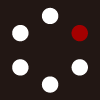
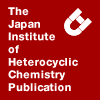
HETEROCYCLES
An International Journal for Reviews and Communications in Heterocyclic ChemistryWeb Edition ISSN: 1881-0942
Published online by The Japan Institute of Heterocyclic Chemistry
e-Journal
Full Text HTML
Received, 12th July, 2012, Accepted, 24th August, 2012, Published online, 28th August, 2012.
DOI: 10.3987/COM-12-S(N)98
■ RAPID ASSEMBLY OF POLYCYCLIC STRUCTURES VIA SIGMATROPIC REARRANGEMENT OF S-PROPARGYL XANTHATES
Ryan M. Harrington, David Wright, and Samir Z. Zard*
Department of Chemistry, Ecole Polytechnique, CNRS UMR 7652, 91128 Palaiseau, France
Abstract
Upon heating in chlorobenzene, propargyl xanthates yield the corresponding 4,5-bis(alkylidene)-1,3-dithiolan-2-ones which are trapped by unactivated olefins in an intramolecular Diels-Alder cycloaddition yielding interesting polycyclic structures.We have found over the years that radicals derived from xanthates provide a rich and varied chemistry for the synthesis of a wide array of molecular architectures.1 In the course of our studies we discovered an unusual non-radical chemistry of S-propargyl xanthates 1. We observed that, upon heating, S-propargyl xanthates undergo a [3,3]-sigmatropic rearrangement to give the corresponding allene 2, which exists in equilibrium with a novel betaine of structure 3 (Scheme 1).2 The equilibrium is almost completely in favor of allene 2, and evidence for the existence of betaine 3 is indirect and based on chemical transformations that cannot be rationalized otherwise.3 These transformations hinge for most part on exploiting the allylic anion character of this species (only two canonical structures 3a and 3b are drawn for clarity).
One such process concerns S-propargyl xanthates of general structure 4, which are converted into 4,5-bis(alkylidene)-1,3-dithiolan-2-ones 6 via the corresponding betaines 5 (Scheme 2).4 These highly reactive rigid cisoid dienes, and especially the parent compound (6a, R = H), readily dimerize into derivatives 7, but can be captured by strongly electrophilic dienophiles as illustrated by cycloadditions with dimethyl acetylenedicarboxylate or naphthoquinone to furnish cycloadducts 8 and 9. The latter is easily oxidized by air to give brown-colored tetracyclic derivative 10 in high overall yield (Scheme 2).4 This method for producing 4,5-bis(alkylidene)-1,3-dithiolan-2-ones 6 was later applied by Gorgues et al. for functionalizing fullerenes.5
In this communication, we report our preliminary results on the generation and capture of the 4,5-bis(alkylidene)-1,3-dithiolan-2-ones 6 with unactivated alkenes. Our attempts involving intermolecular cycloadditions were unfortunately met with failure. Even starting with xanthate 4a, which gives the most reactive parent diene 6a, and using neat refluxing allyl acetate or propargyl acetate as the reaction medium did not result in the isolation of any discernable cycloaddition products 10 (Scheme 3). Clearly, the desired intermolecular cycloaddition with ordinary, unactivated alkenes was unable to compete with the self-reaction of the diene.
More promising were the intramolecular variants. The required precursors were readily prepared by exploiting the possibility of generating the alkynyllithium from propargyl chloride and capturing it with various appropriately substituted aldehydes. A first series of diene precursors 13a-j was prepared from modified salicylaldehydes 11a-j by a simple two-step sequence, as pictured in Scheme 4 (the yields given are overall yields for the two steps). While it is possible to isolate the intermediate chlorides 12, these substances readily decompose and it is best to transform them directly without purification into the corresponding propargyl xanthates 13, which are much more bench stable.
Upon heating in refluxing chlorobenzene, S-propargyl xanthates 13a-j underwent the aforementioned rearrangement to yield intermediary 4,5-bis(alkylidene)-1,3-dithiolan-2-ones 14, which, except for 13j, were trapped by Diels-Alder cycloaddition to yield new 1,3-dithiol-2-ones 15a-i containing carbon skeletons with the formation of three new carbocycles (Scheme 5). As expected for a concerted suprafacial cycloaddition process, the two isomeric chlorides 13c and 13d furnished the corresponding epimeric cycloadducts 15c and 15d. In the case of S-propargyl xanthates 13b, a slight loss of stereochemical integrity was observed, perhaps due to partial isomerization of the butenyl group by adventitious acid. Adduct 15g containing a tertiary bromide was only moderately stable under the reaction conditions and tended to undergo loss of hydrogen bromide to give olefinic side-products. The acetylenic precursor 13i furnished diene adduct 15i, which spontaneously aromatized upon standing in air. Partial aromatization also occurred during the reaction, causing some variability in the yield. Finally, we were disappointed to find that 13j did not lead to the seven-membered ring cycloadduct 15j. Recourse to high dilution or to slow addition of S-propargyl xanthate 13j to refluxing chlorobenzene did not modify the outcome. This reflected the difficult competition between the desired intramolecular cycloaddition and the unwanted dimerization of intermediate diene 14. The importance of pre-organization in speeding up intramolecular Diels-Alder reactions has been noted very recently by Krenske and Houk.6 The longer tether in 13j is clearly too floppy to ensure the necessary favorable pre-organization speeding access to the transition state.
Although the salicylaldehyde scaffold was convenient for the purposes of exploring this chemistry on unactivated olefins, this protocol can be applied in principle to any system in which the propargyl xanthate is appropriately spaced from the dienophile. Indeed, the approach is not limited to scaffolds containing a rigid aryl backbone at all, but may be applied to systems containing varied aliphatic and hetero-aliphatic skeletons. Thus, propargyl xanthates 16a-e shown in Scheme 6 react under similar conditions to yield tetrahydrofuran, tetrahydropyran, pyrrolidine, and piperidine heterocyclic structures 17a-e in good yields.
The Diels-Alder reaction is arguably one of the most powerful reactions for organic synthesis.7 The observation that S-propargyl xanthates, of general structure 4, represent latent, reactive cisoid dienes opens therefore numerous possibilities for the synthesis of useful compounds. For instance, the examples of Diels-Alder products displayed in Scheme 4 have chromene-like structures.8 Chromene subunits are present in several classes of bioactive compounds and are found in numerous plants and edible vegetables.9 Synthetic analogues are known to be pharmacologically important, having anti-fungal and anti-microbial activities.10 Furthermore, the 1,3-dithiolen-2-one motifs in the cycloadducts are interesting in their own right, as they furnish tetrathiafulvenes upon reductive coupling with phosphines or phosphites.11 Tetrathiafulvenes are known for their charge transfer properties and have found applications in non-linear optics and as photovoltaics.12 A variety of derivatives are also used as redox switches and near-IR dyes.13 Furthermore, base induced cleavage yields a 1,2-dithiol moiety, a key bidentate ligand in many biological molybdenum and tungsten complexes such as the molybdopterin cofactor.14 Many transition metals may be sequestered by the dithiol ligand and such complexes are being studied for applications in photographic, lithographic, thermal imaging, and liquid crystal technologies.15
ACKNOWLEDGEMENTS
One of us (R. M. H.) thanks Ecole Polytechnique for a scholarship.
References
1. For reviews of the xanthate transfer, see: S. Z. Zard, Angew. Chem., Int. Ed. Engl., 1997, 36, 672; CrossRef B. Quiclet-Sire and S. Z. Zard, Top. Cur. Chem., 2006, 264, 201; CrossRef B. Quiclet-Sire and S. Z. Zard, Chem. Eur. J., 2006, 12, 6002; CrossRef S. Z. Zard, Aust. J. Chem., 2006, 59, 663; CrossRef B. Quiclet-Sire and S. Z. Zard, Pure Appl. Chem., 2011, 83, 519; CrossRef B. Quiclet-Sire and S. Z. Zard, Chimia, 2012, 66, 404.
2. J. Boivin, C. Tailhan, and S. Z. Zard, J. Am. Chem. Soc., 1991, 113, 5874. CrossRef
3. J. Boivin, E. B. Henriet, C. Tailhan, and S. Z. Zard, Tetrahedron Lett., 1993, 34, 2763; CrossRef J. Boivin, E. B. Henriet, and S. Z. Zard, J. Am. Chem. Soc., 1994, 116, 9739; CrossRef J. Boivin, E. B. Henriet, and S. Z. Zard, Tetrahedron Lett., 1995, 36, 5171; CrossRef M. Tromeur-Fauré and S. Z. Zard, Tetrahedron Lett., 1998, 39, 7301; CrossRef M. Tromeur-Fauré and S. Z. Zard, Tetrahedron Lett., 1999, 40, 1305. CrossRef
4. J. Boivin, C. Tailhan, and S. Z. Zard, Tetrahedron Lett., 1992, 33, 7853. CrossRef
5. C. Coulle, M. Cariou, M. Bainville, A. Gorgues, P. Hudhomme, J. Orduna, and J. Garin, Tetrahedron Lett., 1997, 38, 81. CrossRef
6. E. H. Krenske, E. W. Perry, S. V. Jerome, T. J. Maimone, P. S. Baran, and K. N. Houk, Org. Lett., 2012, 14, 3016. CrossRef
7. For reviews on the Diels-Alder reaction, see: K. Takao, R. Munakata, and K. Tadano, Chem. Rev., 2005, 105, 4779; CrossRef K. C. Nicolaou, S. A. Snyder, T. Montagnon, and G. Vassilikogiannakis, Angew. Chem. Int. Ed., 2002, 41, 1668. CrossRef
8. For alternative methods towards similar substituted chromenes, see: K. A. Korthals and W. D. Wulff, J. Am. Chem. Soc., 2008, 130, 2898. CrossRef
9. M. Curini, G. Cravotto, F. Epifano, and G. Giannone, Curr. Med. Chem., 2006, 13, 199. CrossRef
10. For recent examples of chromene containing biologically interesting structures, see: F. Borges, F. Roleira, N. Milhazes, L. Santana, and E. Uriarte, Curr. Med. Chem., 2005, 12, 887; CrossRef G. A. Kraus and I. Kim, J. Org. Chem., 2003, 68, 4517; CrossRef J. G. Tangmouo, A. L. Meli, J. Komguem, V. Kuete, F. N. Ngounou, D. Lontsi, V. P. Beng, M. I. Choudhary, and B. L. Sondengam, Tetrahedron Lett., 2006, 47, 3067; CrossRef F. Chimenti, B. Bizzarri, A. Bolasco, D. Secci, P. Chimenti, S. Carradori, A. Granese, D. Rivanera, D. Lilli, M. M. Scaltrito, and M. I. Brenciaglia, Eur. J. Med. Chem., 2006, 41, 208. CrossRef
11. N. Crivillers, N. S. Oxtoby, M. Mas-Torrent, J. Veciana, and C. Rovira, Synthesis, 2007, 1621. CrossRef
12. J.-Y. Cho, S. Barlow, S. R. Marder, J. Fu, L. A. Padilha, E. W. van Stryland, D. J. Hagan, and M. Bishop, Optics Lett., 2007, 32, 671; CrossRef M. R. Bryce, J. Mater. Chem., 1995, 5, 1481. CrossRef
13. K. L. Marshall and S. D. Jacobs, Mol. Cryst. Liq. Cryst., 1988, 159, 181; CrossRef K. L. Marshall, G. Painter, K. Lotito, A. G. Noto, and P. Chang, Lab. Laser Energ. Rev., 2006, 106, 112; K. L. Marshall, B. Schudel, and I. A. Lippa, Proc. Soc. Photo. Inst. Eng., 2003, 5213, 201; R. N. Lyubovskaya, Russ. Chem. Rev., 1983, 52, 736. CrossRef
14. C. Kisker, H. Schindelin, D. Baas, J. Retey, R. U. Meckenstock, and P. M. H. Kroneck, FEMS Microbio. Rev., 1999, 22, 503. CrossRef
15. U. T. Mueller-Westhoff, A. Nazzal, R. J. Cox, and A. M. Giroud, Mol. Cryst. Liq. Cryst. Lett., 1980, 56, 249; CrossRef K. Ohta, H. Takagi, I. Muroki, K. Yamamoto, T. Matsuzaki, T. Inabe, and Y. Maruyama, Mol. Cryst. Liq. Cryst., 1987, 147, 15. CrossRef