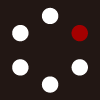
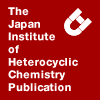
HETEROCYCLES
An International Journal for Reviews and Communications in Heterocyclic ChemistryWeb Edition ISSN: 1881-0942
Published online by The Japan Institute of Heterocyclic Chemistry
e-Journal
Full Text HTML
Received, 29th June, 2012, Accepted, 6th September, 2012, Published online, 19th September, 2012.
DOI: 10.3987/COM-12-S(N)71
■ Selective Cross-Dehydrogenative Coupling of N-Phenyltetrahydroisoquinolines in Aqueous Media Using Poly(Aniline Sulfonic Acid)/Gold Nanoparticles
Toru Amaya, Tsubasa Ito, and Toshikazu Hirao*
Department of Applied Chemistry, Graduate School of Engineering, Osaka University, 2-1 Yamadaoka, Suita, Osaka 560-0871, Japan
Abstract
Selective cross-dehydrogenative coupling of N-phenyltetrahydroisoquinoline with carbon nucleophiles such as dimethyl malonate, ethyl acetoacetate, and nitromethane was demonstrated in complete aqueous media using poly(2-methoxyaniline-5-sulfonic acid)/gold nanoparticles under molecular oxygen.Catalytic oxidative C–H bond functionalizations, particularly C–C bond forming reactions, have attracted significant interest.1 Among them, catalytic cross-dehydrogenative coupling of tert-amines such as N-phenyltetrahydroisoquinoline (1) with carbon nucleophiles has received much attention.2 Concerning about the terminal oxidant, molecular oxygen is favorable due to the following reasons: available from atmosphere, low cost, easy separation, low toxic, ecological, safer than peroxides, and so on. Some transition metals such as Cu catalyze this reaction effectively under molecular oxygen.3 Recently, it was revealed that gold nanoparticles (Au NPs) exhibit efficient catalytic activity.4 This reaction is considered to involve the oxidation of tert-amine 1 to produce the iminium cation species 2, and the subsequent nucleophilic addition, giving the corresponding product. The byproducts of this reaction are just water and/or hydrogen peroxide when molecular oxygen is employed as a terminal oxidant. Therefore, this reaction is considered to be atom economic. On the other hand, organic reactions in aqueous media are required for sustainable chemistry.5 From this point of view, the difficulty of this cross-dehydrogenative coupling is much raised because water can attack to the iminium cationic intermediate 2 and the further oxidation of 3 leads to the lactam 4 (Scheme 1). In fact, only a few examples have achieved the selective coupling reaction in water.3b,g,j So far, we have focused on the aerobic oxidation reactions in aqueous media using a hybrid catalyst consisting of Au NPs and water-soluble polyaniline, poly(2-methoxyaniline-5-sulfonic acid) (PMAS).6-8 The hybrid was revealed to catalyze aerobic oxidation of alcohols and 2-substituted indolines in water, where PMAS works as both a stabilizer of Au NPs and a redox mediator.7,8 Herein, we report the selective cross-dehydrogenative coupling of 1 with carbon nucleophiles such as dimethyl malonate (5), ethyl acetoacetate, and nitromethane in complete aqueous media using PMAS/Au NPs under molecular oxygen (Scheme 1).
PMAS/Au NPs were prepared by treatment of PMAS with N2H4·H2O, followed by addition of NaAuCl4·2H2O.9 The average diameter of particles was 9.6 nm. The thus-obtained aqueous solution of the PMAS/Au NPs was used for the catalytic reaction.
Cross-dehydrogenative coupling of N-phenyltetrahydroisoquinoline (1) with two molar equivalents of dimethyl malonate (5) was conducted in an aqueous solution under oxygen atmosphere, where no organic solvent was used (Table 1). First, the reaction was performed in the presence of 5 mol% PMAS/Au NPs (based on Au atom) at 80 oC for 12 h. The desired cross-coupling product 6 was prepared in a low yield (23%). The lactam 4 was mainly obtained in 72% yield with the further dehydrogenated product 7 (1%) (entry 1). In the absence of 5, the lactam 4 was obtained in 95% (entry 2). The reaction at 50 oC for 24 h gave 6 in 82% yield together with 7% of 4 (entry 3).10,11 Use of one molar equivalent of 5 under the conditions for entry 3 lowered the yield of 6 (entry 4). The selectivity increased at room temperature although the reaction was too slow as shown in entry 5. The iminium cation species 2 and its water adduct 3 are likely to be in equilibrium under these reaction conditions. The water adduct 3 seems to be oxidized slowly at a low temperature. Therefore, the desired cross-coupling reaction proceeded well to give 6 as a main product. On the other hand, PMAS itself showed the less catalytic activity in the absence of Au NPs (entry 6). Poly(N-vinyl-2-pyrrolidone) (PVP)/Au NPs were used as a representative catalyst, in which PVP does not have a redox-active function (the average diameter: 9.4 nm). The yield was lower as compared with PMAS/Au NPs whereas an average particles’ size is similar (entry 7), indicating that the reaction was enhanced by the presence of PMAS. Redox mediating effect of PMAS is suggested to be operative to account for the results as observed with the oxidation of alcohols and 2-substituted indolines.7,8
Table 2 shows some examples for the cross-dehydrogenative coupling. Diethyl malonate worked well as a nucleophile in this kind of transformation (entry 1). Instead of the malonate, ethyl acetoacetate similarly reacted to produce the corresponding cross-coupling product 9 (entry 2). Nitromethane selectively coupled to give the desired product 10 in 91% yield together with 4% yield of 4 (entry 3). Methoxy group in the N-aryl moiety resulted in a good yield of 11 (entry 4).
In conclusion, we demonstrated the selective cross-dehydrogenative coupling of N-aryltetrahydroisoquinoline with some carbon nucleophiles such as dimethyl malonate (5), ethyl acetoacetate, and nitromethane in complete aqueous media using the PMAS/Au NPs under molecular oxygen. PMAS is thought to work as a redox mediator in this reaction. Further investigation of this catalytic system is now underway.
ACKNOWLEDGEMENTS
This work was partially supported by a Grant-in-Aid for Scientific Research on Innovative Areas “Advanced Molecular Transformations by Organocatalysts” from The Ministry of Education, Culture, Sports, Science and Technology, Japan.
References
1. (a) K. R. Campos, Chem. Soc. Rev., 2007, 36, 1069; CrossRef (b) C.-J. Li, Acc. Chem. Res., 2009, 42, 335; CrossRef (c) C. J. Scheuermann, Chem. Asian J., 2010, 5, 436; CrossRef (d) C. S. Yeung and V. M. Dong, Chem. Rev., 2011, 111, 1215; CrossRef (e) M. Klussmann and D. Sureshkumar, Synthesis, 2011, 353. CrossRef
2. E. Boess, C. Schmitz, and M. Klussmann, J. Am. Chem. Soc., 2012, 134, 5317, and references therein. CrossRef
3. (a) S. Murahashi, N. Komiya, H. Terai, and T. Nakae, J. Am. Chem. Soc., 2003, 125, 15312; CrossRef (b) O. Baslé and C.-J. Li, Green Chem., 2007, 9, 1047; CrossRef (c) O. Baslé and C.-J. Li, Org. Lett., 2008, 10, 3661; CrossRef (d) Y. Shen, M. Li, S. Wang, T. Zhan, Z. Tan, and C.-C. Guo, Chem. Commun., 2009, 953; CrossRef (e) S. Singhal, S. L. Jain, and B. Sain, Chem. Commun., 2009, 2371; CrossRef (f) A. Yu, Z. Gu, D. Chen, W. He, P. Tan, and J. Xiang, Catalysis Communications, 2009, 11, 162; CrossRef (g) T. Zeng, G. Song, A. Moores, and C.-J. Li, Synlett, 2010, 2002; CrossRef (h) A. G. Condie, J. C. González-Gómez, and C. R. J. Stephenson, J. Am. Chem. Soc., 2010, 132, 1464; CrossRef (i) E. Boess, D. Sureshkumar, A. Sud, C. Wirtz, C. Farès, and M. Klussmann, J. Am. Chem. Soc., 2011, 133, 8106; CrossRef (j) K. Alagiri, G. S. R. Kumara, and K. R. Prabhu, Chem. Commun., 2011, 47, 11787; CrossRef (k) M. Rueping, C. Vila, R. M. Koenigs, K. Poscharny, and D. C. Fabry, Chem. Commun., 2011, 47, 2360; CrossRef (l) Y. Pan, C. W. Kee, L. Chen, and C.-H. Tan, Green Chem., 2011, 13, 2682; CrossRef (m) Y. Pan, S. Wang, C. W. Kee, E. Dubuisson, Y. Yang, K. P. Loha, and C.-H. Tan, Green Chem., 2011, 13, 3341; CrossRef (n) W.-P. To, G. S.-M. Tong, W. Lu, C. Ma, J. Liu, A. L.-F. Chow, and C.-M. Che, Angew. Chem. Int. Ed., 2012, 51, 2654; CrossRef (o) J. Xie, H. Li, J. Zhou, Y. Cheng, and C. Zhu, Angew. Chem. Int. Ed., 2012, 51, 1252; CrossRef (p) an oxidant free coupling reaction is reported, see X.-Z. Shu, Y.-F. Yang, X.-F. Xia, K.-G. Ji, X.-Y. Liu, and Y.-M. Liang, Org. Biomol. Chem., 2010, 8, 4077. CrossRef
4. M.-H. So, Y. Liu, C.-M. Ho, and C.-M. Che, Chem. Asian J., 2009, 4, 1551. CrossRef
5. (a) C.-J. Li and L. Chen, Chem. Soc. Rev., 2006, 35, 68; CrossRef (b) A. Chanda and V. V. Fokin, Chem. Rev., 2009, 109, 725; CrossRef (c) R. N. Butler and A. G. Coyne, Chem. Rev., 2010, 110, 6302. CrossRef
6. S. Shimizu, T. Saitoh, M. Uzawa, M. Yuasa, K. Yano, T. Maruyama, and K. Watanabe, Synth. Met., 1997, 85, 1337. CrossRef
7. D. Saio, T. Amaya, and T. Hirao, Adv. Synth. Catal., 2010, 352, 2177. CrossRef
8. T. Amaya, T. Ito, Y. Inada, D. Saio, and T. Hirao, Tetrahedron Lett., 2012, 53, 6144. CrossRef
9. The TEM image is shown in reference 8.
10. A general procedure for the catalytic aerobic dehydrogenative oxidation: PMAS (kindly provided by Mitsubishi Rayon Co.) was deionised through cation-exchange resins before use. Other reagents were used as recieved. The water used in the present study is of a milliQ grade. An aqueous solution (1 mL) of PMAS (0.06 mmol based on the aniline monomer unit, 12 mg) was mixed with 3 mL of 0.5 M B(OH)3-NaOH (pH 9.0) aqueous solution. An aqueous solution (1 mL) of N2H4·H2O (0.095 mmol, 4.7 μL) was added to the PMAS solution, which was stirred at room temperature under air for 3.5 h. Then, an aqueous solution (1 mL) of NaAuCl4·2H2O (0.06 mmol, 24 mg) was added to the stirred solution at 0 °C. The mixture was stirred at room temperature under air for 24 h. UV-vis-NIR spectrum, XPS analysis, and TEM picture of the thus-obtained PMAS/Au NPs solution are described in the reference 8. A two-necked flask was evacuated and backfilled with molecular oxygen. Then, N-phenyltetrahydroisoquinoline (1, 41.9 mg, 0.2 mmol), dimethyl malonate (5, 45.7 μL, 0.4 mmol) and 1 mL of the PMAS/Au NPs solution (Au: 0.01 mmol, 5 mol%) were added at room temperature. The mixture was stirred at 50 ˚C under oxygen atmosphere for 24 h. The reaction mixture was extracted with EtOAc. The organic layer was evaporated and examined by 1H NMR analysis (JEOL ECS-400, 400 MHz) with 1,3,5-trimethoxybenzene as an internal standard.
11. Use of the recycled catalyst gave 6 in 73% yield, where the addition of base (NaOH, 0.2 mmol) was required.