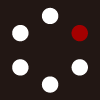
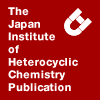
HETEROCYCLES
An International Journal for Reviews and Communications in Heterocyclic ChemistryWeb Edition ISSN: 1881-0942
Published online by The Japan Institute of Heterocyclic Chemistry
e-Journal
Full Text HTML
Received, 5th June, 2012, Accepted, 26th June, 2012, Published online, 28th June, 2012.
DOI: 10.3987/COM-12-12522
■ Microwave-Assisted Synthesis of 2-Aminopyrimidines from Silica Gel-Adsorbed Propargyl Alcohols and Guanidine
Qing-Zhen Chen, Zong-Cang Ding, Yan-Li Ma, Zhen-Dong Wang, and Zhuang-ping Zhan*
College of Chemistry and Chemical Engineering, Department of Chemistry, Xiamen University, 422 Siming Nanlu, Xiamen, Fujian 361005, China
Abstract
A novel methodology toward the synthesis of 2-aminopyrimidines has been developed. The silica gel adsorbed propargyl alcohols and guanidine easily give the corresponding 2-aminopyrimidines under microwave irradiation.2-Aminopyrimidines and their derivatives have been given particular interest due to their diverse biological properties (Figure 1).1 For instance, naturally occurring Meridianin A-E show cytotoxicity towards murine tumor cell lines,1a and Variolin B isolated from the Antarctic sponge Kirkpratickia varialosa display excellent antitumor and antiviral activity.1b In pharmaceutical market, Pyrimethamine,1c Rosuvastatin1d and Imatinib1e have got huge success in virtue of their distinguished activity against malaria, dyslipidemia and chronic myeloid leukemia (CML), respectively.
In the past few decades, the construction of 2-aminopyrimidine moiety has been generally relying on: 1) Amination of 2-Cl or 2-Br-pyrimidines;2 or 2) Condensation of guanidines with 1,3-dicarbonyl compounds or their synthetic equivalents, such as α,β-unsaturated ketones.3 Thus, the development of straightforward and efficient methodologies for the synthesis of 2-aminopyrimidines is still highly desirable.
Lately, our research interest has been focusing on the heterocycle synthesis based on propargyl alcohols. We have demonstrated that various heterocycles can be easily accessed by the propargylation-cycloisomerization tandem reaction.4 Herein, as part of ongoing efforts in our laboratory, we report our recent progress on the synthesis of 2-aminopyrimidines from propargyl alcohols and guanidine for the first time.
We initially investigated the reaction of propargyl alcohol 1a and guanidine 2. The reaction was run in the absence of any catalyst, using chlorobenzene as the solvent. Although all the starting material was consumed in the reaction, we were only able to obtain the desired product in 15% yield. The trimethylsilyl group had fallen off during work-up (Table 1, entry 1). The rest of products were obtained as complex mixtures, and their structures were not determined. We suspect them some decomposed products.
On the basis of our recent work concerning the Cu(OTf)2-catalyzed synthesis of pyrimidines from propargyl alcohols and amidine,4e substrates 1a and 2 were treated with 20 mol% of Cu(OTf)2 in refluxing chlorobenzene. Unfortunately, the yield was still rather low (Table 1, entry 2). In recent years, organic synthesis has greatly benefited from the development of microwave technology.5 We found that the reaction time was sharply shortened under microwave irradiation, but the yield was not increased (Table 1, entry 3).
We next screened various other Lewis acid catalysts to optimize the formation of 3a. However, none of them were able to remarkably improve the reaction efficiency (Table 1, entries 4-9). Other non-metal catalysts, such as 4-methylbenzenesulfonic acid, trifluoromethanesulfonic acid and silica gel, also failed to facilitate this transformation (Table 1, entries 10-12).
Combinations of the adsorbed reagents and microwave irradiation were used to carry out a wide range of reactions.6 Thus, we attempted the MW-assisted synthesis of 2-aminopyrimidine 3a under solvent-free condition. Silica gel was first chosen as the adsorbed reagents, due to its characteristic properties, such as thermal stability and acidity character. We were delighted to find that the reaction accomplished in a shortened time with an increased yield (Table 1, entry 13).7 K10 montmorillonite showed lower activity as the adsorbed reagent (Table 1, entry 14). Our further study revealed that the employment of silica-adsorbed metal catalysts failed to improve the yield of 3a (Table 1, entries 15-16).
With optimized conditions in hand (Table 1, entry 13), the substrate scope of the reaction was examined. A diverse collection of propargyl alcohols underwent this reaction, offering a facile access to 2-aminopyrimidines (Table 2). The alkyne terminus of 1 could accommodate different substituted group (R2 = n-butyl, cyclopropyl, TMS and phenyl). Substrate 1d’ containing a terminal alkyne moiety also took part in the reaction (R2 = H; Table 2, entry 4). Although the same product was obtained in the examples involving 1d and 1d’, the employment of a TMS group led to a considerably improved yield (Table 2, entry 3 vs 4). Various aromatic and heteroaromatic substrates were successfully employed in the reaction to give the corresponding 2-aminopyrimidines. A number of functional groups, such as bromo, methoxy, and methoxycarbonyl, were tolerated under the reaction conditions, allowing the further elaboration of the products (Table 2, entries 6-9). However, the methoxycarbonyl group had an obvious adverse impact on this reaction. In addition, aliphatic propargyl alcohols (R1 = aliphatic) failed to afford the desired 2-aminopyrimidines under this condition.
In conclusion, we have developed a facile method for the synthesis of 2-aminopyrimidines. The silica gel adsorbed propargyl alcohols and guanidine give the corresponding 2-aminopyrimidines under microwave irradiation. The amino group allows further elaboration of the products. Further studies of the application of this methodology to the total synthesis of bioactive natural products are ongoing in our laboratories.
ACKNOWLEDGEMENTS
We thank the NSFC (No. 21072159), the 973 Projects (No. 2011CB935901), the NFFTBS (No. J1030415) and the Science & Technology Bureau of Xiamen (No. 3502Z20103006) for financial support of this project.
References
1. (a) L. H. Franco, E. B. K. Joffé, L. Puricelli, M. Tatian, A. M. Seldes, and J. A. Palermo, J. Nat. Prod., 1998, 61, 1130; CrossRef (b) N. B. Perry, L. Ettonati, M. Litandon, J. W. Blunt, M. H. G. Munro, S. Parkin, and H. Hope, Tetrahedron, 1994, 50, 3987; CrossRef (c) C. O. Obonyo and E. A. Juma, Malar. J., 2012, 11, 2; CrossRef (d) M. Hanefeld, Int. J. Clin. Pract., 2001, 55, 399; (e) H. Joensuu and S. Dimitrijevic, Ann. Med., 2001, 33, 451; CrossRef (f) M. Ban, H. Taguchi, T. Katsushima, S. Aoki, and A. Watanabe, Bioorg. Med. Chem., 1998, 6, 1057; CrossRef (g) C. D. Kwong, J. L. Clark, A. T. Fowler, F. Geng, H. S. III Kezar, A. Roychowdhury, R. C. Reynolds, J. A. Maddry, S. Ananthan, J. A. III Secrist, N.-Y. Shih, J. J. Piwinski, C. Li, B. Feld, H.-C. Huang, X. Tong, N. F. George, and A. Arasappan, Bioorg. Med. Chem. Lett., 2012, 22, 1160; CrossRef (h) E. A. Lindsey, R. J. Worthington, C. Alcaraz, and C. Melander, Org. Biomol. Chem., 2012, 10, 2552. CrossRef
2. (a) D. R. Carver, A. P. Komin, J. S. Hubbard, and J. F. Wolfe, J. Org. Chem., 1981, 46, 294; CrossRef (b) S. Jaime-Figueroa, Y. Liu, J. M. Muchowski, and D. G. Putman, Tetrahedron Lett., 1998, 39, 1313; CrossRef (c) M. K. Elmkaddem, C. Fischmeister, C. M. Thomas, and J.-L. Renaud, Chem. Commun., 2010, 46, 925. CrossRef
3. (a) P. M. Fresneda, P. Molina, S. Delgado, and J. A. Bleda, Tetrahedron Lett., 2000, 41, 4777; CrossRef (b) A. S. Karpov and T. J. J. Mueller, Synthesis, 2003, 2815; CrossRef (c) A. S. Karpov and T. J. J. Mueller, Org. Lett., 2003, 5, 3451; CrossRef (d) S. Goswami, S. Jana, S. Dey, and A. K. Adak, Aust. J. Chem., 2007, 60, 120; CrossRef (e) I. R. Baxendale, S. C. Schou, J. Sedelmeier, and S. V. Ley, Chem. Eur. J., 2010, 16, 89; CrossRef (f) R. S. Keri, K. M. Hosamani, R. V. Shingalapur, and M. H. Hugar, Eur. J. Med. Chem., 2010, 45, 2597; CrossRef (g) P. M. Fresneda, P. Molina, and J. A. Bleda, Tetrahedron, 2001, 57, 2355; CrossRef (h) M. C. Bagley, D. D. Hughes, M. C. Lubinu, E. A. Merritt, P. H. Taylor, and N. C. O. Tomkinson, QSAR Comb. Sci., 2004, 23, 859. CrossRef
4. (a) Z. Zhan, X. Cai, S. Wang, J. Yu, H. Liu, and Y. Cui, J. Org. Chem., 2007, 72, 9838; CrossRef (b) X. Liu, L. Huang, F. Zheng, and Z. Zhan, Adv. Synth. Catal., 2008, 350, 2778; CrossRef (c) Y. Pan, F. Zheng, H. Lin, and Z. Zhan, J. Org. Chem., 2009, 74, 3148; CrossRef (d) L. Hao, Y. Pan, T. Wang, M. Lin, L. Chen, and Z. Zhan, Adv. Synth. Catal., 2010, 352, 3215; CrossRef (e) M. Lin, Q. Chen, Y. Zhu, X. Chen, J. Cai, Y. Pan, and Z. Zhan, Synlett, 2011, 1179; CrossRef (f) T. Wang, X. Chen, L. Chen, and Z. Zhan, Org. Lett., 2011, 13, 3324. CrossRef
5. For recent reviews, see: (a) D. Dallinger and C. O. Kappe, Chem. Rev., 2007, 107, 2563; CrossRef (b) C. O. Kappe, Chem. Soc. Rev., 2008, 37, 1127; CrossRef (c) V. Polshettiwar and R. S. Varma, Acc. Chem. Res., 2008, 41, 629; CrossRef (d) S. Caddick and R. Fitzmaurice, Tetrahedron, 2009, 65, 3325; CrossRef (e) M. A. P. Martins, C. P. Frizzo, D. N. Moreira, L. Buriol, and P. Machado, Chem. Rev., 2009, 109, 4140; CrossRef (f) A. Dastan, A. Kulkarni, and B. Török, Green Chem., 2012, 14, 17; CrossRef (g) J. Gising, L. R. Odell, and M. Larhed, Org. Biomol. Chem., 2012, 10, 2713; CrossRef (h) A. Sharma, P. Appukkuttan, and d. E. E. Van, Chem. Commun., 2012, 48, 1623. CrossRef
6. (a) W. Su, S. Guo, Z. Hong, and R. Chen, Tetrahedron Lett., 2010, 51, 5718; CrossRef (b) A. Serra-Muns, A. Guérinot, S. Reymond, and J. Cossy, Chem. Commun., 2010, 46, 4178; CrossRef (c) D. Tejedor, D. González-Cruz, F. García-Tellado, J. J. Marrero-Tellado, and M. L. Rodríguez, J. Am. Chem. Soc., 2004, 126, 8390; CrossRef (d) V. B. Kurteva and C. A. M. Afonso, Green Chem., 2004, 6, 183; CrossRef (e) C. Ramesh, G. Mahender, N. Ravindranath, and B. Das, Green Chem., 2003, 5, 68; CrossRef (f) S. Balalaie and A. Arabanian, Green Chem., 2000, 2, 274. CrossRef
7. Experimental procedure: To a dry 10-mL flask, propargyl alcohol 1a (0.5 mmol), guanidine (2, 1mmol), CH2Cl2 (2 mL) and silica gel (1 g) were successively added, and were mixed uniformly. Then the solvent was removed under reduced pressure, the residue was then transferred into the domestic microwave oven and irradiated at 700 W. The progress of the reactions was monitored by TLC using CH2Cl2 as eluent. Upon completion, the mixture was extracted into CH2Cl2 (5 mL), then the solvent was removed under reduced pressure. Further purification by column chromatography (petroleum ether and EtOAc as eluent) gave the desired product 3a.