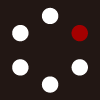
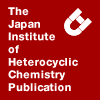
HETEROCYCLES
An International Journal for Reviews and Communications in Heterocyclic ChemistryWeb Edition ISSN: 1881-0942
Published online by The Japan Institute of Heterocyclic Chemistry
e-Journal
Full Text HTML
Received, 27th September, 2012, Accepted, 21st November, 2012, Published online, 27th November, 2012.
DOI: 10.3987/REV-12-753
■ Phthalocyanines and Related Compounds with Chalcogen Functional Groups
Takeshi Kimura*
Center for Instrumental Analysis, Iwate University, 4-3-5 Ueda, Morioka, Iwate 020-8551, Japan
Abstract
5,6-Dibromo-4,7-diethylbenzo[1,2,3]trichalcogenoles could be used as starting compounds for phthalocyanines with sulfur or selenium functional groups at β-positions and alkyl groups at α-positions. Phthalocyanines with dithiastannole rings, trithiole rings, and TTF units, phthalocyanines axially linked with 1,2-benzenedithiols, tetraazaporphyrin fused with four thiophene rings, and related derivatives are described in this review.Phthalocyanines (Pcs) and related compounds have attracted considerable attention for their actual and potential applications in many fields, such as catalysts, optical discs, sensors, semiconductors, sensitizers for photodynamic therapy for cancer, and so forth.1 In these molecules, most of the functionalities depend on their large and flat π-conjugated ring systems and central metal atoms. Pcs are generally insoluble in organic solvents and hence improving their solubility is one of the most important subjects for their design and utilization. In addition, a compact and simple molecular structure would be required to deposit Pcs on the surface of metals and electrodes under reduced pressure. It is also significant that Q band absorption lies in the near infrared region to apply Pcs to new functional materials. For instance, if the Q band absorption can correspond to the solar spectrum by its broadening and redshifting, we would be able to spread the possibility of Pcs as excellent dyes for solar cells. Expanding a π-conjugated ring system and introducing heteroatoms to the periphery of Pcs can be methods for shifting the Q band absorption to the lower energy field.2 An efficient redshift of the Q band absorption can be accomplished by attaching electron-donating groups at the α-positions of Pcs.3,4
On the other hand, in the course of our study regarding cyclic oligosulfides,5 a reaction of 2,3,5,6-tetrabromo-1,4-diethylbenzene with elemental sulfur in DBU at 130 °C unexpectedly produced 5,6-dibromo-4,7-diethylbenzo[1,2,3]trithiole (1a) (Scheme 1).6 Similarly, 5,6-dibromo-4,7-diethylbenzo-[1,2,3]triselenole (1a’) was obtained by the reaction of tetrabromodiethylbenzene with elemental selenium in DBU. In these compounds, if two bromine atoms can be substituted with two nitrile groups, it is possible to apply 1a and 1a’ to the synthesis of Pcs with sulfur or selenium functional groups. Although the direct substitution of the bromine atoms of 1a with CuCN did not give a good result, we could prepare phthalonitrile derivatives by a method using protecting groups. In this review, I describe Pcs with sulfur or selenium functional groups at the β-positions together with other related studies. In the first part, synthetic procedures for phthalonitriles with one or two sulfur or selenium functional groups at the 3, 4, 5, and 6 positions are briefly reviewed. After this part, studies for 1) Pcs with dithiastannole rings, trithiole rings, and TTF units, 2) tetraazaporphyrin fused with thiophene rings, and 3) Pcs and porphyrins, axially linked with 1,2-benzenedithiols, are shown and their related studies are described.
Preparation of phthalonitriles with sulfur or selenium functional groups
In general, phthalonitriles with one or two sulfur substituents have been utilized to synthesize Pcs with four or eight sulfur functional groups at the α- or β-positions. To prepare target phthalonitriles, 3-nitrophthalonitrile (2),7 4-nitrophthalonitrile (3),7 4,5-dichlorophthalonitrile (4),8 and 3,6-bis(trifluoro-methylsulfonyloxy)phthalonitrile (5)3 are the most important starting materials. While the former three compounds can now be purchased, the last one was reported by Cook et al. in 2003. In the same year, Kobayashi et al. also described a reaction of 5 with some thiols.4
In 1980, Derkacheva et al. reported that an aromatic nucleophilic substitution reaction of 2 and 3 with aromatic thiols produced phthalonitriles with one arylthio group at the 3- or 4-position.4 Many Pcs with four sulfur functional groups at the α- or β-positions have been prepared using these phthalonitriles.9
4,5-Dichlorophthalonitrile (4) was prepared from 4,5-dichlorophthalic acid by Wöhrle et al. in 1993.8 Reactions of 4 with many thiols produced 4,5-bis(alkylthio)- and 4,5-bis(arylthio)phthalonitriles, which could have been applied to the synthesis of β-octakis(alkylthio)- or β-octakis(arylthio)phthalocyanines.10
In related studies, several important compounds were prepared using 4 and its derivative. For example, 4,5-bis(propylsulfonyl)phthalonitrile (6) was prepared from 4 by a substitution reaction with 1-propanethiol and subsequent oxidation of the product.11,12 Compound 6 could be applied to the preparation of Pcs with an electrical push-pull system2gh,11 and with an effect of electric field-induced changes in absorption intensity.13 In addition to simple thiols, aromatic dithiol could react with 4 to produce cyclic derivative 7,14 and the product was utilized to prepare optically active Pcs. 2,3-Dibromo-6,7-dicyanonaphthalene could be converted to 2,3-bis(alkylthio)-6,7-dicyanonaphthalene (8) by a reaction with alkylthiols.15,16 Product 8 was tetramerized on treatment with CuCl/DBU to produce Cu (II) naphthalocyaninato with eight alkylthio groups at the peripheral positions.
In 2005, Decurtins et al. used phthalonitrile 4 to construct a tetrathiafulvalene (TTF) unit with two nitrile and two propylthio groups via 4,5-bis(benzylthio)phthalonitrile (9) (Scheme 2).17 The benzyl groups of 9 could be removed by a reaction with toluene in the presence of aluminum chloride, which is a simple and significant method for the debenzylation and may proceed via benzodithiate as an intermediate.18 Finally, a condensation reaction of 10 with 4,5-bis(propylthio)-1,3-dithiole-2-thione in triethylphosphite gave the corresponding TTF derivative 11.
Instead of 4,5-bis(propylthio)-1,3-dithiole-2-thione, Wang et al. used 1,3-dithiole-2-thione (12) fused with 18-membered dithiacrown ether (Scheme 3).17,18 A condensation reaction of 12 with 10 in triethylphosphite produced TTF derivative 13.
To prepare phthalonitrile with two sulfur functional groups at the 3,6-positions, Cook et al. and Kobayashi et al. used an aromatic nucleophilic substitution reaction of 3,6-bis(trifluoromethane-sulfonyloxy)phthalonitrile (5) with alkylthiols, giving phthalonitrile (14) (Scheme 4).3,4,21 A modified reaction using a p-toluenesulfonyloxy group was performed to produce 3,6-bis(alkylthio)- and 3,6-bis(arylthio)phthalonitriles and related compounds,22 and Kobayashi et al. were able to utilize the procedure for the preparation of selenium derivative 15b.23 The reaction of 5 could be applied to the C-C bond formation using alkyl zinc bromide and a nickel catalyst.24
3,4,5,6-Tetrafluorophthalonitrile (17) can be used to prepare phthalonitriles (18) with two or four sulfur functional groups (Scheme 5).25 The number of phenylthio groups, introduced on the benzene ring, could be controlled by the reaction conditions. 3,4,5,6-Tetrachlorophthalonitrile was also converted to tetrakis(phenylthio)phthalonitrile.26
As a substrate for octakis(alkylthio)tetraazaporphyrin derivatives, cis-bis(benzylthio)fumaronitrile (19) was prepared from a reaction of cis-1,2-dicyano-1,2-ethylenedithiolate salt with benzyl chloride (Scheme 6).27,28 The benzylthio group is important for the functional exchange of tetraazaporphyrins via Birch reduction of C-S bonds. Instead of benzyl chloride, compounds 20a and 20b were reacted with the dithiolate salt to give TTF derivatives 21a and 21b with the 12-membered and 18-membered tetrathiacrown ethers, respectively.29,30
As a related compound, TTF derivative 22 with two methylthio and two nitrile groups was prepared by a condensation reaction of 4,5-bis(methylthio)-1,3-dithiole-2-thione with 4,5-dicyano-1,3-dithiole-2-one (Scheme 7).31 Tetraazaporphyrin metal complexes with four TTF units were prepared from a related compound of TTF derivative 22.32
In 2004, we reported that 5,6-dibromo-4,7-diethylbenzo[1,2,3]trichalcogenoles (1a) and (1a’) were prepared from 2,3,5,6-tetrabromo-1,4-diethylbenzene (Scheme 1).6 Protection of the sulfur functional group was undertaken to give 23a (Scheme 8).
After a cyanation reaction of 23a with CuCN in DMF at reflux and a exchange reaction of the 2-cyanoethyl group with the benzyl group, we could obtain 3,6-diethyl-4,5-bis(benzylthio)phthalonitrile (24a). A reaction of 3,6-diethyl-1,2-bis(benzylthio)-4,5-dibromobenzene with CuCN under a similar reaction condition gave 24a in low yield. In the case of 1a’, we used a p-nitrophenethyl group instead of the propionitrile group and could prepare 3,6-diethyl-4,5-bis(benzylseleno)phthalonitrile (24a’) by an alkyl exchange reaction with the benzyl group.
Next, we could use an o-xylylene group as a protecting group for o-benzenedithiol instead of two benzyl groups (Scheme 9).33,34 Benzotrithiole derivatives (1a-d) were prepared by the procedure as described above (Scheme 1) and we converted them to o-xylylenedithio derivatives (25a-d). Then, phthalonitriles (26a-d) with two alkyl groups next to the nitrile groups were prepared. In this case, we did not need the temporary propionitrile protection.
By Decurtins’ procedure as shown in Scheme 2, however, we cannot introduce any substituents on the 3,6-positions of phthalonitrile. Therefore, we prepared phthalonitriles with the TTF unit from 3,6-dialkyl(o-xylelenedithio)phthalonitrile (26) (Scheme 10).35
The o-xylylene group of 26 was removed by a reaction with toluene in the presence of aluminum chloride. After the deprotection reaction, the intermediate was treated with carbonyl diimidazole at reflux to give carbonyl derivatives (27a-c). Phthalonitriles (27a-c) were condensed with 4,5-bis(methylthio)-1,3-dithiole-2-thione to produce the corresponding TTF derivatives (28a-c), respectively.
Photolysis of dibromobenzotrithiole (1a) in dichloromethane under argon produced 2,3,7,8-tetrabromo-1,4,6,9-tetraethylthianthrene (30) via desulfurization, dimerization, and ring contraction reactions (Scheme 11).36-38 2,3,8,9-Tetrabromodibenzotetrathiocin (29) was prepared on treatment of 1a with sodium borohydride and hydrogen peroxide, and photolyzed to produce 30. Compound 30 was further treated with CuCN to give 2,3,7,8-tetracyano-1,4,6,9-tetraethylthianthrene (31).
To prepare 2,3,7,8-tetracyanothianthrene (34), Abramov et al. used a reaction of 4-bromo-5-nitro-phthalonitrile (32) with thioacetamide (Scheme 12).39 The reaction produced bis(2-nitro-4,5-dicyano-phenyl) sulfide (33) and the product 33 was heated with thioacetamide and triethylamine to give 34.
It is well known that a reaction of arylhalogenides with sodium sulfite in a water-dioxane mixture in the presence of cupric salts produces aromatic sulfonic acids. Although the yields are low, the treatment of phthalonitrile 35 with sodium sulfite and cupric salts produced 2,3 or 3,4-dicyanobenzenesulfonic acid (36) (Scheme 13).40 Pcs with the sulfonic acid groups could be prepared from 36.
Preparation and functional exchange of phthalocyanine derivatives
There have been many reports of symmetrically and unsymmetrically substituted Pcs 37, 38, 39, and 40 with four or eight simple alkylthio or arylthio groups at the α or β-positions and related compounds; 37 and 38 consist of several positional isomers (Scheme 14).3,4,9,10,21-24 These compounds could be prepared from the corresponding mono- or di-substituted phthalonitriles. In addition, Pcs with cage-like dithia- or tetrathiacrown ethers at the β-positions were reported as related compounds.41 Pcs with alkylseleno and arylseleno groups are rare,6,24 and it seems that there is no report of Pcs with tellurium functional groups. In these compounds, the Q band absorptions of the α-substituted derivatives were observed in a lower energy region than were those of the β-substituted derivatives.4
There are a few reports about the exchange of sulfur functional groups on tetraazaporphyrin derivatives. The initial work was reported by Hoffman et al. in 1990.27 The debenzylation from compound 42 was performed with Birch reduction using sodium and ammonia at low temperature. The resultant octathiolate anions produced μ-[(tetraazaporphyrinoctathiolato) Ni (II)]tetrakis[di-t-butyltin (IV)] (43) by a reaction with four molecules of di-t-butyl tin dinitrate under nitrogen (Scheme 15).27
At first, they expected that the product was tetraazaporphyrin with four dithiastannole rings. However, X-ray crystallography showed that the structure of the tetraazaporphyrin 43 had four N-Sn bonds and the Sn atoms had a pentacoordinated structure. Hoffman et al. explained that compound 43 was more stable than was tetraazaporphyrin with the dithiastannole rings because of the bond formation between the nitrogen and stannum atoms. In addition to compound 43, several unsymmetrical tetraazaporphyrins with metal-sulfur bonds were prepared via the debenzylation and metalation.42
The S-C bond cleavage could be performed by the mercury (II) cation as shown in Scheme 16.43 The cleavage of tetraazaporphyrin (44) from a polymer backbone using mercury (II) acetate followed by a reaction with [1,2-bis(diphenylphosphynoethane)]dichloronickel (II) produced nickel (II) complex (45).
Hoffman et al. reported the exchange of sulfur functional groups on tetraazaporphyrin. However, there was no report about the sulfur-functional exchange on phthalocyanines using Birch reduction. Therefore, we prepared octakis(benzylchalcogeno)octaethylphthalocyanines (46a,b) from phthalonitriles (24a,b) by treatment with lithium alkoxide (Scheme 17).6
In the UV-vis spectrum, the Q band absorption of 46b was observed at 757 nm, which is similar to that of 46a (755 nm). To prepare peripherally modified Pcs, 46a,b were treated with lithium/THF/ammonia at –78 °C and then dibutyl tin dichloride to produce Pcs 47a,b with four dichalcogenastannole rings, respectively. The selenium-functional exchange on Pcs was first performed by this reaction.
As shown in Scheme 9, we can prepare phthalonitriles (26a-d) with two alkyl groups such as ethyl (Et), butyl (Bu), octyl (Oc), and dodecyl (Dd) groups. The treatment of 26a-d with lithium in 1-pentanol gave tetrakis(o-xylylenedithio)phthalocyanines (48a-d) in moderate yields (Scheme 18).33 Compound 48b was further treated with nickel (II) acetate to produce phthalocyaninato nickel (II) complex (48b-Ni).
The reductive removal of four o-xylylene groups from Pcs 48b,c was performed by the similar procedure as described above. Octathiolate anions generated were then reacted with elemental sulfur to give Pcs 49b,c with four trithiole rings, respectively.33,44,45 The NMR spectra of 49c were measured using CDCl3 (Figure 1a) and CDCl3 containing 5vol% of TFA-d (CDCl3/TFA-d) (Figure 1b). In the spectrum, the signals of the methylene groups connected directly to the benzene ring and of the next methylene groups were observed as two split signals, respectively (Figure 1b). The UV-vis spectrum of 49c, measured in the presence of 3vol% of TFA, is shown in Figure 2.44
There are three ways of preparing unsymmetrically substituted Pcs.1e,46-48 Thus, 24a was mixed with 4-t-butylphthalonitrile and then treated with lithium alkoxide to produce unsymmetrically substituted Pcs 50 and 51 with two or four benzylthio groups (Scheme 19).44,49 Compound 51 consisted of opposite and adjacent isomers 51a and 51b but we could not separate these isomers. Treatment of phthalocyanine 50 with nickel (II) acetate yielded the corresponding metal complex 50-Ni.
Two benzyl groups of 50 and 50-Ni were removed with lithium/THF/ammonia at –78 °C under argon, and the dithiolate anions generated were then reacted with elemental sulfur to give Pcs 52 and 52-Ni, respectively (Scheme 20).44 Similar treatment of the mixtures of 51a and 51b produced the corresponding Pcs with two trithiole rings.
Phthalocyanines and related derivatives with the TTF unit
Tetrathiafulvalene (TTF) is a strong electron donor and can be useful for developing new functional materials.50 If we can combine the TTF unit with Pcs, the products would show unique electro-chemical and optoelectronic properties. There are two ways to connect TTF with Pcs. The first is a connection of Pc with TTF using some linkers while the second is a direct fusion with no spacers.
The former method is described first. In 1996, Bryce et al. first reported Pc 54 with eight TTF units linked by ether bonds at the β-positions (Scheme 21).51 The obtained product was virtually insoluble in most organic solvents and showed the Q band absorption as split peaks (647 and 677 nm, DMF). When the UV-vis spectrum of compound 54 was measured in the presence of iodine, new broadened absorption appeared at around 880 nm. They could demonstrate that the fluorescence from related compounds of 54 is efficiently quenched with the intramolecular electron transfer.51b
In the same year, Cook et al. also reported Pcs with one or two TTF units at the α-positions (Scheme 22).52 Compound 57 and its mono-TTF derivative were deposited on quartz as spin-coated films. The UV-vis spectra of the films on the quartz were measured in the presence and absence of iodine. In the presence of iodine, broadened absorption appeared at around 900 nm in the spectra. It was reported that the mono-TTF derivative is the first discotic liquid crystalline of phthalocyanine linked with the TTF unit.
In addition to Pcs, Bryce et al. prepared octaazaphthalocyanine (59) with eight TTF units from 58 (Scheme 23).53 They measured the UV-vis and emission spectra of 59 but they could not observe any fluorescence of this compound. In the presence of iodine, the UV-vis spectrum of 59 showed new absorption at around 880 nm.
In 2005, Nolte et al. prepared tetrakis(tetrathiafulvalene-crown ether) substituted phthalocyanines 61 from dithiacrown ether 60 (Scheme 24).54 It was reported that the helical tape of 61 was formed by slow addition of dioxane to its chloroform solution at 5 °C. In addition, compound 61 was mixed with tetracyanoquinodimethane [TCNQ] to produce a charge-transfer complex, which showed new absorption in the UV-vis spectrum at 750 nm. Formation of the TCNQ–/TTF+ charge-transfer complex was confirmed by FT-IR which showed the nitrile stretch of the TCNQ radical anion at 2179 cm-1.
Tetraazaporphyrins 62a, 62a’, 62b, and 62c were prepared by a tetramerization reaction of phthalonitrile 21 with butoxy magnesium (Scheme 25).29,30 The treatment of compound 62 with tetrafluoro-tetracyanoquinodimethane [F4TCNQ] produced an electron transfer complex, which was determined by the UV-vis and ESR spectra.
The electron transfer complex derived from 62a showed new absorption at 760 and 863 nm in the UV-vis spectrum and the nitrile stretch of the TCNQ radical anion at 2191 cm-1 in the IR spectrum. The ESR spectrum of this complex showed a broadened signal at g = 2.008. Compound 62 was reacted with Ag+ and Hg2+ at the thiacrown moiety to produce the corresponding metal complexes. The reaction was monitored by the UV-vis spectroscopy.
Recently, Yin et al. prepared unsymmetrical tribenzotetraazaporphyrin (64) with two TTF units by a reaction of dithiomaleonitrile derivative 63 with an excess amount of phthalonitrile in the presence of butoxy magnesium (Scheme 26).55,56 The magnesium complex produced the corresponding metal-free derivative by treatment with trifluoroacetic acid. Compound 64 and its metal-free derivative were treated with F4TCNQ to produce the electron transfer complex, respectively, which showed absorption at around 866 nm in the UV-vis spectra. In addition, complex 64/F4TCNQ showed absorption of the nitrile group at 2196 cm-1 in the FT-IR spectrum.
In 2002, silicon Pc 67 with two axial ligands containing two TTF units and its related compounds were synthesized by a reaction of silicon phthalocyaninato dichloride (65) and benzoic acid derivative 66 connected with the TTF unit (Scheme 27).57 The intensity of the emissions of 67 and related compounds depended on the length of the linker and the number of TTF units.
The second method for preparing Pcs with the TTF units is their direct connection without any spacers. In 2005, Decurtins et al. prepared phthalocyanine (68) with four TTF units from TTF derivative 11 (Scheme 28).17 Although Pc 68 has alkyl groups on the TTF units, the molecule seemed to have a highly aggregative property in solution even at low concentration.
It was reported that the Zn derivative of 68 (68-Zn) dissolved in THF did not show any luminescence in a neutral form. Compound 68-Zn could be oxidized by a five-fold excess of FeCl3, which produced the corresponding electron transfer complex 68-Zn/FeCl3. In the UV-vis spectrum, the Q band absorption of 68-Zn/FeCl3 was observed at 700 nm. Interestingly, when the solution of this species was irradiated by the light (380 nm), 68-Zn/FeCl3 exhibited very strong luminescence at 710 and 786 nm. It is expected that an electron transfer from the TTF unit to the central Pc did not occur because the TTF units of 64-Zn were oxidized by FeCl3.
On the other hand, tetra-TTF annulated Pcs 69b and 69c were obtained from phthalonitriles 28b and 28c as a dark-green powder, respectively (Scheme 29). On measurement of the UV-vis spectrum, the Q band absorption of 69c was observed at 770 nm. In the presence of iodine, while the spectrum showed the broadened and slightly blue-shifted Q band absorption, the solution of 69c showed no fluorescence in the region between 770 nm and 900 nm.
Wang et al. reported Pc 70 with four TTF units, which were further connected by four dithiacrown ethers (Scheme 30).20 It was reported that Pc 70 produced an electron transfer complex on treatment with TCNQ. In the UV-vis spectrum of the complex measured in dichloromethane, while the absorption at around 400 nm was changed dramatically by addition of TCNQ compared with that of 70 measured without TCNQ, no change of the Q band absorption was reported. In addition, it was shown that compound 70 had good selectivity for the Na+ ion.
Recently, tetraazaporphyrins (72) with four TTF units were reported by Yin et al. (Scheme 31).32 Since products 72 have eight pentyloxycarbonyl groups, these compounds did not show an electron transfer band in the presence of TCNQ in the UV-vis spectrum. When compound 72 was mixed with F4TCNQ in dichloromethane, the solution showed a new CT band at 863 nm in the UV-vis spectrum.
To prepare unsymmetrical Pcs with the TTF unit and the β-octyloxy groups,58,59 phthalonitriles 28c and 73 were mixed and treated with lithium alkoxide to produce Pcs 74 and 75 (Scheme 32). It appeared that compound 75 consisted of opposite and adjacent isomers. Unfortunately, we could not separate these isomers. The reaction of compound 74 with nickel (II) acetate produced the corresponding nickel (II) complex 74-Ni.
Scheme 33 shows the preparation of unsymmetrical Pcs with the TTF units and the a-octyloxy groups.60 In this case, mono-TTF-derivative 77, di-TTF-derivatives 78 and 79 as opposite and adjacent isomers, and tri-TTF-derivative 80 were obtained. Furthermore, we treated compound 77 with nickel (II) acetate and the reaction produced the corresponding nickel (II) complex 77-Ni. In the UV-vis spectrum of 74, the Q band absorption was observed as split peaks at 681 nm and 705 nm (Figure 3). In contrast, 77 showed the absorption at 771 nm. The UV-vis spectrum of 77 in the presence of iodine showed the broadened and red shifted Q band absorptions (824 and 863 nm).
In the 1H NMR spectrum measured in the presence of an excess amount of iodine, compound 77 showed broadened signals for the octyl, octyloxy, and aromatic protons. When the solution was measured by ESR at room temperature, one strong, broad signal was found at g = 2.00763 in the spectrum (Figure 4). The g value of the electron transfer complex 77•+ could be related to the radical cation of TTF.61
Unsymmetrical Pc 82 with one TTF unit was prepared from TTF derivative 81 and 4-t-butyl phthalonitrile (Scheme 34).62 This compound produced LB films by a π-π interaction between aromatic components. The LB films showed low conductivity at room temperature. In contrast, the conductivity increased by exposure of the film to I2 vapor.
As related compounds, Becher et al. reported symmetrical and unsymmetrical porphyrin derivatives bearing the TTF unit.63,64 Jeppesenn et al. reported tetrathiafulvalene-calix[4]pyrrole derivatives whose four pyrrole units did not conjugate each other.65,66 Takimiya et al. reported a porphyrin derivative with TTF connected by a linker.67,68
Tetraazaporphyrins fused with sulfur or selenium heterocycles
It is known that tetraazaporphyrins that are fused with four five-membered heterocycles such as furan, pyrrole, and thiophene at their 3,4-positions are extremely unstable, although these compounds can be considered as isosteric structures of phthalocyanine.69
If the four five-membered heterocycles are linked at their 3,4-positions on the tetraazaporphyrin skeleton, their structures cannot be represented by a usual bonding system. For example, it is probable that tetra(3,4-thieno)tetraazaporphyrin (3,4-TTTAP) contains the unusual tetravalent sulfur atom in one thiophene ring.70 This tetravalent sulfur atom is a bonding system similar to the well-known “nonclassical” thiophene, thieno[3,4-c]thiophene (3,4-TT).71
Despite many early attempts to prepare tetraazaporphyrins with the four five-membered heterocycles, tetra(2,3-thieno)tetraazaporphyrin bearing four thiophene units linked at their 2,3-positions and several related compounds were reported as stable derivatives (Scheme 35).72 Tetra(2,3-thieno)tetraaza-porphyrins (84a,b) were prepared by the tetramerization reaction of 2,5-dialkyl-3,4-dicyanothiophenes (83a,b), respectively. Compounds 84a, 84b, and 85b exhibited stable liquid-crystalline phases and optical microscopy revealed leaf textures with linear birefringent defects, which are characteristic of discotic hexagonal phases.
Thienotribenzoporphyrazines and related compounds were synthesized by Cook et al. and Kobayashi et al. (Scheme 36).73 Compounds 88 bore six or eight alkyl groups and showed the Q band absorption which shifted to the lower energy field relative to Pcs. The spin-coated films of 88 and 89 showed broad absorption extending into the near IR region. The broadband absorbers have potentials as filters and as light-absorbing molecules for devices such as solar cells. Compounds 88 and 89 exhibited discotic mesophase behavior.
Although the synthesis of 3,4-TTTAP 91 by a tetramerization reaction of 90 was described in 1995, characterization of the products was not given (Scheme 37).74 So, preparing the 3,4-TTTAP derivative and examining its structure and optical and electrochemical properties could be of considerable interest.
Therefore, we prepared 2,5-diphenyl-3,4-dicyanothiophene (92) from tetrabromothiophene. By a tetramerization reaction of 92, bis(4-methylpyridine)-1,3,5,7,9,11,13,15-octaphenyltetra(3,4-thieno)tetraazaporphyrinato ruthenium (II) (93) with a nonclassical bonding system was obtained as a dark-green solid in 2% yield (Scheme 38).75
The thiophene rings of 93 are deformed by steric repulsion between the eight phenyl groups. The Q band absorption of 93 was observed at 758 nm in the UV-vis spectrum and the MCD curve corresponding to the Q band absorption consisted of Faraday A-term-like dispersed absorptions at 746 and 776 nm.
Tetraazaporphyrins with thiadiazole and selenadiazole rings were prepared by Ercolani et al. and Hoffman et al. and it was reported that the compounds are stable (Scheme 39).76,77 Since there has been a recent review on these molecules,78 I am not treating these compounds in this review.
There are several types of dinuclear phthalocyanines with a flat structure.79,80 However, gable-type dinuclear phthalocyanine is rare.81 To prepare gable-type dinuclear phthalocyanine linked by the dithiin ring, 31 was prepared from compound 1a as a bent molecule, as described in Scheme 11.38 Pc 94 linked by the dithiin ring was constructed by treatment of a mixture of 31 and 73 with lithium alkoxide (Scheme 40).38 In the UV-vis spectra measured in chloroform, the Q band absorptions of 94 and 95 were observed at 702 nm and 704 nm, respectively.
Phthalocyanines and related derivatives with chalcogen ligands
To construct functionalized Pcs, it is possible to select central metal atoms and axial ligands. Phthalocyaninato titanium (IV) dichloride has two chlorine atoms situated on the same face of the macrocycle, which was determined by X-ray crystallographic analysis.82 Therefore, the titanium complex could connect to the bidentate ligand in the one face of the macrocycle.
In 1984, Deronzier et al. prepared porphyrinato titanium (IV) complexes (97a,b) through treatment of tetraphenylporphyrinato titanium (IV) (96) with catechol or toluenedithiol as bidentate ligands (Scheme 41).84 The electrochemical reduction of these complexes gave the corresponding anion radicals, respectively. The anion radical derived from 97b showed one broad signal in the ESR spectrum.
Hanack et al. reported phthalocyaninato titanium (IV) complexes (99) (Scheme 42).84 The nonlinear optical (NLO) properties of some of the complexes were determined in order to evaluate the potential role of these new compounds in optical limiting devices.
Kobayashi et al. used 2,2’-biphenyldiol, hexahydroxytriphenylene, and octahydroxyphthlocyanine and synthesized monophthalocyanine-coordinated, triphthalocyanine-coordinated, and tetraphthalocyanine-coordinated complexes (100), (101), and (102) (Scheme 43).85 The UV-vis spectra and MCD spectra were measured and molecular orbital calculations were performed to characterize the electronic state of these compounds. In addition, phthalocyaninato titanium (IV) complexes with chiral ligands were prepared by a similar procedure.86
In 2005, Chen et al. reported Pcs 103 axially connected with fullerene (Scheme 44).87 When the fluorescence spectrum was measured, fluorescence peaks of the C60 moiety of 104 appeared in a region similar to that of C60 but the intensity of the complex was low compared with that of C60.
Treatment of 105 with benzenedithiol produced 106a (Scheme 45).88 A similar reaction of 105 with benzenediselenol gave compound 106b. In the 1H NMR spectrum of 106a, signals for the ethyl group of benzenedithiolate were observed at δ = 0.23 (t) and 1.81 (q) ppm. The signals for the ethyl group of 106b were observed at δ = 0.29 (t) and 1.93 (q) ppm. In the UV-vis spectra, the Q band absorptions of 106a and 106b were observed at λmax = 764 and 766 nm, respectively.
A solution of benzenedithiol was then added to 96 and the reaction gave 107a with benzenedithiolate as the axial ligand (Scheme 46). A similar treatment of 96 with benzenediselenol produced 107b.
In the 1H NMR spectrum, while signals for the ethyl group of 107a were observed at δ = 0.20 (t) and 1.64 (q) ppm, those of 107b appeared at δ = 0.27 (t) and 1.80 (q) ppm. Compounds 107a,b exhibited two split Soret band absorptions at λmax = 399 and 432 nm for 107a and λmax = 396 and 427 nm for 107b.
Compound 108 was added to the solution of titanium complex 105 and this reaction gave double-decker Pc 109 in 17% yield.89 The Q band absorption of Pc 109 was observed at λmax = 757 nm in the UV-vis spectrum, which is a broader bandwidth than those of 105.
CONCLUSION
In this review, I described phthalocyanines and related compounds with sulfur or selenium as the functional groups at the α- or β-positions and as the axial ligand. Although there are many phthalonitriles with one or more sulfur functional groups, phthalonitriles with the substituents at the 3,6-positions would be the most important compounds, because the α-substituents on phthalocyanines can more affect their optical and electrochemical properties than can the β-substituents. It appeared that Birch reduction of the benzylthio groups on tetraazaporphyrins or phthalocyanines could be useful for the exchange of the sulfur functional groups. In addition, tetraazaporphyrins or phthalocyanines with the TTF or thiophene unit were prepared by the several procedures and some of the products would be applied to sensors, liquid crystals, nonlinear optical materials, and so forth. Finally, the axial ligand on the titanium atom of phthalocyanine could effectively reduce the aggregative properties and the several complexes showed the nonlinear optical property.
References
1. a) ‘The Porphyrin Handbook’, Vols. 15-20, ed. by K. M. Kadish, K. M. Smith, and R. Guilard, Academic Press, San Diego, 2003; b) ‘Phthalocyanines: Properties and Applications’, Vols. 1-4, ed. by C. C. Leznoff and A. B. P. Lever, VCH, New York, 1989-1996; c) G. de la Torre, C. G. Claessens, and T. Torres, Chem. Commun., 2007, 2000; d) G. Bottari, G. de la Torre, D. M. Guldi, and T. Torres, Chem. Rev., 2010, 110, 6768; CrossRef e) J. Mack and N. Kobayashi, Chem. Rev., 2011, 111, 281. CrossRef
2. a) M. J. Cook, A. J. Dunn, S. D. Howe, and A. J. Thomson, J. Chem., Perkin Trans. 1, 1988, 2453; b) M. Brewis, G. J. Clarkson, P. Humberstone, S. Makhseed, and N. B. McKeown, Chem. Eur. J., 1998, 4, 1633; CrossRef c) N. Kobayashi, H. Miwa, and V. N. Nemykin, J. Am. Chem. Soc., 2002, 124, 8007; CrossRef d) N. Kobayashi and T. Fukuda, J. Am. Chem. Soc., 2002, 124, 8021; CrossRef e) N. Kobayashi, J. Mack, K. Ishii, and M. J. Stillman, Inorg. Chem., 2002, 41, 5350; CrossRef f) F. Mitzel, S. FitzGerald, A. Beeby, and R. Faust, Chem. Eur. J., 2003, 9, 1233; CrossRef g) T. Fukuda, S. Masuda, and N. Kobayashi, J. Am. Chem. Soc., 2007, 129, 5472; CrossRef h) T. Fukuda, S. Masuda, N. Hashimoto, and N. Kobayshi, Inorg. Chem., 2008, 47, 2576. CrossRef
3. P. M. Burnham, M. J. Cook, L. A. Gerrard, M. J. Heeney, and D. L. Hughes, Chem. Commun., 2003, 2064. CrossRef
4. N. Kobayashi, H. Ogita, N. Nonaka, and E. A. Luk’yanets, Chem. Eur. J., 2003, 9, 5123. CrossRef
5. T. Kimura, S. Ogawa, and R. Sato, Mini-Rev. Org. Chem., 2007, 4, 15. CrossRef
6. T. Kimura, A. Yomogita, T. Matsutani, T. Suzuki, I. Tanaka, Y. Kawai, Y. Takaguchi, T. Wakahara, and T. Akasaka, J. Org. Chem., 2004, 69, 4716. CrossRef
7. V. M. Derkacheva and E. A. Luk’yanets, Zhurnal Obshchei Khimii, 1980, 50, 2313.
8. D. Wöhrle, M. Eskes, K. Shigehara, and A. Yamada, Synthesis, 1993, 194. CrossRef
9. Since there have been a great number of reports regarding Pcs with four alkylthio and arylthio groups at the α or β-positions and related compounds, I do not treat these compounds in this review.
10. As a result of a research with SciFinder, there are more than 150 reports about symmetrical and unsymmetrical Pcs prepared from 4,5-dialkylthiophthalonitriles and 4,5-diarylthiophthalonitriles. Recent reports published in 2011 and 2012 are shown below; a) P. Zimcik, V. Novakova, K. Kopecky, M. Miletin, R. Zeynep, U. Kobak, E. Svandrlikova, L. Váchová, and K. Lang, Inorg. Chem., 2012, 51, 4215; CrossRef b) T. Fukuda, Y. Kikukawa, S. Takahashi, and N. Kobayashi, Chem. Asian. J., 2012, 7, 751; CrossRef c) M. M. Ayhan, A. Singh, C. Hirel, A. G. Gürek, V. Ahsen, E. Jeanneau, I. Ledoux-Rak, J. Zyss, C. Andraud, and Y. Bretonnière, J. Am. Chem. Soc., 2012, 134, 3655; CrossRef d) A. Altındal, M. Coşkun, and Ö. Bekaroğlu, Synth. Met., 2012, 162, 477; CrossRef e) Z. Hao, X. Wu, R. Sun, C. Ma, and X. Zhang, Chem. Phys. Chem., 2012, 13, 267; CrossRef f) A. L. Ugar, H. A. Dincer, and A. Erdogmus, Polyhedron, 2012, 31, 431; CrossRef g) D. Pietrangeli, A. Rosa, A. Pepe, and G. Ricciardi, Inorg. Chem., 2011, 50, 4680; CrossRef h) U. Siemeling, C. Schirrmacher, U. Glebo, C. Bruhn, J. E. Baio, L. Arnadottir, D. G. Castner, and T. Weidner, Inorg. Chim. Acta, 2011, 374, 302; CrossRef i) M. Canlica and T. Nyokong, Polyhedron, 2011, 30, 1975; CrossRef j) P. Şen, F. Dumludağ, B. Salih, A. R. Ö zkaya, and Ö. Bekaroğlu, Synth. Met., 2011, 161, 1245; CrossRef k) Y. Zhang, P. Ma, P. Zhn, X. Zhang, Y. Gao, D. Qi, Y. Bian, N. Kobayashi, and J. Jiang, J. Material. Chem., 2011, 21, 6515; CrossRef l) N. Durmus, H. Yaman, C. Gol, V. Ahsen, and T. Nyokong, Dyes Pigments, 2011, 91, 153; CrossRef m) T. Booysen, F. Matemadombo, M. Durmus, and T. Nyokong, Dyes Pigments, 2011, 89, 111; CrossRef n) D. Atilla, A. G. Gürek, T. V. Basova, V. G. Kiselev, A. Hassan, L. A. Sheludyakova, and V. Ahsen, Dyes Pigments, 2011, 88, 280; CrossRef o) N. W. Polaske, H.-C. Lin, A. Tang, M. Mayukh, L. E. Oquendo, J. T. Green, E. L. Ratcliff, N. R. Armstrong, S. S. Saavedra, and D. V. McGrath, Langmuir, 2011, 27, 14900; CrossRef p) A. Nas, E. C. Kaya, H. Kantekin, A. Soekmen, and V. Cakir, J. Organomet. Chem., 2011, 696, 1659; CrossRef q) N. Kabay and Y. Goek, Inorg. Chem. Commun., 2011, 14, 1880; CrossRef r) M. Mayukh, C. W. Lu, E. Hernandez, and D. V. McGrath, Chem. Eur. J., 2011, 17, 8472. CrossRef
11. E. M. Maya, C. García, E. M. García-Frutos, P. Vázquez, and T. Torres, J. Org. Chem., 2000, 65, 2733; CrossRef A. de la Escosura, M. V. Martínez-Díaz, D. M. Guldi, and T. Torres, J. Am. Chem. Soc., 2006, 128, 4112; CrossRef A. de la Escosura, M. V. Martínez-Díaz, and T. Torres, J. Org. Chem., 2008, 73, 1475. CrossRef
12. B. del Rey, U. Keller, T. Torres, G. Rojo, F. Agulló-López, S. Nonell, C. Martí, S. Brasselet, I. Ledoux, and J. Zyss, J. Am. Chem. Soc., 1998, 120, 12808. CrossRef
13. T. Fukuda, S. Masuda, M. Wahadoszamen, N. Ohta, and N. Kobayashi, Daiton Trans., 2009, 6065.
14. N. Kobayashi, R. Higashi, B. C. Titeca, F. Lamote, and A. Ceulemans, J. Am. Chem. Soc., 1999, 121, 12018. CrossRef
15. K. Kitahara, T. Asano, K. Hamano, S. Tokita, and H. Nishi, J. Heterocycl. Chem., 1990, 27, 2219. CrossRef
16. D. K. P. Ng, Y.-O. Yeung, W. K. Chan, and S.-C. Yu, Tetrahedron Lett., 1997, 38, 6701; CrossRef Y.-O. Yeung, R. C. W. Liu, W.-F. Law, J. Jiang, and D. K. P. Ng, Tetrahedron, 1997, 53, 9087; CrossRef M. T. M. Choi, P. P. S. Li, and D. K. P. Ng, Tetrahedron, 2000, 56, 3881. CrossRef
17. C. Loosli, C. Jia, S.-X. Liu, M. Haas, M. Dias, E. Levillain, A. Neels, G. Labat, A. Hauser, and S. Decurtins, J. Org. Chem., 2005, 70, 4988; CrossRef C. A. Donders, S.-X. Liu, C. Loosli, L. Sanguinet, A. Neels, and S. Decurtins, Tetrahedron, 2006, 62, 3543; CrossRef S. Delahaye, C. Loosli, S.-X. Liu, S. Decurtins, G. Labat, A. Neels, A. Loosli, T. R. Ward, and A. Hauser, Adv. Funct. Mater., 2006, 16, 286. CrossRef
18. J. Nakayama and A. Ishii, Adv. Heterocycle. Chem., 2000, 77, 221; CrossRef K. Kobayashi, E. Koyama, M. Goto, C. Noda, and N. Furukawa, Chem. Commun., 2000, 1667. CrossRef
19. T. K. Hansen, T. Jørgensen, P. C. Stein, and J. Becher, J. Org. Chem., 1992, 57, 6403. CrossRef
20. R. Wang, W. Liu, Y. Chen, J.-L. Zuo, and X.-Z. You, Dyes Pigments, 2009, 81, 40. CrossRef
21. K. Sakamoto, E. Ohno-Okuma, T. Kato, and H. Soga, J. Porphyrins, Phthalocyanines, 2010, 14, 47. CrossRef
22. G. Mbambisa, P. Tau, E. Tntunes, and T. Nyokong, Polyhedron, 2007, 26, 5355; CrossRef G. Mbambisa and T. Nyokong, Polyhedron, 2008, 27, 2977; CrossRef T. B. Ogunbayo and T. Nyokong, Polyhedron, 2009, 28, 2710; CrossRef V. Chauke and T. Nyokong, Inorg. Chem. Acta, 2010, 363, 3662; CrossRef T. B. Ogunbayo and T. Nyokong, J. Mol. Catal. A: Chem., 2011, 337, 68. CrossRef
23. N. Kobayashi, T. Furuyama, and K. Satoh, J. Am. Chem. Soc., 2011, 133, 19642. CrossRef
24. A. N. Cammidge, C.-H. Tseng, I. Chambrier, D. L. Hughes, and M. J. Cook, Tetrahedron Lett., 2009, 50, 5254. CrossRef
25. E. Ohno-Okumura, K. Sakamoto, T. Kato, T. Hatano, K. Fukui, T. Karatsu, A. Kitamura, and T. Urano, Dyes and Pigments, 2002, 53, 57. CrossRef
26. O. V. Balakireva, V. E. Maizlish, and G. P. Shaposhnikov, Russian Journal of General Chemistry, 2003, 73, 292; CrossRef K. A. Volkov, G. V. Avramenko, V. M. Negrimovskii, and E. A. Luk’yanets, Russian Journal of General Chemistry, 2007, 77, 1108; CrossRef K. A. Volkov, V. M. Negrimovskii, G. V. Avramenko, and E. A. Luk’yanets, Russian Journal of General Chemistry, 2008, 78, 1794. CrossRef
27. C. S. Velázquez, W. E. Broderick, M. Sabat, A. G. M. Barrett, and B. M. Hoffman, J. Am. Chem. Soc., 1990, 112, 7408; CrossRef C. S. Velázquez, G. A. Fox, W. E. Broderick, K. A. Anderson, O. P. Anderson, A. G. M. Barrett, and B. M. Hoffman, J. Am. Chem. Soc., 1992, 114, 7416. CrossRef
28. G. Bähr and G. Schleitzer, Chem. Ber., 1955, 88, 1771; CrossRef G. Bähr and G. Schleitzer, Chem. Ber., 1957, 90, 438. CrossRef
29. R. Hou, C. Jiang, and B. Yin, Heterocycles, 2010, 81, 717. CrossRef
30. R. Hou, L. Jin, and B. Yin, Inorg. Chem. Commun., 2009, 12, 739; CrossRef R. Hou, B. Li, K. Zhong, H. Li, L.-Y. Jin, and B. Yin, Eur. J. Org. Chem., 2012, 1138. CrossRef
31. G. C. Papavassiliou, G. A. Mousdis, S. Y. Yiannopoulos, V. C. Kakoissis, and J. S. Zambounis, Synth. Metals, 1988, 27, B373; CrossRef T. Chen, C. Wang, Z. Cong, B. Yin, and K. Imafuku, Heterocycles, 2005, 65, 187; CrossRef M. Hasegawa, K. Takeda, Y. Kuwatani, M. Yoshida, H. Matsuyama, and M. Iyoda, J. Sulfur Chem., 2009, 30, 301. CrossRef
32. F. Leng, R. Hou, L. Jin, B. Yin, and R.-G. Xiong, J. Porphyrins, Phthalocyanines, 2010, 14, 108. CrossRef
33. T. Kimura, T. Suzuki, Y. Takaguchi, A. Yomogita, T. Wakahara, and T. Akasaka, Eur. J. Org. Chem., 2006, 1262. CrossRef
34. M. Kumada, K. Tamao, and K. Sumitani, Org. Synth., 1978, 58, 127.
35. a) T. Kimura, D. Watanabe, and T. Namauo, Heterocycles, 2008, 76, 1023; CrossRef b) T. Kimura, T. Namauo, K. Amano, N. Takahashi, Y. Takaguchi, T. Hoshi, and N. Kobayashi, J. Porphyrins, Phthalocyanines, 2011, 15, 547. CrossRef
36. S. Ogawa, M. Sugawara, Y. Kawai, S. Niizuma, T. Kimura, and R. Sato, Tetrahedron Lett., 1999, 40, 9101. CrossRef
37. T. Kimura, K. Tsujimura, S. Mizusawa, S. Ito, Y. Kawai, S. Ogawa, and R. Sato, Tetrahedron Lett., 2000, 41, 1801; CrossRef T. Kimura, S. Mizusawa, A. Yoneshima, S. Ito, K. Tsujimura, T. Yamashita, Y. Kawai, S. Ogawa, and R. Sato, Bull. Chem. Soc. Jpn., 2002, 75, 2647. CrossRef
38. T. Kimura, T. Obonai, T. Nozaki, K. Matsui, T. Namauo, A. Yamakawa, and Y. Takaguchi, Heterocycles, 2010, 80, 183. CrossRef
39. I. G. Abramov, A. V. Smirnov, S. A. Ivanovsky, M. B. Abramova, and V. V. Plakhtinskii, Heterocycles, 2001, 55, 1161; CrossRef I. G. Abramov, A. V. Smirnov, S. A. L. S. Kalandadze, V. N. Sakharov, A. V. Sapegin, and V. V. Plakhtinskii, Kimiya i Khimicheskaya Tekhnologiya, 2004, 47, 18.
40. V. M. Negrimovsky, V. M. Derkacheva, E. A. Luk’yanets, A. Weitemeyer, D. Wöhrle, and G. Schneider, Phosphorus Sulfur Silicon Relat. Elem., 1995, 104, 161. CrossRef
41. Y. Gök, H. Kantekin, and İ. Değirmencioğlu, Supramolecular Chem., 2003, 15, 335; CrossRef A. Bilgin and Y. Gök, Supramolecular Chem., 2006, 18, 491; CrossRef M. N. Mısır, Y. Gök, and H. Kantekin, J. Organomet. Chem., 2007, 692, 1451; CrossRef H. Kantekin, G. Dilber, and A. Nas, J. Organomet. Chem., 2010, 695, 1210; CrossRef E. T. Saka, N. Yıldırım, and H. Kantekin, Heteroat. Chem., 2010, 21, 456; CrossRef A. Nas, E. Ç. Kaya, H. Kantekin, A. Sökmen, and V. Çakır, J. Organomet. Chem., 2011, 696, 1659. CrossRef
42. a) C. S. Velázquez, T. F. Baumann, M. M. Olmstead, H. Hope, A. G. M. Barrett, and B. M. Hoffman, J. Am. Chem. Soc., 1993, 115, 9997; CrossRef b) T. F. Baumann, M. S. Nasir, J. W. Sibert, A. J. P. White, M. M. Olmstead, D. J. Williams, A. G. M. Barrett, and B. M. Hoffman, J. Am. Chem. Soc., 1996, 118, 10479; CrossRef c) T. F. Baumann, A. G. M. Barrett, and B. M. Hoffman, Inorg. Chem., 1997, 36, 5661; CrossRef d) D. P. Goldberg, S. L. J. Michel, A. J. P. White, D. J. Williams, A. G. M. Barrett, and B. M. Hoffman, Inorg. Chem., 1998, 37, 2100; CrossRef e) S. L. J. Michel, D. P. Goldberg, C. Stern, A. G. M. Barrett, and B. M. Hoffman, J. Am. Chem. Soc., 2001, 123, 4741. CrossRef
43. M. J. Fucheter, B. M. Hoffman, and A. G. M. Barrett, J. Org. Chem., 2005, 70, 5086. CrossRef
44. T. Kimura, N. Kanota, K. Matsui, I. Tanaka, T. Tsuboi, Y. Takaguchi, A. Yomogita, T. Wakahara, S. Kuwahara, F. Nagatsugi, and T. Akasaka, Inorg. Chem., 2008, 47, 3577. CrossRef
45. T. Kimura, T. Namauo, A. Yamakawa, and Y. Takaguchi, Heterocycles, 2009, 79, 1081. CrossRef
46. a) N. Kobayashi, R. Kondo, S. Nakajima, and T. Osa, J. Am. Chem. Soc., 1990, 112, 9640; CrossRef b) N. Kobayashi, T. Ishizaki, K. Ishii, and H. Konami, J. Am. Chem. Soc., 1999, 121, 9096; CrossRef c) A. Weitemeyer, H. Kliesch, and D. Wöhrle, J. Org. Chem., 1995, 60, 4900. CrossRef
47. W. J. Youngblood, J. Org. Chem., 2006, 71, 3345. CrossRef
48. a) C. C. Leznoff and T. W. Hall, Tetrahedron Lett., 1982, 23, 3023; CrossRef b) D. Wöhrle and G. Krawczyk, Polymer Bull., 1986, 15, 193; c) C. C. Leznoff, P. I. Svirskaya, B. Khouw, R. L. Cerny, P. Seymour, and A. B. P. Lever, J. Org. Chem., 1991, 56, 82; CrossRef d) A. Hirth, A. K. Sobbi, and D. Wöhrle, J. Porphyrins Phthalocyanines, 1997, 1, 275. CrossRef
49. T. Kimura, T. Obonai, and T. Namauo, Heterocycles, 2009, 77, 755. CrossRef
50. J. L. Segura and N. Martín, Angew. Chem. Int. Ed., 2001, 40, 1372; CrossRef M. Iyoda, M. Hasegawa, and Y. Miyake, Chem. Rev., 2004, 104, 5085; CrossRef J. O. Jeppesen, M. B. Nielsen, and J. Becher, Chem. Rev., 2004, 104, 5115; CrossRef N. Martín, L. Sánchez, M. Á. Herranz, B. Illescas, and D. M. Guldi, Acc. Chem. Res., 2007, 40, 1015. CrossRef
51. a) M. A. Blower, M. R. Bryce, and W. Devonport, Adv. Mater., 1996, 63; CrossRef b) C. Wang, M. R. Bryce, A. S. Batsanov, C. F. Stanley, A. Beeby, and J. A. K. Howard, J. Chem. Soc., Perkin Trans. 2, 1997, 1671. CrossRef
52. M. J. Cook, G. Cooke, and A. Jafari-Fini, J. Chem. Soc., Chem. Commun., 1996, 1925.
53. C. Wang, M. R. Bryce, A. S. Batsanov, and J. A. K. Howard, Chem. Eur. J., 1997, 3, 1679. CrossRef
54. J. Sly, P. Kasák, E. Gomar-Nadal, C. Rovira, L. Górriz, P. Thordarson, D. B. Amabilino, A. E. Rowan, and R. J. M. Nolte, Chem. Commun., 2005, 1255. CrossRef
55. R. Hou, H. Qiu, T. Chen, and B. Yin, Heterocycles, 2009, 78, 1799. CrossRef
56. R. Hou, C. Jiang, T. Chen, L.-Y. Jin, and B. Yin, Heterocycles, 2011, 83, 1859. CrossRef
57. C. Farren, C. A. Christensen, S. FitzGerald, M. R. Bryce, and A. Beeby, J. Org. Chem., 2002, 67, 9130. CrossRef
58. T. Kimura, N. Takahashi, T. Tajima, and Y. Takaguchi, Heterocycles, 2012, 84, 333. CrossRef
59. T. Kimura, D. Watanabe, and T. Namauo, Heteroat. Chem., 2011, 22, 605. CrossRef
60. T. Kimura, N. Takahashi, T. Tajima, and Y. Takaguchi, Heterocycles, 2012, 86, 679. CrossRef
61. F. Wudl, G. M. Smith , and E. J. Hufnagel, J. Chem. Soc., Chem. Commun., 1970, 1453.
62. M. Kimura, S. Otsuji, J. Takizawa, Y. Tatewaki, T. Fukawa, and H. Shirai, Chem. Lett., 2010, 39, 812. CrossRef
63. J. Becher, T. Brimert, J. O. Jeppesen, J. Z. Pedersen, R. Zubarev, T. Bjørnholm, N. Reitzel, T. R. Jensen, K. Kjaer, and E. Levillain, Angew. Chem. Int. Ed., 2001, 40, 2497. CrossRef
64. H. Li, J. O. Jeppesen, E. Levillain, and J. Becher, Chem. Commun., 2003, 846. CrossRef
65. K. A. Nielsen, J. O. Jeppesen, E. Levillain, and J. Becher, Angew. Chem. Int. Ed., 2003, 42, 187.
66. K. A. Nielsen, W.-S. Cho, J. Lyskawa, E. Levillian, V. M. Lynch, J. L. Sessler, and J. O. Jeppesen, J. Am. Chem. Soc., 2006, 128, 2444. CrossRef
67. S. Sadaike, K. Takimiya, Y. Aso, and T. Otsubo, Tetrahedron Lett., 2003, 44, 161. CrossRef
68. F. Leng, R. Hou, L. Jin, B. Yin, and R.-G. Xiong, J. Porphyrins, Phthalocyanines, 2010, 14, 108. CrossRef
69. P. A. Stuzhin and C. Ercolani, in The Porphyrin Handbook, Vols. 15 (ed. by K. M. Kadish, K. M. Smith, and R. Guilard) ACADEMIC PRESS, San Diego, 2003, 263.
70. R. P. Linstead, E. G. Noble, and J. M. Wright, J. Chem. Soc., 1937, 911. CrossRef
71. a) M. P. Cava and G. E. M. Husbands, J. Am. Chem. Soc,. 1969, 91, 3952; CrossRef b) M. D. Glick and R. E. Cook, Acta Crystallogr. Sect. B, 1972, 28, 1336; CrossRef c) S. Yoneda, K. Ozaki, T. Inoue, and A. Sugimoto, J. Am. Chem. Soc., 1985, 107, 5801; CrossRef d) A. Ishii, J. Naklayama, J. Kazami, Y. Ida, T. Nakamura, and M. Hoshino, J. Org. Chem., 1991, 56, 78; CrossRef e) R. A. Amaresh, M. V. Lakshmikantham, J. W. Baldwin, M. P. Cava, R. M. Metzger, and R. D. Rogers, J. Org. Chem., 2002, 67, 2453. CrossRef
72. a) D. M. Knawby and T. M. Swager, J. Mater. Chem., 1997, 9, 535; CrossRef b) E. Miyazaki, A. Kaku, H. Mori, M. Iwatani, and K. Takimiya, J. Mater. Chem., 2009, 19, 5913; CrossRef c) J. A. Bilton and R. P. Linstead, J. Chem. Soc., 1937, 922. CrossRef
73. a) M. J. Cook and A. Jafari-Fini, J. Mater. Chem., 1997, 7, 5; CrossRef b) M. J. Cook and A. Jafari-Fini, Tetrahedron, 2000, 56, 4085; CrossRef c) V. N. Nemykin, A. E. Polshina, and N. Kobayashi, Chem. Lett., 2000, 1236. CrossRef
74. D. Vegh, M. Landl, R. Pavlovicova, H. Kuzmany, and P. Zalupsky, Khimiya Geterotsiklicheskikh Soedinenii, 1995, 1409.
75. T. Kimura, T. Iwama, T. Namauo, E. Suzuki, T. Fukuda, N. Kobayashi, T. Sasamori, and N. Tokitoh, Eur. J. Inorg. Chem., 2011, 888. CrossRef
76. P. A. Stuzhin, E. M. Bauer, and C. Ercolani, Inorg. Chem., 1998, 38, 1533. CrossRef
77. S. M. Baum, A. A. Trabanco, A. G. Montalban, A. S. Micallef, C. Zhong, H. G. Meunier, K. Suhling, D. Phillips, A. J. P. White, D. J. Williams, A. G. M. Barrett, and B. M. Hoffman, J. Org. Chem., 2003, 68, 1665. CrossRef
78. M. P. Donzello, C. Ercolani, and P. A. Stuzhin, Coord. Chem. Rev., 2006, 250, 1530. CrossRef
79. D. Lelièvre, L. Bosio, J. Simon, J.-J. André, and F. Bensebaa, J. Am. Chem. Soc., 1992, 114, 4475; CrossRef N. Kobayashi, H. Lam, W. A. Nevin, P. Janda, C. C. Leznoff, T. Koyama, A. Monden, and H. Shirai, J. Am. Chem. Soc., 1994, 116, 879; CrossRef M. Calvete and M. Hanack, Eur. J. Org. Chem., 2003, 2080; CrossRef N. Kobayashi and H. Ogata, Eur. J. Inorg. Chem., 2004, 906. CrossRef
80. S. G. Makarov, O. N. Suvorova, C. Litwinski, E. A. Ermilov, B. Röder, O. Tsaryova, T. Dülcks, and D. Wöhrle, Eur. J. Inorg. Chem., 2007, 546; CrossRef S. G. Makarov, A. V. Piskunov, O. N. Suvorova, G. Schnurpfeil, G. A. Domrachev, and D. Wöhrle, Chem. Eur. J., 2007, 13, 3227. CrossRef
81. Y. Asano and N. Kobayashi, Tetrahedron Lett., 2004, 45, 9577. CrossRef
82. V. L. Goedken, G. Dessy, C. Ercolani, V. Fares, and L. Gastaldi, Inorg. Chem., 1985, 24, 991. CrossRef
83. A. Deronzier and J. M. Latour, Nouv. J. Chim., 1984, 8, 393.
84. M. Barthel and M. Hanack, J. Porphyrins Phthalocyanines, 2000, 4, 635; CrossRef M. Barthel, D. Dini, S. Vagin, and M. Hanack, Eur. J. Org. Chem., 2002, 3756. CrossRef
85. N. Kobayashi and A. Muranaka, Chem. Commun., 2000, 1855; N. Kobayashi, A. Muranaka, and K. Ishii, Inorg. Chem., 2000, 39, 2256; CrossRef A. Muranaka, K. Yoshida, Y. Akagi, H. Naka, M. Uchiyama, Y. Kondo, and N. Kobayashi, Tetrahedron Lett., 2008, 49, 5084. CrossRef
86. A. Muranaka, M. Okuda, N. Kobayashi, K. Somers, and A. Ceulemans, J. Am. Chem. Soc., 2004, 126, 4596; CrossRef A. Muranaka, K. Yoshida, T. Shoji, N. Moriichi, S. Masumoto, T. Kanda, Y. Ohtake, and N. Kobayashi, Org. Lett., 2006, 8, 2447. CrossRef
87. Y. Chen, M. E. El-Khouly, M. Sasaki, Y. Araki, and O. Ito, Org. Lett., 2005, 7, 1613. CrossRef
88. T. Kimura, K. Amano, A. Yamamoto, and T. Namauo, Heterocycles, 2011, 82, 1229. CrossRef
89. T. Kimura, J. Kumasaka, and T. Namauo, Eur. J. Org. Chem., 2008, 5079. CrossRef