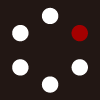
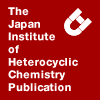
HETEROCYCLES
An International Journal for Reviews and Communications in Heterocyclic ChemistryWeb Edition ISSN: 1881-0942
Published online by The Japan Institute of Heterocyclic Chemistry
e-Journal
Full Text HTML
Received, 30th June, 2012, Accepted, 17th August, 2012, Published online, 28th August, 2012.
DOI: 10.3987/COM-12-S(N)78
■ An Effective Procedure to Promote Aza–Prins Cyclization Reactions Employing a Combination of Ferric Chloride and an Imidazolium Salt in Benzotrifluoride
Chika Osawa, Minami Tateyama, Kensuke Miura, Eiji Tayama, Hajime Iwamoto, and Eietsu Hasegawa*
Department of Chemistry, Faculty of Sciences, Niigata University, Ikarasi 2-8050, Niigata 950-2181, Japan
Abstract
Aza–Prins cyclization reactions of N-tosyl-3-butenylamine with p-methoxybenzaldehyde, 1-naphthaldehyde and 2-naphthaldehyde take place efficiently using a combination of ferric chloride and 1-butyl-3-methylimidazolium hexafluorophosphate in benzotrifluoride (FeIm–BTF procedure). The new methodology, leading to formation of target N-tosyl-4-chloro-2-substituted piperidines, is superior to the one using 1-butyl-3-methylimidazolium tetrachloroferrate. The FeIm–BTF procedure was also applied to aza–Prins cyclization reactions of other aldehydes. Finally, the effects of imidazolium salts on aza–Prins cyclization reaction promoted by boranetrifluoride-diethyl ether complex, leading to formation of fluorinated piperidines, were explored.INTRODUCTION
Piperidines are biologically and pharmaceutically relevant nitrogen heterocycles. As a result, they have attracted interest as synthetic targets.1 The aza–Prins cyclization reaction is among the most useful methods to construct piperidines.2,3 We recently reported that the use of a combination of ferric chloride (FeCl3) and 1-butyl-3-methylimidazolium hexafluorophosphate (BmimPF6) or tetrachloroferrate (BmimFeCl4) in benzotrifluoride (BTF) (named as FeIm–BTF procedure)4 is effective in promoting aza–Prins cyclization reactions.5 The FeIm–BTF procedure has several attractive features, including the employment of a liquid-liquid biphasic reaction mixtures, and the fact that both the imidazolium salts6 and BTF7 are environmentally benign solvents and FeCl3 is an inexpensive and nontoxic iron reagent. Therefore, the FeIm–BTF procedure for carrying out the aza–Prins process is compatible with green sustainable chemistry.8
Application of the FeIm–BTF procedure using BmimFeCl4 to the reaction of N-tosyl-3-butenylamine 1 and aromatic aldehydes 2a–f results in formation of the desired N-tosyl-4-chloro-2-substituted piperidines 3a–f in good to excellent yields with high levels of diastereoselectivity (Scheme 1).5
Observed diastereoselectivity is rationalized by the plausible reaction pathways described in Scheme 2.5 FeCl3 activated aldehyde (2–FeCl3) is attacked by 1 to give the zwitterionic ammonium intermediate. Proton transfer in the ammonium produces the hydroxonium intermediate. Releasing the ferric salt gives the iminium intermediate whose E-form and Z-form are in the equilibrium with each other. Since E-form is more stable than Z-form due to the steric repulsion between Ts and R groups, the formation of the piperidine cation possessing axial R is more favorable than that of the piperidine cation possessing equatorial R, and then in situ generated chloride ion attacks to these piperidine cations from the less crowded upper side. Consequently, t-3 is exclusively formed.
On the other hand, it was also found that p-methoxybenzaldehyde 2g, 1-naphthaldehyde 2h, and 2-naphthaldehyde 2i are much less reactive under these conditions (Scheme 1). In fact, only 8% of t-3i is obtained at 8% conversion of 1, and neither 2g nor 2h undergo the desired reactions. We have undertaken an effort to uncover suitable reaction conditions that would promote the inefficient reactions described above. The results of the studies described below have shown that piperidines 3g–i can be generated by using the FeIm–BTF procedure when BmimFeCl4 is replaced by BmimPF6. The improved procedure was applied to aza–Prins cyclization reactions of other aldehydes including 2f. In this effort, the effects of imidazolium salts on aza–Prins cyclization reaction of 1 with 2a promoted by boranetrifluoride-diethyl ether complex and leading to formation of fluorinated piperidines, were also explored.
RESULTS AND DISCUSSION
Preliminary observations made in this investigation suggest that an increase in the quantity of FeCl3 and a decrease in the volume of BTF leads to increased yields of 3a in the reaction of 1 and 2a using BmimPF6.5 Thus, we decided to conduct the reactions using BmimPF6. An exploratory study was carried out with the aim of identifying possible side products formed in the reaction of 1 and 2a with FeCl3. Although it is known that 2a undergoes a Lewis acid promoted Tishchenko reaction,9 when 2a (0.75 mmol) is treated with FeCl3 (2.0 equiv) and BmimPF6 (1.0 equiv) in BTF (1.0 mL) at room temperature for 24 h, the expected Tishchenko-type ester product is not generated and, instead, only a mixture of unidentifiable substances were produced together with recovered 2a (Scheme 3). On the other hand, treatment of 1 (0.50 mmol) with FeCl3 (3.0 equiv for X = PF6, 1.5 equiv for X = FeCl4) and an imidazolim salt (BmimPF6 or BmimFeCl4, 1.5 equiv) in BTF (1.0 mL) at room temperature for 24 h leads to formation of 4 in yields of 82% for BmimPF6 and 60% (85% conversion of 1) for BmimFeCl4 (Scheme 4). Although not proven, the mechanism for this process likely involves formal electrophilic addition to the alkene moiety of 1 by HCl, generated from water present in the reaction mixture.
Factors that influence the yields of the reaction of 2a with 1, promoted by BmimPF6, were explored next (Table 1). The results show that an increase in the concentration of FeCl3 significantly shortens the reaction time without decreasing the yield of 3a (compare entries 2, 3 with 1, also see Scheme 1). Moreover, the reaction does not occur to completion, the yield of 3a significantly decreases, and 4 (11%) is also produced when BmimPF6 is not included in the reaction mixture (entry 4). An exploration of other solvents for the reaction promoted by FeIm reagent revealed that FeCl3 and BmimPF6, while not being soluble in toluene and cyclohexane, dissolve in CH2Cl2, MeCN, and DMF. Although heterogeneous reactions in the former solvents lead to moderate to good yields of 3a, purification of the product is difficult (entries 5 and 6). Moreover, while the yield of 3a formed by homogeneous reaction in CH2Cl2 is comparable with that in BTF (compare entry 7 with 3), the efficiency of the process in MeCN is low and the reaction does not proceed in DMF (entries 9 and 10). Another notable observation is that 4 (43%) is produced to a considerable extent when BmimPF6 is not present in the reaction conducted in CH2Cl2 (entry 8).
Based on the results described above, we have designed studies aimed at improving FeIm–BTF promoted aza–Prins cyclization reactions (Table 2).10 The observations made in this effort show that when quantity of BTF is decreased by one fifth, product 3 still forms (compare entry 3 with 1, 6 with 5, and 9 with 8, respectively), but when 3.0 equivalents of FeCl3 are used along with BmimFeCl4, 3 is not generated and 4 is formed exclusively (see entries 2 and 10). In contrast, conditions utilizing 3.0 equivalents of FeCl3 with BmimPF6 lead to a significantly accelerated reaction and an increased yield of 3 (entries 4, 7 and 11). While the use of CH2Cl2 is comparable to BTF as solvent for the reaction promoted by BmimPF6 (compare 13 with 11), CH2Cl2 is a better solvent than BTF for the BmimFeCl4 induced process (compare 12 with 9).
The results clearly show that the presence of an imidazolium salt accelerates the reaction (compare entry 14 with 12, also see entries 3, 4, 7, and 8 in Table 1). Although the exact role of the salts is not clear, mixing of FeCl3 with BmimX may cause an ion pair exchange process to occur11 to give another more reactive Fe(III) species (FeCl2X) (Scheme 5). When 1.5 equivalents of BmimPF6 and 3.0 equivalents of FeCl3 are employed to promote reaction of 1.0 equivalents of 1 (see Table 1 and 2), FeCl2PF6 is likely present in the reaction mixture. Thus, it is possible that FeCl2PF6 is a more effective promoter of the aza–Prins cyclization reaction than is FeCl3, which itself leads to a process that forms 4 (Table 1, entries 4 and 8; Table 2, entries 2 and 10).
In order to explore advantageous feature of the FeIm–BTF procedure further (entries 4, 7, and 11 in Table 2), it was applied to the reaction of 2f with 1 (Scheme 6). While the reaction time (5 h) was shorter than that (24 h) in Table 2, this process generates piperidine 3f in 74% yield (t-3f : c-3f = 78 : 22) at 89% conversion of 1, which is greater than that previously reported (48% yield at 62% conversion of 1, see Scheme 1). This procedure was also applied to the reaction of keto-aldehyde 2j (Scheme 7), which was found to form trans-3j in 80% yield while cis-3j could not be isolated. This observation suggests that ketone carbonyl is unaffected under the FeIm–BTF conditions.
In order to determine if imidazolium salts effect other Lewis acid promoted reactions, we studied the fluorinated piperidine forming aza–Prins cyclization reaction promoted by the boranetrifluoride-diethyl ether complex.12,13 Treatment of a mixture of 1 (0.50 mmol) and 2a (1.5 equiv vs 1) with BF3•Et2O (1.5 equiv vs 1) in the presence of an imidazolim salt (BmimX: 1.5 equiv vs 1) in BTF (1.0 mL) at room temperature for 24 h, leads to generation of the fluorinated piperidine 5a as a mixture of diastereoisomers (t-5a : c-5a) in moderate yields, 53% (t-5a : c-5a = 24 : 76) and 54% (t-5a : c-5a = 23 : 77) for the respective BmimPF6 and BmimBF4 induced reactions (Scheme 8).14 Reaction in the absence of BmimX generates 5a but in only 29% yield (t-5a : c-5a = 32 : 68).
It should be noted that the reaction of 1 with 2a promoted by BmimPF6 also affords a small quantity of tetrahydropyridine 6a (6%), which is perhaps produced via deprotonation of a cationic intermediate (Scheme 9).
In order to explore possible derivatization reactions of the piperidines produced in the aza–Prins cyclization reactions, a transformation of 3a was briefly explored. We observed that free radical reaction of t-3a using [(CH3)3Si]3SiH (TTMSS)15 and AIBN at 85 ºC for 24 h proceeds smoothly to give dechlorinated piperidine 7a in 97% (Scheme 10).
In conclusion, the investigation described above has led to the development of the FeIm−BTF procedure for promotion of aza−Prins cyclization reactions of N-tosyl-3-butenylamine with p-methoxybenzaldehyde, 1-naphthaldehyde and 2-naphthaldehyde, processes that do not take place efficiently under the previously devised conditions.5 Significant improvements in the yields of the desired N-tosyl-4-chloro-2-substituted piperidines were achieved. A combination of an imidazolium salt and BTF was also applied to carry out the boranetrifluoride-diethyl ether complex promoted aza–Prins cyclization reaction. Among noteworthy observations made in this effort are that addition of imidazolium salts accelerate the Lewis acid promoted aza–Prins cyclization in both BTF and CH2Cl2, and that BmimPF6 is a more effective additive than BmimFeCl4 in this regard. Although not extensively explored yet, a homolytic cleavage of the carbon-chlorine bond in the aza–Prins cyclization product t-3a via a free radical pathway, suggests an interesting method to form and utilize piperidine radicals as synthetically useful intermediates.
EXPERIMENTAL
NMR spectra were recorded in CDCl3 with Me4Si as an internal standard at 270 MHz, 400 MHz and 700 MHz for 1H-NMR, and 68 MHz, 100 MHz and 176 MHz for 13C-NMR. Benzotrifluoride (BTF, –63.7 ppm) was used as an internal standard for 19F–NMR spectra (376 MHz). Column chromatography was performed with silica gel (Wakogel C-200). Preparative TLC was performed on 20 cm x 20 cm plates coated with silica gel (Wakogel B-5F). FeCl3 and BF3•Et2O were purchased and used for the aza-Prins cyclization reactions. BTF, DMF, c-C6H12, BmimPF6, and BmimFeCl4 were purchased and used without distillation. CH2Cl2 and toluene were treated with H2SO4, water, 5% NaOH, water, CaCl2, and then distilled with CaH2. MeCN was distilled over P2O5 and subsequently distilled with K2CO3. Other reagents and solvents were purchased and used without further purification.
Starting Materials. 1-Phenyl-1,4-butanedione (2j) were prepared by previously reported procedures.16 Other aldehydes 2 were purchased and used without purifications.
Preparation of N-(3’-Buthenyl)phthalimide. 4-Bromo-1-butene (2.23 mL, 22.0 mmol) was added to the suspention of potassium phthalimide (3.71 g, 20.0 mmol) in anhydrous DMF (30 mL). The mixture was stirred under N2 at 80 °C for 20 h. The reaction was quenched by water (30 mL) and extracted with Et2O. The combined organic extracts were washed with water, sat. aqueous Na2S2O3, sat. aqueous NaHCO3 and sat. aqueous NaCl. The Et2O solution was dried over MgSO4, and the filtrated to give crude N-(3’-Butenyl)phthalimide (4.04 g, 20.1 mmol); yellow oil; 1H-NMR (CDCl3, 400 MHz) δ 7.87-7.82 (m, 2H), 7.73-7.68 (m, 2H), 5.86-5.73 (m, 1H), 5.09-5.00 (m, 2H), 3.77 (t, J = 6.4 Hz, 2H), 2.45 (q, J = 6.4, 14.8 Hz, 2H).
Preparation of N-(3’-Butenyl )-4-toluenesulfonamide (1). N-(3’-Buthenyl)phthalimide (4.04 g, 20.1 mmol) was added to 50 mL EtOH to 50 °C. Hydrazine monohydrate (1.94 mL, 40.0 mmol) was then added, and this mixture was stirred under N2 for 1 h. The white suspension was quenched with 2M HCl (20 mL). The resulting solution was stirred for another 10 min. The white solid was filtered off and the remaining solution was concentrated. The residue was added 20% NaOH (12 g) and water (80 mL), the mixture was extracted with CH2Cl2. The combined organic extracts were washed with water (2 times) and sat. aqueous NaCl. The solution was dried over MgSO4, and then the filtrate was concentrated to about 70 mL. To this CH2Cl2 solution containing crude 3-buten-1-amine were added Et3N (8.4 mL, 60.4 mmol) and TsCl (7.63g, 40.0 mmol) at 0 °C. The reaction mixture was allowed to warm to room temperature and stirred under N2 for 24 h. The mixture was quenched with sat. aqueous Na2S2O3 (40 mL) and extracted with Et2O. The combined organic extracts were washed with water, sat. aqueous Na2S2O3 and sat. aqueous NaCl. The ether solution was dried over MgSO4, and concentrated. This crude reaction mixture was purified by silica gel column chromatography (EtOAc/n-hexane = 1/3) and distillation to give 1 (1.63 g, 7.21 mmol, 36%); pale yellow oil; IR (liquid film) 3524, 3052, 1590, 1300, 1138 cm-1; 1H-NMR (CDCl3, 400 MHz) δ 7.75 (d, J = 8.4 Hz, 2H), 7.31 (d, J = 8.6 Hz, 2H), 5.68-5.57 (m, 1H), 5.09-5.01 (m, 2H), 4.51 (brd, 1H), 3.02 (q, J = 6.4 Hz, 2H), 2.43 (s, 3H), 2.20 (q, J = 6.7 Hz, 2H); 13C-NMR (CDCl3, 68 MHz) δ 143.5 (s), 137.0 (s), 134.4 (d), 129.8 (d, 2C), 127.2 (d, 2C), 118.1 (t), 42.4 (t), 33.9 (t), 21.8 (q).
General reaction procedure of aza–Prins cyclization. A BTF solution of 1 (112.7 mg, 0.50 mmol) and 2 (0.75 mmol) was added to Lewis acid (FeCl3: 0.75 or 1.50 mmol, BF3•Et2O: 0.75 mmol) and BmimX (X = FeCl4, PF6, BF4, 0.75 mmol) in BTF under N2. In case a solid aldehyde is not completely dissolved in an appropriate volume of BTF, the aldehyde is placed with FeCl3 and BmimX. This devised operation was used for 2i. The resulting mixture was stirred under N2 at room temperature for 4-24 h. Then, it was extracted with Et2O after addition of water. The extract was treated with water, sat. aqueous Na2S2O3, sat. aqueous NaHCO3, sat. aqueous NaCl, and dried over anhydrous MgSO4. The residue obtained after concentration was purified by silica-gel column chromatography (EtOAc/n-hexane, etc., volume ratio varied), and then subjected to TLC (EtOAc/n-hexane, EtOAc/benzene, etc., volume ratio varied).
The X-ray crystallographic analysis of related piperidines such as trans-N-tosyl-2-benzyl-4- chloropiperidine and trans-N-tosyl-4-chloro-2-isobutylpiperidine is previously reported,3e and characterization of the obtained piperidines 3 and determination of their relative stereochemistry were performed by the comparison of their spectral data with those of the reported piperidines. Thus, characterizations of t-3a, c-3a, t-3b, c-3b, t-3c, c-3c, t-3d, c-3d, t-3e and t-3f were completed and reported in our previous publication.5
trans-N-Tosyl-4-chloro-2-(trans-styryl)piperidine (t-3f)5: pale yellow solid; mp 125.9-129.2 °C; IR (KBr) 2959, 2924, 2862, 1595, 1494, 1447, 1332, 1156, 1089, 936, 726 cm-1; 1H-NMR (CDCl3, 400 MHz) δ 7.67 (d, J = 10.3 Hz, 2H), 7.37-7.14 (m, 7H), 6.42 (d, J = 16.4 Hz, 1H), 5.91 (dd, J = 17.1, 6.9 Hz, 1H), 4.86 (broad s, 1H), 4.12-4.00 (m, 1H), 3.92-3.82 (m, 1H), 3.11 (t, J = 12.6 Hz, 1H), 2.38 (s, 3H), 2.30-2.27 (m, 1H), 2.16-2.09 (m, 1H), 1.98 (dt, J = 12.4, 5.7 Hz, 1H), 1.76 (dq, J = 16.4, 3.4 Hz, 1H) ppm; 13C-NMR (CDCl3, 100 MHz) δ 143.5 (s), 137.1 (s), 135.9 (s), 132.9 (d), 129.7 (d), 128.5 (d), 128.0 (d), 127.3 (d), 126.4 (d), 125.1 (d), 55.5 (d), 53.0 (d), 41.4 (t), 40.6 (t), 35.6 (t), 21.4 (q) ppm; HRMS (ESI) Calcd for C20H2335ClNO2S [M+H]+ = 376.1133, found [M+H]+ = 376.1137; Calcd for C20H2337ClNO2S [M+H]+ = 378.1103, found [M+H]+ = 378.1107.
cis-N-Tosyl-4-chloro-2-(trans-styryl)piperidine (c-3f): white solid; mp 93.5-96.3 °C; IR (KBr) 3429, 3029, 2959, 2920, 2863, 1598, 1493, 1450, 1341, 1296, 1259, 1159, 1092, 1065, 1037, 971, 942, 896, 831, 813, 751, 732, 693, 656, 610 cm-1; 1H-NMR (CDCl3, 400 MHz) δ 7.63 (d, J = 8.4 Hz, 2H), 7.26-7.15 (m, 7H), 6.29-6.27 (m, 2H), 4.46-4.42 (m, 1H), 4.34-4.29 (m, 1H), 3.62-3.56 (m, 1H), 3.53-3.47 (m, 1H), 2.34-2.28 (m, 4H), 2.18-2.08 (m, 2H), 2.01-1.96 (m, 1H) ppm; 13C-NMR (CDCl3, 100 MHz) δ 143.3, 136.5, 136.3, 130.5, 129.4, 128.4, 127.7, 127.7, 127.6, 126.4, 55.9, 54.2, 39.8, 38.9, 33.7, 21.4 ppm; HRMS (ESI) Calcd for C20H2235ClNO2S [M+Na]+ = 398.0952, found [M+Na]+ = 398.0954; Calcd for C20H2237ClNO2S [M+Na]+ = 400.0922, found [M+Na]+ = 400.0920.
trans-N-Tosyl-4-chloro-2-(p-methoxylphenyl)piperidine (t-3g): pale yellow solid; mp 108.5-109.2 °C; IR (KBr) 3049, 2957, 2931, 2871, 2837, 1605, 1511, 1452, 1334, 1249, 1152, 1087, 1033, 954, 929, 844, 730, 658, 612 cm-1; 1H-NMR (CDCl3, 400 MHz) δ 7.76 (d, J = 8.4 Hz, 2H), 7.33 (d, J = 8.0 Hz, 2H), 7.23 (d, J = 8.4 Hz, 2H), 6.87 (d, J = 6.8 Hz, 2H), 5.34 (broad s, 1H), 3.97-3.89 (m, 2H), 3.79 (s, 3H), 3.02 (ddd, J = 14.0, 2.8 Hz, 1H), 2.68 (dd, J = 13.7 Hz 1H), 2.45 (s, 3H), 1.93-1.80 (m, 2H), 1.60-1.49 (m, 1H); 13C-NMR (CDCl3, 100 MHz) δ 158.7, 143.5, 137.9, 129.9, 129.0, 127.7, 126.8, 114.2, 55.5, 55.2, 52.8, 41.2, 37.5, 35.1, 21.5; HRMS(ESI) Calcd for C19H2235ClNO3S [M+Na]+ = 402.0901, found [M+Na]+ = 402.0900; Calcd for C19H2237ClNO3S [M+Na]+ = 404.0872, found [M+H]+ = 404.0864.
cis-N-Tosyl-4-chloro-2-(p-methoxyphenyl)piperidine (c-3g): white solid; mp 126.0-127.8 °C; IR (KBr) 3006, 2920, 2848, 1611, 1514, 1450, 1347, 1302, 1283, 1250, 1160, 1091, 1066, 1036, 866, 818, 735, 721, 654 cm-1; 1H-NMR (CDCl3, 400 MHz) δ 7.50 (d, J = 8.0 Hz, 2H), 7.20 (d, J = 8.4 Hz, 2H), 7.11 (d, J = 8.8 Hz, 2H), 6.73 (d, J = 8.8 Hz, 2H), 4.57 (t, J = 5.8 Hz, 1H), 4.12-4.06 (m, 1H), 3.94-3.88 (m, 1H), 3.78 (s, 3H), 3.41-3.35 (m, 1H), 2.44-2.36 (m, 4H), 2.26-2.20 (m, 1H), 2.17-2.10 (m, 1H), 1.94-1.86 (m, 1H); 13C-NMR (CDCl3, 100 MHz) δ 158.7, 143.1, 136.8, 131.1, 129.4, 128.3, 127.3, 113.3, 57.6, 55.2, 54.2, 41.8, 40.0, 34.1, 21.5; HRMS(ESI); Calcd for C19H2235ClNO3S [M+Na]+ = 402.0901, found [M+Na]+ = 402.0903; Calcd for C19H2237ClNO3S [M+Na]+ = 404.0872, found [M+Na]+ = 404.0869.
trans-N-Tosyl-4-chloro-2-(1’-naphthyl)piperidine (t-3h): pale yellow white turbidness oil; IR (KBr) 3052, 2928, 1597, 1509, 1450, 1398, 1339, 1258, 1157, 1092, 935, 859, 802, 778, 721, 664 cm-1; 1H-NMR (CDCl3, 400 MHz) δ 7.93 (d, J = 8.0 Hz, 1H), 7.77 (d, J = 8.0 Hz, 1H), 7.69 (d, J = 7.6 Hz, 1H), 7.49-7.42 (m, 2H), 7.34-7.25 (m, 4H), 6.96 (d, J = 8.0 Hz, 2H), 5.92 (t, J = 5.2 Hz, 1H), 4.25-4.19 (m, 1H), 4.06-4.00 (m, 1H), 3.90-3.83 (m, 1H), 2.93-2.88 (m, 1H), 2.31-2.20 (m, 5H), 1.99-1.90 (m, 1H); 13C-NMR (CDCl3, 100 MHz) δ 142.8, 136.7, 134.2, 133.9, 130.7, 129.0, 128.9, 128.5, 126.9, 126.3, 125.5, 125.0, 124.6, 123.1, 54.6, 54.0, 43.6, 39.6, 34.8, 21.3; HRMS (ESI); Calcd for C22H2235ClNO2S [M+Na]+ = 422.0952, found [M+Na]+ = 422.0955; Calcd for C22H2237ClNO2S [M+Na]+ = 424.0922, found [M+Na]+ = 424.0919.
cis-N-Tosyl-4-chloro-2-(1’-naphthyl)piperidine (c-3h): yellow oil; IR (KBr) 3048, 2930, 2863, 1597, 1510, 1448, 1397, 1340, 1317, 1260, 1213, 1154, 1092, 1068, 1033, 1007, 944, 823, 800, 779, 728, 711, 657 cm-1; 1H-NMR (CDCl3, 400 MHz) δ 7.83-7.81 (m, 1H), 7.66 (d, J = 8.8 Hz, 2H), 7.50 (d, J = 7.2 Hz, 1H), 7.37-7.33 (m, 3H), 7.03 (d, J = 8.0 Hz, 2H), 6.72 (d, J = 8.0 Hz, 2H), 4.92 (dd, J = 10.6, 3.4 Hz, 1H), 4.42-4.36 (m, 1H), 4.17-4.11 (m, 1H), 3.33 (dt, J = 12.2, 3.2 Hz 1H), 2.86 (dq, J = 13.3, 2.8 Hz, 1H), 2.47-2.41 (m, 1H), 2.38-2.34 (m, 1H), 2.24-2.13 (m, 4H); 13C-NMR (CDCl3, 100 MHz) δ 142.3, 135.8, 133.7, 133.5, 131.1, 128.8, 128.6, 128.4, 127.0, 126.4, 126.0, 125.1, 124.9, 123.2, 58.3, 55.7, 46.4, 40.4, 35.7, 21.2; HRMS (ESI); Calcd for C22H2235ClNO2S [M+Na]+ = 422.0952, found [M+Na]+ = 422.0955; Calcd for C22H2237ClNO2S [M+Na]+ = 424.0922, found [M+Na]+ = 424.0919.
trans-N-Tosyl-4-chloro-2-(2’-naphthyl)piperidine (t-3i)5: white solid; mp 123.5-126.5 °C; IR (KBr) 3442, 3054, 2974, 2929, 2874, 1598, 1504, 1493, 1449, 1338, 1302, 1288, 1261, 1201, 1159, 1122, 1092, 1079, 1044, 1006, 969, 939, 861, 845, 816, 784, 752, 731, 662 cm-1; 1H-NMR (CDCl3, 400 MHz) δ 7.76-7.66 (m, 5H), 7.56 (s, 1H), 7.46-7.34 (m, 3H), 7.26 (d, J = 9.1 Hz, 2H), 5.46 (broad s, 1H), 3.96-3.87 (m, 2H), 3.02(dt, J = 12.8, 2.2 Hz, 1H), 2.81-2.77 (m, 1H), 2.38 (s, 3H), 2.01-1.80 (m, 2H), 1.64-1.44 (m, 2H) ppm; 13C-NMR (CDCl3, 100 MHz) δ 143.6 (s), 138.0 (s), 134.8 (s), 133.2 (s), 132.5 (s), 130.0 (d), 128.9 (d), 127.9 (d), 127.5 (d), 127.0 (d), 126.4 (d), 126.3 (d), 125.5 (d), 124.4 (d), 56.2 (d), 52.8 (d), 41.6 (t), 37.8 (t), 35.2 (t), 21.5 (q) ppm; HRMS (ESI); Calcd for C22H2335ClNO2S [M+H]+ = 400.1133 found, [M+H]+ = 400.1118; Calcd for C22H2337ClNO2S [M+H]+ = 402.1103, found [M+H]+ = 402.1088.
cis-N-Tosyl-4-chloro-2-(2’-naphthyl)piperidine (c-3i): white solid; mp 167.3-169.0 °C; IR (KBr) 3056, 2953, 2860, 1598, 1505, 1448, 1345, 1291, 1161, 1092, 1063, 832, 730, 656 cm-1; 1H-NMR (CDCl3, 400 MHz) δ 7.80-7.76 (m, 1H), 7.70 (d, J = 8.4 Hz, 1H), 7.67-7.63 (m, 1H), 7.53-7.50 (m, 3H), 7.46-7.42 (m, 2H), 7.36 (dd, J = 8.8, 1.6Hz, 1H), 7.12 (d, J = 8.4 Hz, 2H), 4.85 (t, J = 5.8 Hz, 1H), 4.19-4.13 (m, 1H), 4.00-3.93 (m, 1H), 3.55-3.49 (m, 1H), 2.60-2.53 (m, 1H), 2.35-2.30 (m, 4H), 2.20-2.13 (m, 1H), 1.97-1.89 (m, 1H); 13C-NMR (CDCl3, 100 MHz) δ 143.3, 136.8, 136.7, 132.9, 132.5, 129.4, 127.8, 127.6, 127.5, 127.2, 125.9, 125.9, 125.6, 125.1, 57.7, 54.2, 41.4, 39.6, 33.9, 21.4; HRMS(ESI); Calcd for C22H2235ClNO2S [M+Na]+ = 422.0952, found [M+Na]+ = 422.0954; Calcd for C22H2237ClNO2S [M+Na]+ = 424.0922, found [M+Na]+ = 424.0918.
trans-N-Tosyl-4-chloro-2-(3’-phenyl-3’-propione)piperidine (t-3j): brown solid; mp 73.5-78.0 °C; IR (KBr) 3060, 2959, 1683, 1595, 1491, 1448, 1379, 1330, 1208, 1154, 1096, 1033, 991, 911, 842, 814, 749, 692, 650, 605 cm-1; 1H-NMR (CDCl3, 700 MHz) δ 7.93 (d, J = 7.7 Hz, 2H), 7.70 (d, J = 9.8 Hz, 2H), 7.56 (t, J = 7.4 Hz, 1H), 7.46 (t, J = 7.7 Hz, 2H), 7.29-7.26 (m, 2H), 4.22-4.19 (m, 1H), 4.14-4.10 (m, 1H), 3.96 (dd, J = 14.7, 4.2 Hz, 1H), 3.14-3.05 (m, 3H), 2.41 (s, 3H), 2.15-2.09 (m, 1H), 2.00 (ddd, J = 37.5, 13.0, 4.2 Hz, 2H), 1.88-1.83 (m, 1H), 1.63-1.58 (m, 1H), 1.44-1.37 (m, 1H); 13C-NMR (CDCl3, 175 MHz) δ 199.2, 143.5, 138.0, 136.7, 133.1, 129.9, 128.5, 128.0, 126.8, 53.6, 52.8, 40.3, 39.0, 35.1, 35.0, 24.4, 21.5; HRMS(ESI); Calcd for C21H2435ClNO3S [M+Na]+ = 428.1058, found [M+Na]+ = 428.1060; Calcd for C21H2437ClNO3S [M+Na]+ = 430.1028, found [M+Na]+ = 430.1029.
trans-N-Tosyl-4-fluoro-2-phenylpiperidine (t-5a)12a: pale yellow solid; mp 107.9-110.0 °C; IR (KBr) 3055, 2963, 2928, 1596, 1493, 1450, 1346, 1321, 1254, 1154, 1086, 1027, 949, 921, 878, 809, 779, 756, 729, 699, 651 cm-1; 1H-NMR (CDCl3, 400 MHz) δ 7.70 (d, J = 8.4 Hz, 2H), 7.31-7.18 (m, 7H), 5.24 (d, J = 6.4 Hz, 1H), 4.93-4.75 (m, JH,F = 49.2 Hz, 1H), 3.75 (d, J = 14.4 Hz, 1H), 3.39 (dt, J = 13.4, 3.2 Hz, 1H), 2.69-2.61 (m, 1H), 2.41 (s, 3H), 2.07-1.88 (m, J = 46.0 Hz, 1H), 1.77-1.56 (m, 2H) ppm; 13C-NMR (CDCl3, 100 MHz) δ 143.3, 139.4, 137.9, 129.7, 128.2, 127.0, 126.8, 126.5, 126.5, 86.2 (d, J = 171.8 Hz), 53.3, 36.7, 32.1 (d, J = 19.4 Hz), 29.2 (d, J = 21.5 Hz), 21.5 ppm; 19F-NMR (CDCl3, 376 MHz) δ -181.9 ~ -182.4 (m); HRMS (ESI); Calcd for C18H20FNO2S [M+Na]+ = 356.1091, found [M+Na]+ = 356.1093.
cis-N-Tosyl-4-fluoro-2-phenylpiperidine (c-5a)12a: white solid; mp 114.5-119.0 °C; IR (KBr) 3058, 3030, 2973, 2945, 2885, 1596, 1492, 1448, 1340, 1305, 1281, 1249, 1203, 1156, 1092, 1058, 1013, 984, 938, 815, 796, 760, 722, 696, 672 cm-1; 1H-NMR (CDCl3, 400 MHz) δ 7.78 (d, J = 8.4 Hz, 2H), 7.36-7.20 (m, 7H), 5.44 (broad s, 1H), 4.69-4.50 (m, JH,F = 48.7 Hz, 1H), 3.98 (d, J = 14.8 Hz, 1H), 3.03 (t, J = 13.2 Hz, 1H), 2.70-2.64 (m, 1H), 2.46 (s, 3H), 1.87-1.84 (m, 1H), 1.75-1.66 (m, 1H), 1.51-1.39 (m, 1H) ppm; 13C-NMR (CDCl3, 100 MHz) δ 143.5, 137.9, 137.7, 129.9, 128.8, 127.4, 126.9, 126.4, 86.7 (d, J = 172.6 Hz), 55.4 (d, J = 13.4 Hz), 39.9 (d, J = 12.7 Hz), 33.4 (d, J = 18.6 Hz), 31.0 (d, J = 18.6 Hz), 21.5 ppm; 19F-NMR (CDCl3, 376 MHz) δ -175.9~-176.0 (m); HRMS (ESI) Calcd for C18H20FNO2S [M+Na]+ = 356.1091, found [M+Na]+ = 356.1092.
N-Tosyl-2-phenyl-1,2,3,6-tetrahydropyridine (6a)17: white solid; 1H-NMR (CDCl3, 400 MHz) δ 7.69 (d, J = 8.4 Hz, 2H), 7.36 (d, J = 7.2 Hz, 2H), 7.31-7.23 (m, 5H), 5.83-5.75 (m, 1H), 5.62-5.55 (m, 1H), 5.32-5.28 (m, 1H), 4.16-4.07 (m, 1H), 3.39 (d, J = 18.0 Hz, 1H), 2.48-2.34 (m, 5H); 13C-NMR (CDCl3, 100 MHz) δ 143.1, 139.1, 137.7, 129.5, 128.3, 127.4, 127.3, 127.0, 123.9, 123.7, 52.7, 40.8, 26.3, 21.5; HRMS (ESI) Calcd for C18H19NO2S [M+Na]+ = 336.1029, found [M+Na]+ = 336.1027.
Reaction of benzaldehyde (2a) with FeCl3. A BTF solution of 2a (0.75 mmol) was added to FeCl3 (1.50 mmol) and BmimPF6 (0.75 mmol) in BTF under N2. The resulting mixture was stirred under N2 at room temperature for 24 h. Then, it was extracted with Et2O after addition of water. The extract was treated with water, sat. aqueous Na2S2O3, sat. aqueous NaHCO3, sat. aqueous NaCl, and dried over anhydrous MgSO4. The residue obtained after concentration was purified by TLC (EtOAc/benzene = 1/3).
Reaction of N-(3’-Butenyl)-4-toluenesulfonamide (1) with FeCl3. A BTF solution of 1 (112.7 mg, 0.50 mmol) was added to FeCl3 (0.75 or 1.50 mmol) and BmimX (X = FeCl4, PF6, 0.75 mmol) in BTF under N2. The resulting mixture was stirred under N2 at room temperature for 24 h. Then, it was extracted with Et2O after addition of water. The extract was treated with water, sat. aqueous Na2S2O3, sat. aqueous NaHCO3, sat. aqueous NaCl, and dried over anhydrous MgSO4. The residue obtained after concentration was purified by TLC (EtOAc/benzene or EtOAc/n-hexane, volume ratio varied) to give N-(3’-chlorobuthyl)-4-toluenesulfonamide (4): yellow oil; IR 3565, 3281, 2975, 2928, 2872, 2359, 1598, 1495, 1445, 1380, 1325, 1158, 1094, 815, 666, 610, 551 cm-1; 1H-NMR (CDCl3, 400 MHz) δ 7.76 (d, J = 8.4 Hz, 2H), 7.32 (d, J = 8.4 Hz, 2H), 4.98 (broad s, 1H), 4.11-4.03 (m, 1H), 3.13-3.08 (m, 2H), 2.44 (s, 3H), 1.99-1.91 (m, 1H), 1.84-1.75 (m, 1H), 1.48 (d, J = 6.4 Hz, 3H); 13C-NMR (CDCl3, 100 MHz) δ 143.5, 136.6, 129.7, 127.0, 55.5, 40.6, 39.7, 25.3, 21.5; HRMS (ESI) Calcd for C11H1635ClNO2S [M+Na]+ = 284.0482, found [M+H]+ = 284.0486. HRMS (ESI); Calcd for C11H1637ClNO2S [M+Na]+ = 286.0453, found [M+H]+ = 286.0454.
Free radical dechlorination of trans-N-tosyl-4-chloro-2-phenylpiperidine (t-3a): α,α’-Azobisisobutyronitrile (AIBN, 4.9 mg, 0.030 mmol) and tris(trimethylsilyl)silane (TTMSS, 0.14 mL, 0.45 mmol) was added to t-3a (105.0 mg 0.30 mmol) in BTF (3.0 mL). The mixture was heated at 85 °C under N2 for 24 h. The residue obtained after concentration was purified by silica-gel column chromatography (CH2Cl2/n-hexane = 5/1), and then subjected to TLC (CH2Cl2/n-hexane = 5/1) to give N-tosyl-2-phenylpiperidine (7a)18: pale yellow solid; mp 128.8-131.6 °C; IR (KBr) 3049, 2953, 2926, 2865, 1596, 1493, 1449, 1373, 1327, 1297, 1220, 1151, 1099, 1049, 1026, 947, 817, 756, 722, 662, 631 cm-1; 1H-NMR (CDCl3, 400 MHz) δ 7.76 (d, J = 7.7 Hz, 2H), 7.36-7.21 (m, 7H), 5.27 (broad s, 1H), 3.84 (d, J = 14.1 Hz, 1H), 3.01 (dt, J = 13.5, 3.0 Hz, 1H), 2.43 (s, 3H), 2.21 (d, J = 13.4 Hz, 1H), 1.68-1.60 (m, 1H), 1.52-1.25 (m, 4H); 13C-NMR (CDCl3, 175 MHz) δ 142.9, 138.8, 138.6, 129.6, 128.5, 126.9, 126.9, 126.7, 55.2, 41.8, 27.1, 24.2, 21.5, 18.9; HRMS(ESI); Calcd for C18H21NO2S [M+Na]+ = 338.1185, found [M+Na]+ = 338.1186.
ACKNOWLEDGEMENTS
This work was partly supported by the grant from the Uchida Energy Science Promotion Foundation.
References
1. General reviews of piperidines: (a) M. G. P. Buffat, Tetrahedron, 2004, 60, 1701; CrossRef (b) G. J. Brizgys, H. H. Jung, and P. E. Floreancig, Chem. Sci., 2012, 3, 438. CrossRef
2. Reviews of aza–Prins cyclization reaction: (a) W. N. Speckamp and H. Hiemstra, Tetrahedron 1985, 41, 4367; CrossRef (b) C. Olier, M. Kaafarani, S. Gastaldi, and M. P. Bertrand, Tetrahedron, 2010, 66, 413. CrossRef
3. For representative examples of aza–Prins cyclization reaction: (a) A. P. Dobbs, S. J. J. Guesne, M. B. Hursthouse, and S. J. Coles, Synlett, 2003, 1740; CrossRef (b) A. P. Dobbs, S. J. J. Guesne, S. Martinove, S. J. Coles, and M. B. Hursthouse, J. Org. Chem., 2003, 68, 7880; CrossRef (c) S. Hanessian, M. Tremblay, and J. F. W. Petersen, J. Am. Chem. Soc., 2004, 126, 6064; CrossRef (d) A. P. Dobbs and S. J. Guesne, Synlett, 2005, 13, 2101; CrossRef (e) R. M. Carballo, M. A. Ramırez, M. L. Rodrıguez, V. S. Martın, and J. I. Padron, Org. Lett., 2006, 8, 3837; CrossRef (f) M. S. R. Murty, K. R. Ram, and J. S. Yadav, Tetrahedron Lett., 2008, 49, 1141; CrossRef (g) J. S. Yadav, B. V. S. Reddy, D. N. Chaya, G. G. K. S. Narayana Kumar, S. Aravind, A. C. Kunwar, and C. Madavi, Tetrahedron Lett., 2008, 49, 3330; CrossRef (h) P. O. Miranda, R. M. Carballo, V. S. Martın, and J. I. Padron, Org. Lett., 2009, 11, 357; CrossRef (i) J. S. Yadav, B. V. Subba Reddy, D. N. Chaya, G. G. K. S. Narayana Kumar, P. Naresh, and B. Jagadeesh, Tetrahedron Lett., 2009, 50, 1799; CrossRef (j) A. D. Dobbs, S. J. J. Guesne, R. J. Parker, J. Skidmore, R. A. Stephenson, and M. B. Hursthouse, Org. Biomol. Chem., 2010, 8, 1064; CrossRef (k) J. S. Yadav, P. Borkar, P. P. Chakravarthy, B. V. Subba Reddy, A. V. S. Sarma, S. J. Basha, B. Sridhar, and R. Gree, J. Org. Chem., 2010, 75, 2081; CrossRef (l) R. M. Carballo, G. Valdomir, M. Purino, V. S. Martin, and J. I. Padron, Eur. J. Org. Chem., 2010, 8, 2304; CrossRef (m) G. Sabitha, S. K. Das, R. Srinivas, and J. S. Yadav, Helv. Chim. Acta, 2010, 93, 2023; CrossRef (n) B. V. Subba Reddy, K. Ramesh, A. V. Ganesh, G. G. K. S. Narayana Kumar, J. S. Yadav, and R. Grée, Tetrahedron Lett., 2011, 52, 495; CrossRef (o) B. V. Subba Reddy, Prashant Borkar, J. S. Yadav, B. Sridhar, and R. Grée, J. Org. Chem., 2011, 76, 7677. CrossRef
4. H. Tsuchida and E. Hasegawa, Tetrahedron, 2010, 66, 3447. CrossRef
5. E. Hasegawa, N. Hiroi, C. Osawa, E. Tayama, and H. Iwamoto, Tetrahedron Lett., 2010, 51, 6535.. CrossRef
6. (a) T. Welton, Chem. Rev., 1999, 99, 2071; CrossRef (b) P. Wasserscheid and W. Keim, Angew. Chem. Int. Ed., 2000, 39, 3772; CrossRef (c) T. Itoh, Y. Nishimura, M. Kashiwagi, and M. Onaka, In Ionic Liquids As Green Solvents, ed. by R. D. Rogers and K. R. Seddon, Oxford University Press USA: New York, NY, ACS Symposium Series, 2003, Vol. 856, Chapter 21, pp. 251; (d) T. Fuchigami and T. Tajima, J. Fluorine Chem., 2005, 126, 181; CrossRef (e) H. Hagiwara, In Ionic Liquids in Organic Synthesis, ed. by S. V. Malhotra, Oxford University Press USA: New York, NY, ACS Symposium Series, 2007, Vol. 950, Chapter 11, pp. 127; (f) V. I. Pârvulescu and C. Hardacre, Chem. Rev., 2007, 107, 2615; CrossRef (g) F. Van Rantwijik and R. A. Sheldon, Chem. Rev., 2007, 107, 2757; CrossRef (h) M. A. P. Martins, C. P. Frizzo, D. N. Moreira, N. Zanatta, and H. G. Bonacorso, Chem Rev., 2008, 108, 2015; CrossRef (i) J. Pavlinac, M. Zupan, K. K. Laali, and S. Stavber, Tetrahedron, 2009, 65, 5625; CrossRef (j) R. Giernoth, Angew. Chem. Int. Ed., 2010, 49, 2834. CrossRef
7. (a) A. Ogawa and D. P. Curran, J. Org. Chem., 1997, 62, 450; CrossRef (b) J. J. Maul, P. J. Ostrowski, G. A. Ublacker, B. Linclau, and D. P. Curran, Modern Solvents in Organic Synthesis, In P. Knochel, Ed., Springer: Berlin, 1999, pp. 79; (c) Y. Nakamura, S. Takeuchi, K. Okumura, Y. Ohgo, and D. P. Curran, Tetrahedron, 2002, 58, 3963; CrossRef (d) H. Jona, H. Mandai, W. Chavasiri, K. Takeuchi, and T. Mukaiyama, Bull. Chem. Soc. Jpn., 2002, 75, 291; CrossRef (e) S. G. Nelson, C. Zhu, and X. Shen, J. Am. Chem. Soc., 2004, 126, 14; CrossRef (f) K. Mikami, M. N. Islam, M. Yamanaka, Y. Itoh, M. Shindo, and K. Kudo, Tetrahedron Lett., 2004, 45, 3681; CrossRef (g) D. Crich and Y. Zou, Org. Lett., 2004, 6, 775; CrossRef (h) D. Crich and Y. Zou, J. Org. Chem., 2005, 70, 3309; CrossRef (i) H. Yorimitsu, Y. Murakami, H. Takamatsu, S. Nishimura, and E. Nakamaura, Angew. Chem. Int. Ed., 2005, 44, 2708; CrossRef (j) M. Zhang, C. Chen, W. Ma, and J. Zhao, Angew. Chem. Int. Ed., 2008, 47, 9730; CrossRef (k) E. Hasegawa, Y. Ogawa, K. Kakinuma, H. Tsuchida, E. Tosaka, S. Takizawa, H. Muraoka, and T. Saikawa, Tetrahedron, 2008, 64, 7724; CrossRef (l) D. Suarez, G. Laval, S. M. Tu, D. Jiang, C. L. Robinson, R. Scott, and B. T. Golding, Synthesis, 2009, 1807. CrossRef
8. (a) P. T. Anastas and J. C. Warner, “Green Chemistry: Theory and Practice”, Oxford University Press: Oxford, 1998; (b) Green Chemistry: I. T. Horvath and P. T. Anastas, Chem. Rev., 2007, 107, 2169. CrossRef
9. M. M. Mojtahedi, E. Akbarzadeh, R. Sharifi, and M. S. Abaee, Org. Lett., 2007, 9, 2791. CrossRef
10. No significant trans-diastereoselectivity was observed in 3h. In the reaction of 2h with 1, relatively bulky 1-naphthyl substituent would destabilize the piperidine cation intermediate possessing axial R in Scheme 2.
11. J. W. Lee, J. Y. Shin, Y. S. Chun, H. B. Jang, C. E. Song, and S. G. Lee, Acc. Chem. Res., 2010, 43, 985. CrossRef
12. Examples of aza–Prins cyclization synthesizing fluorinated piperidines: (a) Y. Kishi, H. Nagura, S. Inagi, and T. Fuchigami, Chem. Commun., 2008, 3876; CrossRef (b)Y. Kishi, S. Inagi, and T. Fuchigami, Eur. J. Org. Chem., 2009, 103; CrossRef (c) J. S. Yadav, B. V. Subba Reddy, D. N. Chaya, K. Ramesh, G. G. K. S. Narayana Kumar, and R. Gree, Tetrahedron Lett., 2010, 51, 1578. CrossRef
13. Although boranetrifluoride-diethyl ether complex has been previously applied to aza–Prins cyclization reaction, it was not used for fluorination but for arylation: J. S. Yadav, B. V. Subba Reddy, K. Ramesh, G. G. K. S. Narayana Kumar, and R. Gree, Tetrahedron Lett., 2010, 51, 818. CrossRef
14. Previous investigations of aza–Prins cyclization reactions giving fluorinated piperidines demonstrate a characteristic diastereoselectivity in which cis-diastereoisomers become predominat.12 However, no clear rationalization for the reverse diastereoselectivity between chlorinated and fluorinated piperidines has been made.
15. (a) C. Chatgilialoglu, Acc. Chem. Res., 1992, 25, 188; CrossRef (b) C. Chatgilialoglu, Chem. Rev., 1995, 95, 1229; CrossRef (c) C. Chatgilialoglu and J. Lalevée, Molecules, 2012, 17, 527. CrossRef
16. C. Uyeda and E. N. Jacobsen, J. Am. Chem. Soc., 2008, 130, 9228. CrossRef
17. C. Wang and J. A. Tunge, Org. Lett., 2006, 8, 3211. CrossRef
18. B. Schlummer and J. F. Hartwig, Org. Lett., 2002, 4, 1471. CrossRef