriridoids, scholareins A (1), C (3), and D (4), using the 2nd generation palladium-catalyzed cycloalkenylation.
RESULTS AND DISCUSSION
Since we have already demonstrated a 2nd generation palladium-catalyzed cycloalkenylation of olefinic lactone ester 6 in total syntheses of onikulactone and mitsugashiwalactone,5 we next focused on developing a concise method for transformation of 7 into further oxidized iridoids, such as boonein (5) and scholareins (1-4) (Scheme 1). It should be noted that the yield and the product ratio (7 versus 8) of the 2nd generation palladium-catalyzed cycloalkenylation of 6 could be increased after a prolonged reaction time.
After separation of compounds 7 and 8, exo-olefin 7 was subjected to allylic oxidation under a variety of conditions. Some of the conditions and yields examined for allylic oxidation of 7 are listed in Table 1. Although no allylic alcohol was obtained using SeO2 in CH2Cl2-AcOH, enone 11 was produced in 14% yield (entry 1). Allylic alcohols 9 and 10 were produced in 52% yield as an 11:9 separable mixture when the reaction was performed in the presence of 6.0 equivalents of SeO2 in CH2Cl2-AcOH-H2O (entry 2). The best result, 80% yield (based upon recovered starting material 7), was obtained for the reaction using 1.4 equivalents of SeO2 and 6.4 equivalents of tert-butyl hydroperoxide in CH2Cl2-THF-HMPA (3.8:8.5:1 v/v) as solvent (entry 3). The relative stereochemistry of 10 was established on the basis of NOE correlation as depicted in Figure 2. It should be noted that the reaction solvent is a critical factor in the allylic oxidation. Investigations into the solvent effect are underway.
With allylic alcohol 9 in hand, a stereoselective hydrogenation was next conducted in the presence of a catalytic amount of Rh-Al2O3 in EtOH to lead to a 4:1 separable mixture of 12 and 13 in nearly quantitative yield. The relative stereochemistry of the major product 12 was elucidated by the NOE correlation shown in Figure 3. Finally, 12 was transformed into (±)-boonein (5) after removal of the ester moiety under the Krapcho reaction conditions (Scheme 2).6 The 1H and 13C NMR spectra of the synthetic (±)-boonein (5) were identical to those reported.1
To assemble (±)-scholareins A (1), C (3), and D (4), (±)-boonein (5) was reduced with 2 equivalents of DIBAL-H to give rise to (±)-scholarein C (3) in 98% yield. Upon treatment of 3 with PPTS in MeOH-CH2Cl2 at 0 °C, (±)-scholarein D (4) was afforded as a major acetal together with 14. Although (±)-scholarein D (4) was easily separable, (±)-scholarein A (1) was directly produced from a mixture of (±)-scholarein D (4) and 14 in 94% yield when the mixture was treated with boron trifluoride etherate in the presence of triethylsilane (Scheme 3).7 The spectral properties of the synthetic compounds 1, 3 and 4 were identical to the corresponding previously reported spectra.2
In conclusion, we have achieved the first total synthesis of (±)-scholareins A (1), C (3), and D (4) by means of 2nd generation palladium-catalyzed cycloalkenylation. The methodology developed here should also provide access to synthetic analogues of scholareins.
EXPERIMENTAL
Unless otherwise noted, all reactions were performed in an oven-dried glassware, sealed with a rubber septum under an atmosphere of argon. Anhydrous THF, CH2Cl2, and Et2O were purchased from Kanto Chemical Co., Inc. DMSO and HMPA were distilled from CaH2 under reduced pressure. Unless otherwise mentioned, materials were obtained from commercial suppliers and used without further purification. Flash column chromatography was carried out using Cica 60 N (spherical / 40-50 μm) silica gel. Reactions and chromatography fractions were analyzed employing precoated silica gel 60 F254 plates (Merck). Compounds were visualized using an ultraviolet lamp (254 nm) and/or by staining with p-anisaldehyde (in EtOH), phosphomolybdic acid (in EtOH), ammonium molybdate (in 10% H2SO4), or potassium permanganate (in water containing NaOH and K2CO3). IR spectra were measured on a SHIMADZU FT-IR 8300 spectrophotometer. 1H NMR spectra were recorded on Varian 400 MR (400 MHz), JEOL AL-400 (400 MHz) or JEOL JX-500 (500 MHz) spectrometers with tetramethylsilane (δ 0), CHCl3 (δ 7.26), C6H6 (δ 7.16), or CH3COCH3 (δ 2.05) as an internal standard. 13C NMR spectra were recorded on Varian 400 MR (100 MHz) spectrometers with CDCl3 or Acetone-d6 as an internal standard. Mass spectra were recorded on JEOL JMS-DX 303, JEOL JMS-AX 500, or JEOL JMS-AX 700 spectrometers. All melting points were determined on Yanaco micro melting point apparatus and are uncorrected. Analytical and semipreparative HPLC separation were performed by using a HPLC system composed of a Jacso PU-2086 pump, a Jasco UV-2075 detector, and a Jasco RI-2031 detector
(1SR,6RS)-Methyl 9-methylene-2-oxo-3-oxabicyclo[4.3.0]nonane-1-carboxylate (7) and (1SR,6RS)-Methyl 9-methyl-2-oxo-3-oxabicyclo[4.3.0]non-8-en-1-carboxylate (8): To a solution of 6 (50 mg, 0.24 mmol) in DMSO (0.50 mL), Pd(OAc)2 (5.39 mg, 0.024 mmol) was added at rt, and then the resulting mixture was stirred at 45 °C for 48 h under an atmosphere of oxygen. To the reaction mixture was added saturated aqueous NaHCO3 solution, and then the mixture was extracted three times with EtOAc. The combined organic phases were washed with brine, dried over MgSO4, and evaporated to afford a crude oil. The crude product was purified by flash column chromatography with hexane-EtOAc (3:1 v/v) as an eluent, giving 7 (35.9 mg, 71%) as a pale yellow oil and 8 (3.5 mg, 7%) as a pale yellow oil.
Data for 7: IR (neat) 1745, 1730 cm-1; 1H NMR (400 MHz, CDCl3) δ 5.41 (1H, dd, J = 2.0, 2.0 Hz), 5.33 (1H, dd, J = 2.0, 2.0 Hz), 4.36 (1H, ddd, J = 11.5, 4.8, 4.4 Hz), 4.42 (1H, ddd, J = 11.4, 10.2, 3.0 Hz), 3.77 (3H, s), 3.01 (1H, ddd, J = 13.3, 8.7, 6.8 Hz), 2.56-2.50 (2H, m), 2.08-1.94 (2H, m), 1.68 (1H, dddd, J = 14.3, 10.2, 8.6, 4.0 Hz), 1.53 (1H, dddd, J = 12.8, 7.8, 7.8, 6.4 Hz); 13C NMR (100 MHz, CDCl3) δ 170.9, 168.3, 146.7, 113.6, 67.7, 63.7, 53.4, 42.9, 31.4, 30.3, 27.2. Data for 8: IR (neat) 1745, 1730 cm-1; 1H NMR (400 MHz, CDCl3) δ 5.47 (1H, br s), 4.35 (1H, ddd, J = 11.2, 7.6, 3.2 Hz), 4.28 (1H, dddd, J = 11.2, 7.2, 2.8, 0.4 Hz), 3.78 (3H, s), 3.08-3.01 (1H, m), 2.79-2.70 (1H, m), 2.22-2.06 (2H, m), 1.87 (3H, br s), 1.77-1.69 (1H, m); 13C NMR (100 MHz, CDCl3) δ 171.1, 168.3, 137.0, 131.4, 67.7, 66.9, 53.1, 41.1, 38.1, 29.4, 14.8.
(1SR,6SR,8SR)-Methyl 8-hydroxy-9-methylene-2-oxo-3-oxabicyclo[4.3.0]nonane-1-carboxylate (9) and (1SR,6SR,8RS)-Methyl 8-hydroxy-9-methylene-2-oxo-3-oxa-bicyclo[4.3.0]nonane-1-carboxylate (10). To a suspension of selenium oxide (22.2 mg, 0.20 mmol) in CH2Cl2 (1.5 mL), tBuO2H (0.13 mL, 0.92 mmol) was added dropwise at rt. After 20 min of vigorous stirring, a solution of 7 (30 mg, 0.14 mmol) in THF (3.4 mL) and HMPA (5.0 mL, 28.7 mmol) was added to the mixture. The resulting mixture was heated at 80 °C and stirred at the same temperature for 24 h. After cooling to rt, a saturated aqueous solution of NaHCO3/Na2SO3 was added, and then the resulting mixture was stirred for 30 min. The mixture was extracted three times with CH2Cl2. The combined organic phases were washed with brine, dried over MgSO4, and evaporated to afford a crude oil. The crude product was purified by flash column chromatography with hexane-EtOAc (1:3 v/v) as an eluent, giving a separable mixture of 9 and 10 (18.2 mg, 80%, based on recovered starting material) as a pale yellow oil. Data for 9: IR (neat) 3437, 1728, 1267 cm-1; 1H NMR (400 MHz, CDCl3) δ 5.66 (2H, d, J = 7.2 Hz), 4.62-4.58 (1H, m), 4.37-4.25 (2H, m), 3.81 (3H, s), 3.23 (1H, dddd, J = 7.2, 7.2, 7.2, 7.2 Hz), 2.22-2.14 (2H, m), 2.04 (1H, ddd, J = 13.6, 7.6, 3.2 Hz), 1.78 (1H, ddd, J = 13.6, 8.0, 5.6 Hz), 1.66 (1H, ddd, J = 14.6, 7.6, 3.6 Hz); 13C NMR (100 MHz, CDCl3) δ 170.7, 168.3, 149.2, 114.3, 73.0, 67.0, 61.2, 53.5, 38.6, 38.3, 27.7; LRMS m/z 167 [(M-CO2CH3)+]; HRMS calcd for C11H14O5 (M+) 226.0841, C9H11O3 [(M-CO2CH3)+] 167.0708, found 167.0718. Data for 10: IR (neat) 3438, 1731, 1269 cm-1; 1H NMR (400 MHz, CDCl3) δ 5.57 (2H, dd, J = 2.0, 2.0 Hz), 4.62-4.57 (1H, m), 4.39 (1H, ddd, J = 11.4, 6.4, 3.6 Hz), 4.23 (1H, ddd, J = 10.4, 10.0, 2.8 Hz), 3.77 (3H, s), 2.94 (1H, dddd, J = 7.6, 7.6, 7.6, 7.6 Hz), 2.32 (1H, ddd, J = 13.2, 7.2, 7.2 Hz), 2.21-2.12 (2H, m), 1.88-1.79 (1H, m), 1.49 (1H, ddd, J = 7.6, 7.6, 13.2 Hz); 13C NMR (100 MHz, CDCl3) δ 171.6, 167.7, 148.9, 117.8, 74.5, 67.1, 61.9, 53.8, 40.2, 39.3, 27.3; LRMS m/z 167 [(M-CO2CH3)+]; HRMS calcd for C11H14O5 (M+) 226.0841, C9H11O3 [(M-CO2CH3)+] 167.0708, found 167.0710. Data for (1SR,6SR,8RS)-Methyl 2,8-dioxo-9-methylene-3-oxabicyclo[4.3.0]-nonane-1-carboxylate (11): IR (neat), 1730, 1647 cm-1; 1H NMR (400 MHz, CDCl3) δ 6.49 (1H, s), 6.03 (1H, s), 4.45- 4.42 (2H, m), 3.82 (3H, s), 3.19-3.12 (1H, m), 2.73 (1H, dd, J = 18.4, 8.4 Hz), 2.29 (1H, dd, J = 18.4, 3.2 Hz), 2.13-2.06 (1H, m), 1.73-1.63 (1H, m); 13C NMR (100 MHz, CDCl3) δ 171.6, 167.7, 148.9, 117.8, 74.5, 67.1, 61.9, 53.8, 40.2, 39.3, 27.3; LRMS m/z 165 [(M-CO2CH3)+]; HRMS calcd for C11H12O5 (M+) 224.0685, C9H9O3 [(M-CO2CH3)+] 165.0552, found 165.0552.
(1SR,6SR,8SR,9SR)-Methyl 8-hydroxy-9-methyl-2-oxo-3-oxabicyclo[4.3.0]nonane-1-carboxylate (12) and (1SR,6SR,8SR,9RS)-Methyl 8-hydroxy-9-methyl-2-oxo-3-oxabicyclo[4.3.0]nonane-1-carboxylate (13). To a stirred solution of 9 (32.0 mg, 0.14 mmol) in EtOH (3.0 mL), rhodium-alumina (10.3 mg, 0.050 mmol) was added. The mixture was stirred at rt under an atmosphere of hydrogen. After 2 h, the reaction mixture was filtered through Celite® and the filtrate was concentrated in vacuo to afford a crude oil. The crude product was purified by flash column chromatography with hexane-EtOAc (1:3 v/v) as an eluent, giving 12 (25.6 mg, 80%) as a colorless oil and 13 (6.40 mg, 20%) as a colorless oil. Data for 12: IR (neat) 3471, 1730, 1263 cm-1; 1H NMR (400 MHz, CDCl3) δ 4.35 (1H, ddd, J = 11.6, 2.8, 2.8 Hz), 4.07-4.01 (2H, m), 3.82 (3H, s), 3.25-3.18 (1H, m), 2.78-2.70 (1H, m), 2.31-2.21 (2H, m), 1.62-1.47 (2H, m), 1.20 (3H, d, J = 7.2 Hz); 13C NMR (100 MHz, CDCl3) δ 174.1, 171.3, 75.8, 67.7, 62.0, 53.8, 47.6, 43.2, 42.7, 30.7, 11.0; LRMS m/z 169 [(M-CO2CH3)+]; HRMS calcd for C11H16O5 (M+) 228.0998, C9H13O3 [(M-CO2CH3)+] 169.0865, found 169.0861. Data for 13: IR (neat) 3459, 1730, 1271 cm-1; 1H NMR (400 MHz, CDCl3) δ 4.45-4.34 (2H, m), 4.03 (1H, br s), 3.78 (3H, s), 3.04-2.97 (2H, m), 2.32-2.23 (2H, m), 2.06 (1H, dddd, J = 15.6, 8.8, 1.2, 1.2 Hz), 1.85 (1H, ddd, J = 13.7, 8.6, 4.8 Hz), 1.66 (1H, dddd, J = 14.0, 6.4, 6.4, 3.2 Hz), 1.05 (3H, d, J = 7.6 Hz); 13C NMR (100 MHz, CDCl3) δ 173.4, 168.9, 78.3, 67.3, 62.7, 53.7, 49.1, 39.4, 38.7, 27.9, 15.6; LRMS m/z 169 [(M-CO2CH3)+]; HRMS calcd for C11H16O5 (M+) 228.0998, C9H13O3 [(M-CO2CH3)+] 169.0865, found 169.0861.
(±)-Boonein (5). A mixture of 12 (5.3 mg, 0.023 mmol), lithium chloride (10.0 mg, 0.23 mmol), and one drop of H2O in DMSO (0.30 mL) was stirred at 150 °C for 2 h. After cooling to rt, H2O was added, and the resulting mixture was extracted three times with EtOAc. The combined organic phases were washed with brine, dried over MgSO4, and evaporated to afford a crude oil. The crude product was purified by flash column chromatography with hexane-EtOAc (1:3 v/v) as an eluent, giving 5 (3.7 mg, 95%) as a colorless solid; mp 92 °C. Data for (±)-boonein (5): IR (neat) 3415, 1698, 1273 cm-1; 1H NMR (400 MHz, CDCl3) δ 4.32 (1H, ddd, J = 11.2, 6.0, 3.2 Hz), 4.21 (1H, ddd, J = 11.2, 8.8, 2.4 Hz), 4.15-4.13 (1H, m), 2.93-2.82 (1H, m), 2.66 (1H, dd, J = 10.8, 10.8 Hz), 2.29-2.20 (1H, m), 2.14-2.06 (2H, m), 1.53-1.45 (2H, m), 1.24 (3H, d, J = 6.8 Hz); 13C NMR (100 MHz, CDCl3) δ 175.0, 75.4, 67.1, 47.3, 44.2, 41.2, 34.0, 29.6, 13.9; LRMS m/z 170 (M+); HRMS calcd for C9H14O3 (M+) 170.0943, found 170.944.
(±)-Scholarein C (3). To a stirred solution of 5 (12.5 mg, 0.073 mmol) in CH2Cl2 (3.7 mL) was added dropwise DIBAL-H (1.02 M solution in hexane, 0.14 mL, 0.15 mmol) at –78 °C. After 1 h of stirring at –78 °C, H2O was added dropwise over 5 min. The resulting mixture was slowly warmed to rt, and then hexane and EtOAc were added. The solution was cooled to 0 °C, and MgSO4 was added. After 30 min of stirring, the resulting suspension was filtered through Celite®. The filtrate was concentrated to afford a crude oil. The crude product was purified by flash column chromatography with hexane-EtOAc (1:3 v/v) as an eluent, giving 3 (12.3 mg, 98%) as a colorless solid; mp 103 °C. Data for (±)-scholarein C (3): IR (neat) 3362 cm-1; 1H NMR (400 MHz, Acetone-d6) δ 4.93 (1H, br s), 4.17-4.13 (1H, m), 3.87 (1H, ddd, J = 11.4, 11.4, 3.2 Hz), 3.37 (1H, ddd, J = 11.4, 3.6, 3.6 Hz), 2.41-2.32 (1H, m), 1.97-1.91 (1H, m), 1.80-1.61 (3H, m), 1.50-1.42 (1H, m), 1.34-1.27 (1H, m), 0.98 (3H, d, J = 7.2 Hz); 13C NMR (100 MHz, Acetone-d6) δ 93.6, 73.6, 58.7, 48.8, 42.5, 39.5, 32.2, 30.1, 13.1; HRMS (FAB+) calcd for C9H15O3 [(M-H)]+ 171.1021, found 171.1018.
(±)-Scholarein D (4). To a solution of 3 (16.0 mg, 0.093 mmol) in MeOH (5.0 mL) and acetone (0.10 mL), PPTS (1.2 mg, 4.7×10-3 mmol) was added at 0 °C. After 1 h of stirring at 0 °C, saturated aqueous NaHCO3 solution was added and the resulting mixture was extracted three times with EtOAc. The combined organic phases were washed with brine, dried over MgSO4, and evaporated to afford a crude oil. The crude product was purified by flash column chromatography with hexane-EtOAc (1:1 v/v) as an eluent, giving 4 (12.5 mg, 72%) as a colorless oil and 14 (3.1 mg, 18%) as a colorless oil. Data for (±)-scholarein D (4); IR (neat) 3426 cm-1; 1H NMR (400 MHz, CDCl3) δ 4.54 (1H, br s), 4.27-4.22 (1H, m), 3.73 (1H, dddd, J = 11.6, 11.6, 2.8, 0.4 Hz), 3.49 (1H, ddd, J = 11.2, 4.8, 2.8 Hz), 3.38 (3H, s), 2.41-2.33 (1H, m), 2.07 (1H, ddq, J = 12.0, 6.8, 6.8, Hz), 1.86 (1H, ddd, J = 14.4, 6.4, 2.4 Hz), 1.76-1.69 (2H, m), 1.53-1.47 (1H, m), 1.40-1.29 (2H, m), 1.04 (3H, d, J = 6.8 Hz); 13C NMR (100 MHz, CDCl3) δ 99.7, 74.2, 58.4, 54.9, 46.7, 42.0, 38.4, 31.4, 28.9 12.3; HRMS (FAB+) calcd for C10H17O3 [(M-H)]+ 185.1178, found 185.1176. Data for 14; IR (neat) 3434 cm-1; 1H NMR (400 MHz, CDCl3) δ 4.59 (1H, d, J = 4.0 Hz), 4.25-4.21 (1H, m), 3.90 (1H, ddd, J = 11.2, 4.8, 2.8 Hz), 3.49 (1H, ddd, J = 11.2, 11.2, 3.6 Hz), 3.42 (3H, s), 2.33-2.17 (2H, m), 1.96 (1H, ddd, J = 9.6, 8.0, 3.6 Hz), 1.79-1.75 (2H, m), 1.59-1.43 (2H, m), 1.32 (1H, br s) 1.07 (3H, d, J = 6.8 Hz); 13C NMR (100 MHz, CDCl3) δ 102.5, 75.3 63.2, 56.2, 47.5, 41.4, 37.3, 34.2, 28.5, 14.0; HRMS (FAB+) calcd for C9H16O3 [(M-H)]+ 185.1178, found 185.1183.
(±)-Scholarein A (1). To a stirred solution of a mixture of 4 and 14 (7.0 mg, 0.036 mmol) and Et3SiH (11.0 μL, 0.072 mmol) in CH2Cl2 (3.6 mL), BF3・OEt2 (9.0 μL, 0.072 mmol) was added dropwise at –78 °C. After 0.5 h of stirring at –78 °C, saturated aqueous NaHCO3 solution was added. The resulting mixture was allowed to warm to rt with vigorous stirring and then the mixture was extracted three times with CH2Cl2. The combined organic phases were washed with brine, dried over MgSO4, and evaporated to afford a crude oil. The crude product was purified by flash column chromatography with hexane-EtOAc (1:1 v/v) as an eluent, giving 1 (5.3 mg, 94%) as a colorless oil. Data for (±)-scholarein A (1); IR (neat) 3416 cm-1; 1H NMR (400 MHz, CDCl3) δ 4.29-4.25 (1H, m), 3.85-3.80 (2H, m), 3.68 (1H, dd, J = 12.0, 3.6 Hz), 3.33 (1H, ddd, J = 11.6, 11.6, 2.4 Hz), 2.26-2.17 (1H, m), 2.10 (1H, ddq, J = 10.8, 6.8 6.8 Hz), 1.85 (1H, ddd, J = 14.4, 6.4, 3.2 Hz), 1.77 (1H, ddd, J = 14.8, 7.6, 3.2 Hz), 1.64-1.49 (2H, m) 1.43-1.32 (2H, m) 1.01 (3H, d, J = 6.8 Hz); 13C NMR (100 MHz, CDCl3) δ 74.8, 67.4 67.0, 43.3, 41.7, 37.8, 34.0, 30.3, 11.9; HRMS (FAB+) calcd for C9H15O3 [(M-H)]+ 155.1072, found 155.1070.
References
1. G. B. Marini–Bettolo, M. Nocoletti, I. Messana, M. Patania, C. Galeffi, J. U. Oguakwa, G. Portalone, and A. Vaciago, Tetrahedron, 1983, 39, 323. CrossRef
2. T. Feng, X.-H. Cai, Z.-Z. Du, and X.-D. Luo, Helv. Chim. Acta, 2008, 91, 2247. CrossRef
3. Total syntheses: (a) T. V. Lee, J. Toczek, and S. M. Roberts, J. Chem. Soc., Chem. Commun., 1985, 371; CrossRef (b) M.-Y. Chang, C.-Y. Lin, P.-P. Sun, and N.-C. Chang, J. Chin. Chem. Soc., 2007, 54, 239.
4. A. Hibi and M. Toyota, Tetrahedron lett., 2009, 50, 4888. CrossRef
5. (a) M. Saeki and M. Toyota, Heterocycles, 2011, 82, 1705; CrossRef Further applications: (b) M. Saeki and Toyota, Tetrahedron Lett., 2010, 51, 4620; CrossRef (c) K. Takeda and M. Toyota, Tetrahedron Lett., 2011, 52, 5872; CrossRef (d) K. Takeda and M. Toyota, Tetrahedron, 2011, 67, 9909; CrossRef (e) K. Takeda and M. Toyota, Heterocycles, 2012, 84, 1271. CrossRef
6. A. P. Krapcho, Synthesis, 1982, 805; CrossRef and 893. CrossRef
7. G. A. Kraus, K. A. Frazier, B. D. Roth, M. J. Taschner, and K. Neuenschwander, J. Org. Chem., 1981, 46, 2417. CrossRef