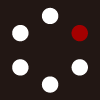
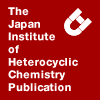
HETEROCYCLES
An International Journal for Reviews and Communications in Heterocyclic ChemistryWeb Edition ISSN: 1881-0942
Published online by The Japan Institute of Heterocyclic Chemistry
e-Journal
Full Text HTML
Received, 19th June, 2012, Accepted, 20th July, 2012, Published online, 31st July, 2012.
DOI: 10.3987/COM-12-S(N)44
■ Catalytic Asymmetric Synthesis of (S)-Laudanosine by Hydroamination
Tokutaro Ogata, Tetsutaro Kimachi, Ken-ichi Yamada, Yasutomo Yamamoto, and Kiyoshi Tomioka*
Department of Medicinal Chemistry, Doshisha Women’s College of Liberal Arts, Kodo, Kyotanabe, Kyoto 610-0395, Japan
Abstract
Lithium amide–chiral bisoxazoline-catalyzed asymmetric intramolecular hydroamination was examined with respect to the structural variants of starting aminoalkenes. Substituents on the nitrogen and olefin of aminoalkenes were found to be important factors affecting reaction efficiency as well as enentioselectivity in the production of chiral tetrahydroisoquinolines and isoindolines. The catalytic asymmetric total synthesis of (S)-laudanosine highlights the utility of the asymmetric hydroamination.INTRODUCTION
Asymmetric intramolecular hydroamination of aminoalkenes is a highly versatile C–N bond forming reaction useful for the synthesis of nitrogen-containing chiral heterocycles.1 We have already reported the chiral bisoxazoline (box) 3a–lithium diisopropylamide (LDA)-catalyzed asymmetric intramolecular hydroamination of aminoalkenes 1a giving 1-benzyltetrahydroisoquinoline 2a with 84% ee in 97% yield (Scheme 1).2,3,4 1-Benzylisoindoline 2b with excellent 91% ee was also synthesized in 99% yield by the catalysis of chiral bisoxazoline 3b–LDA. In this paper we describe the extension of this intramolecular hydroamination method to the cyclization of other type of aminoalkenes and further application to the asymmetric total synthesis of representative isoquinoline alkaloid (S)-laudanosine.
RESULTS AND DISCUSSION
The asymmetric intramolecular hydroamination of aminoalkenes 1a and 1b was effectively catalyzed by chiral box 3–lithium amide catalyst.2 The structural features of aminoalkenes 1a and 1b are (a) an N-methyl group, (b) an aromatic ring connecting an amino group and an olefin, and (c) phenyl group on a terminal carbon of olefin (Figure 1). To verify whether these features are essential for the reaction, other types of aminoalkenes 1c–f were prepared and examined their possibilities in the intramolecular hydroamination.
Synthesis of aminoalkenes 1c–1f
As shown in Scheme 2 N-PMB (4-methoxybenzyl) aminoalkene 1c and N-allyl aminoalkene 1d were prepared from the same aldehyde 4, which was prepared by the Heck reaction of commercially available 2-bromobenzaldehyde and styrene.5 The Henry reaction of 4 and following LiAlH4 reduction of nitroalkene 56 gave primary amine 6.7 Reductive 4-methoxybenzylation of 6 afforded N-PMB aminoalkene 1c. Reductive amination of 4 with allylamine gave 1d.8 The sequential treatment of benzaldehyde with vinylmagnesium bromide,9 Johnson–Claisen rearrangement,10 hydrolysis of ethyl ester 8, amidation of a carboxylic acid 9 with allylamine, and finally LiAlH4 reduction of amide 10 gave 1e. According to the reported procedure, 1f was synthesized starting from 2-bromobenzaldehyde 11 by the Wittig olefination.11 Halogen–lithium exchange of 12 and formylation with DMF gave 13, and then reductive amination with methylamine gave 1f.11c,d
Asymmetric hydroamination of aminoalkenes 1c–f
Substituents of amine were examined on the reaction efficiency and enantioselectivity (Figure 1, moiety (a) of 1ab). From the synthetic viewpoint, easily removable PMB and allyl groups were selected as a candidate (Table 1, entries 3 and 4). N-PMB aminoalkene 1c was treated with chiral box 3c and LDA in toluene at 0 °C to give cyclized tetrahydroisoquinoline derivative 2c in as high as 94% yield. However, enantioselectivity was only 11% ee (entry 3). Since the stereoselectivity of the corresponding N-methyl derivative 2a is 71% ee by using the same box 3c (entry 1),2 N-PMB group was unfavorable for this asymmetric reaction. On the other hand, the reaction of N-allyl aminoalkene 1d12 gave isoindoline derivative 2d with 83% ee in 98% yield (entry 4), which was almost same selectivity as that of N-methyl derivatives 2b2 (entries 2 and 4). These results indicated that methyl or allyl N-substituent led to the cyclization with better enantioselectivities.
Aromatic ring connecting secondary amine and olefin moieties (Figure 1, (b) of 1ab) was examined with respect to their effect on the reaction efficiency by the reaction of 1e in which no aromatic ring exists.
The hydroamination of 1e gave 2e in 90% yield; however, the enantioselectivity was only 18% ee (entry 5). This result indicated that the presence of aromatic ring was also important to achieve good enantioselectivity.
The terminal phenyl group of olefin, (c) moiety of 1ab in Figure 1, affected regioselectivity as well as enantioselectivity. The reaction of 1f, having no terminal phenyl group, afforded the expected 5-exo-cyclization product 2f as the major one in 33% yield with 43% ee, 6-endo cyclization product 14f in 7% yield, intermolecularly reacted dimer 15 in 28% yield, and recovered 1f in 19% yield (entry 6). These results indicated that a terminal phenyl group directs originally favored 5-exo cyclization (entries 2, 4 and 5) by sterically blocking attack at the terminal olefin carbon.
The absolute configuration of 2d was unambiguously determined by correlating with (S)-2b13 of the established configuration (Scheme 3). The sense of enantiofacial selectivity of N-allyl aminoalkene 1d is identical with that of N-methyl aminoalkene 1b.
Reaction pathway of intramolecular hydroamination
The plausible reaction pathway was shown in Scheme 4. The aminoalkene 1b was deprotonated by n-BuLi and/or LDA to give the corresponding lithium amide 18, to which chiral bisoxazoline 3 coordinated. Aminolithiation of 18 gave cyclized product 19, and subsequent protonation by i-Pr2NH 20 and/or 1b furnished hydroamination product 2b, and i-Pr2NLi 21 or 16. In the case that 19 was protonated with i-Pr2NH 20, catalytic cycle was accomplished by the deprotonation of 1b with LDA 21 to give 18 again. The possible stereoinduction step is kinetically controlled aminolithiation step, 18 to 19, of which C–Li is immediately converted to C–H by protonation with 20 and/or 1b.
Stereoinduction of intramolecular hydroamination
The cyclization would proceed through transition state A not through B to avoid the steric repulsion between the bulky alkyl group of 3 (shown as R in A) and the aromatic ring of aminoalkene 1b as shown in B to give (S)-product preferentially (Figure 2). The results of Table 1 supported this analysis; the aromatic rings of 1b would contribute to the steric repulsion with the alkyl group of 3 in disfavored transition state B, and thus the absence of aromatic ring in 1e reduces superiority of A over B to result in poorer enantioselectivities (Table 1, entries 5 and 6). Moreover, the bulky N-protecting group of 1c caused another steric repulsion against the alkyl group of 3 as shown in C, which also resulted in poor enantioselectivity (Table 1, entry 3). A terminal phenyl group contributes to the selectivity of 5-exo/6-endo cyclization; in the reaction of 1f, both 5-exo cyclization product 2f (33%) and 6-endo cyclization product 14f (7%) were obtained, whereas 1b, 1d and 1e gave only 5-exo cyclization products. The absence of terminal phenyl group reduces the steric hindrance at the terminal of the olefin, and consequently 6-endo cyclization product 14f and dimer 15 were produced. Apparently, this chiral bisoxazoline–lithium amide catalyst system is suitable for the asymmetric synthesis of 1-benzyltetrahydroisoquinolines and 1-benzylisoindolines.
Asymmetric total synthesis of (S)-laudanosine
The asymmetric hydroamination was applicable for the total synthesis of opium alkaloid (S)-laudanosine (29).14 Commercially available 6-bromoveratrylaldehyde 22 and 3,4-dimethoxystyrene 23 were coupled by the Heck reaction to give stilbene 24 in 80% yield (Scheme 5). Henry reaction gave nitroalkene 25, whose LiAlH4 reduction gave primary amine 26. Formylation followed by LiAlH4 reduction gave secondary N-methylamine 28, a cyclization precursor of (S)-laudanosine 29. The hydroamination reaction of 28 was carried out at –30 °C due to its low solubility at temperature lower than –30 °C to give desired (S)-laudanosine 29 in 96% yield with 76% ee. Optically pure (S)-29 was obtained by recrystallization from hexane/ethyl acetate in 64% yield. Thus, catalytic asymmetric synthesis of optically pure (S)-laudanosine 29 was accomplished in 33% overall yield.
CONCLUSION
In conclusion, the substituents of aminoalkenes significantly affected the efficiency of the chiral bisoxazoline–lithium amide-catalyzed asymmetric hydroamination. This catalytic system was suitable for the preparation of chiral 1-benzyltetrahydroisoquinolines and 1-benzylisoindolines. Asymmetric total synthesis of (S)-laudanosine was achieved in 6 steps using catalytic asymmetric hydroamination as a key step.
EXPERIMENTAL
1H NMR (500 MHz) and 13C NMR (125 MHz) were measured in CDCl3. Chemical shift values were expressed in ppm relative to an internal reference of tetramethylsilane (0 ppm) in 1H NMR and CDCl3 (77.0 ppm) in 13C NMR. 13C peak multiplicity assignments were made based on DEPT data. IR spectroscopy of oil and solid samples were measured as neat liquid films and KBr pellets, respectively. Coupling constants were shown in Herz. Abbreviations are as follows: s, singlet; d, doublet; t, triplet; m, multiplet; br, broad. The wave-numbers of maximum absorption peaks of IR spectroscopy were presented in cm–1. Column chromatography was performed using silica gel as a stationary phase.
1-((E)-2-Nitrovinyl)-2-styrylbenzene (5)7: According to literature procedure,6 a solution of 45 (624 mg, 3 mmol) and NH4OAc (231 mg, 3 mmol) in dry MeNO2 (5.7 mL) was stirred at 100 °C for 1 h. After concentration, the residue was partitioned between AcOEt and water. The aqueous layer was extracted with AcOEt, and the combined organic extracts were washed with water and brine, dried over Na2SO4 and concentrated. Column chromatography (benzene/hexane 1/4-1/3) gave 5 (560 mg, 74%) as a bright red oil.
(E)-2-(2-Styrylphenyl)ethanamine (6)7: To a suspension of LiAlH4 (7.75 g, 204 mmol) in dry Et2O (100 mL) was added 5 (10.25 g, 40.8 mmol) in dry Et2O (250 mL) at 0 °C and the mixture was stirred for 1 h. The reaction was quenched with water (7.8 mL), 10% NaOH (7.8 mL) and water (20 mL). After filtration, the filtrate was dried over K2CO3 and concentrated. Column chromatography (hexane/EtOAc 3/1, then EtOH) gave 6 (6.32 g, 69 %) as a pale yellow oil.
N-(4-Methoxybenzyl)-2-(styrylphenyl)ethanamine (1c): A suspension of 6 (1.41 g, 6.32 mmol) and p-anisaldehyde (856 mg, 6.32 mmol) in water (10 mL) was stirred vigorously at rt for 3 h. To the mixture was added CHCl3 (20 mL) and it was stirred for 1 h at the same temperature. The mixture was extracted with CHCl3, and the combined organic extracts were washed with brine and dried over K2CO3. Concentration gave 2.4 g of imine as a pale yellow oil. To a suspension of LiAlH4 (932 mg, 25.3 mmol) in dry Et2O (5 mL) was added imine (2.4 g) in dry Et2O (4 mL) at 0 °C. The mixture was stirred for 1 h at 0 °C, and quenched with water (0.15 mL), 10% NaOH (0.15 mL) and water (0.45 mL) and then filtered through a Celite pad. The filtrate was dried over K2CO3 and concentrated. Column chromatography (AcOEt/hexane 1/5) gave 1c (1.87 g, 86%) as a pale yellow oil. 1H NMR: 1.50 (1H, br s), 2.89 (2H, t, J = 7.3), 2.99 (2H, t, J = 7.3), 3.75 (2H, s), 3.76 (3H, s), 6.80 (2H, d, J = 8.6), 6.99 (1H, d, J = 15.9), 7.18-7.40 (9H, m), 7.50 (2H, d, J = 8.6), 7.61 (1H, m). 13C NMR: 34.0, 50.0, 53.2, 55.2, 113.8, 126.0, 126.2, 126.65, 126.74, 127.68, 127.72, 128.7, 129.2, 130.1, 130.5, 136.4, 137.7, 137.9. IR: 3024, 2932, 2831, 1612, 1512. EIMS m/z: 343 (M+). Anal. Calcd for C24H25NO: C, 83.93; H, 7.34; N, 4.08. Found: C, 84.19; H, 7.46; N, 4.03.
(E)-N-(2-Styrylbenzyl)prop-2-en-1-amine (1d): To a solution of 4 (10.0 g, 48 mmol) in MeOH (100 mL) was added dropwise allylamine (5.4 mL, 72 mmol) at rt and the mixture was stirred for 1 h. Then the solution was cooled to 0 °C. To the solution was added portionwise NaBH4 (1.83 g, 48 mmol) over 5 min. The mixture was stirred for 1 h and then concentrated. After addition of water (200 mL), the whole was extracted with ether (200+100+100 mL). The combined organic layers were washed with brine and dried over K2CO3. Concentration and column chromatography (hexane/EtOAc 5/1-1/2) gave 1d (11.4 g, 95%) as a colorless oil. 1H NMR: 1.34 (1H, br s), 3.33 (2H, d, J = 6.0), 3.91 (2H, s), 5.13 (1H, dd, J = 10.2, 1.5), 5.23 (1H, dd, J = 17.0, 1.5), 5.96 (1H, m), 7.04 (1H, d, J = 16.0), 7.20-7.40 (6H, m), 7.48 (1H, d, J = 16.0), 7.53 (2H, d, J = 7.7), 7.62-7.65 (1H, m). 13C NMR: 50.9 (CH2), 52.0 (CH2), 116.2 (CH2), 125.9 (CH), 126.0 (CH), 126.7 (CH), 127.5 (CH), 127.6 (CH), 127.7 (CH), 128.7 (CH), 129.5, (CH), 130.7 (CH), 136.6 (C), 136.9 (CH), 137.6 (C), 137.7 (C). IR: 3325, 3063, 3024, 2816, 1597, 1496, 1450. EIMS m/z: 249 (M+). Anal. Calcd for C18H19N: C, 86.70; H, 7.68; N, 5.62. Found: C, 86.77; H, 7.69; N, 5.61.
(E)-N-Allyl-5-phenylpent-4-en-1-amine (1e)9,10: Under argon atmosphere, to a solution of benzaldehyde (5.1 mL, 50 mmol) in THF (72 mL) was added vinylmagnesium chloride (1.48 M, 41 mL, 60 mmol) at 0 °C. The solution was stirred for 1 h, diluted with Et2O (100 mL), and then quenched with satd NH4Cl (30 mL). The mixture was extracted with Et2O (50 + 50 mL) and the combined organic extracts were concentrated to give 7 (7.83 g) as pale yellow oil. To a solution of 7 in xylene (125 mL) were added HC(OEt)3 (34 mL, 250 mmol) and EtCO2H (0.5 g, 5 mmol) under argon atmosphere. The solution was heated at reflux for 2.5 h, and then diluted with AcOEt (400 mL). The whole was washed with satd NaHCO3 (100 mL), water (100 mL) and brine (100 mL), and then dried over Na2SO4. Concentration gave 8 (13.0 g) as a pale yellow oil. To a solution of 8 in dioxane (95 mL), MeOH (16 mL) and water (50 mL) was added KOH (8.4 g, 150 mmol). The whole was stirred for 1 h at rt and then concentrated to remove MeOH. The residue was diluted with Et2O (300 mL) and extracted with satd. aq. NaHCO3 (300 + 100 + 100 mL). The combined aqueous layers were acidified with 10% HCl and extracted with Et2O (500 + 200 + 100 mL). The combined organic extracts were concentrated to give 9 (5.9 g) as a white solid. To a solution of 9 in THF (125 mL) were added Et3N (5.0 mL, 44 mmol), ClCO2Et (4.5 mL, 44 mmol) and allylamine (5 mL, 67 mmol) at 0 °C under argon atmosphere. The mixture was stirred for 10 min at 0 °C and for 20 h at rt, and then quenched with satd. aq. NaHCO3 (50 mL). The whole was extracted with AcOEt (100 + 100 + 100 mL). The combined organic layers were washed with water (100 mL) and brine (100 mL), dried over Na2SO4, and concentrated to give 10 (7.9 g) as an orange oil. To a suspension of LiAlH4 (6.4 g, 168 mmol) in Et2O (100 mL) was added a solution of 10 in Et2O (20 mL) and THF (130 mL) at 0 °C under argon atmosphere. The reaction was stirred for 20 h at rt, and was quenched with water (6.4 mL), 10% NaOH (6.4 mL) and water (19.2 mL). After filtration, the filtrate was dried over K2CO3 and concentrated. Column chromatography (acetone/hexane 1/1) gave 1e (5.3 g, 5 steps 50%) as a pale yellow oil. 1H NMR: 1.21 (1H, br s), 1.68 (2H, tt, J = 7.3, 7.3), 2.26 (2H, dt, J = 7.3, 7.3), 2.67 (2H, t, J = 7.3), 3.26 (2H, d, J = 5.8), 5.08 (1H, d, J = 10.4), 5.17 (1H, d, J = 17.1), 5.91 (1H, m), 6.22 (1H, dt, J = 15.9, 7.3), 6.40 (1H, d, J = 15.9), 7.19 (1H, m), 7.27–7.35 (4H, m). 13C NMR: 29.7 (CH2), 30.7 (CH2), 48.9 (CH2), 52.5 (CH2), 115.7 (CH2), 125.9 (CH), 126.9 (CH), 128.5 (CH), 130.1 (CH), 130.4 (CH), 137.0 (CH), 137.8 (C). IR: 3364, 2924, 1450. Anal. Calcd for C14H19N: C, 83.53; H, 9.51; N, 6.96. Found: C, 83.43; H, 9.81; N, 6.78.
1-Bromo-2-vinylbenzene (12)11a: Under argon atmosphere, to a suspension of MePPh3Br (134 g, 375 mmol) in dry THF (335 mL) was added n-BuLi (1.6 N in hexane, 234 mL, 375 mmol) at 0 °C. The mixture was stirred for 2 h. A solution of 2-bromobenzaldehyde 11 (62.0 g, 335 mmol) in dry THF (335 mL) was added at 0 °C. The whole was stirred for 19 h at rt and quenched with brine and extracted with benzene. The organic layers were washed with brine, dried over Na2SO4 and concentrated. Hexane (1.7 L) was added to the residue and the mixture was stirred for 1 h at 50 °C and filtered off Ph3P=O. The filtrate was concentrated and then distilled (57 °C, 4 mmHg) to give 12 (35 g 57%) as a colorless oil. 1H NMR: 5.35 (1H, d, J = 11.0), 5.69 (1H, d, J = 17.4), 7.05 (1H, dd, J = 17.4, 11.0), 7.09 (1H, t, J = 7.7), 7.25 (1H, t, J = 7.7), 7.54 (2H, d, J = 7.7). IR: 3063, 2361, 1836, 1628, 1589, 1466, 1412. EIMS m/z: 184 (M+2), 182 (M+), 103 (M+ – Br).
2-Vinylbenzaldehyde (13)11a: Under argon atmosphere, to a solution of 12 (20.0 g, 109 mmol) in dry THF (140 mL) was added n-BuLi (1.6 N in hexane, 69 mL, 110 mmol) at –78 °C. The mixture was stirred for 0.5 h at the same temperature, and then treated with Me2NCHO (8.6 mL, 111 mmol) in dry THF (40 mL). The mixture was stirred for 1 h at –78 °C and for 1 h at rt, and then quenched with brine at 0 °C and extracted with CHCl3. The organic layer was washed with brine, dried over Na2SO4 and concentrated. Distillation (58 °C, 0.3 mmHg) gave 13 (9.8 g, 68%) as a pale yellow oil. 1H NMR: 5.51 (1H, d, J = 11.0), 5.70 (1H, d, J = 17.4), 7.40-7.47 (1H, m), 7.54 (1H, dd, J = 11.0, 17.4), 7.56-7.61 (2H, m), 7.83 (1H, d, J = 7.4), 10.29 (1H, s). 13C NMR: 119.7, 127.7, 128.2, 131.5, 133.1, 133.6, 134.1, 140.8, 192.7. IR: 3062, 2846, 2738, 1697, 1596, 1566, 1481, 1411, 1296, 1203. EIMS m/z: 132 (M+), 103 (M+ – CHO).
N-Methyl-1-(2-vinylphenyl)methanamine (1f)11c,d: A 40% aqueous solution of MeNH2 (20.8 mL, 240 mmol) was added to a solution of 13 (10.6 g, 80 mmol) in MeOH (180 mL). After stirring at rt for 4 h, NaBH4 (3.04 g, 80 mmol) was added at 0 °C. The mixture was stirred for 1 h at rt and quenched with brine and concentrated. The resulting aqueous residue was extracted with CHCl3. The organic layer was washed with brine, dried over K2CO3 and concentrated. Distillation (62 °C, 0.4 mmHg) gave 1f (12.7 g, 68%) as a colorless oil. 1H NMR: 1.42 (1H, br s), 2.47 (3H, s), 3.79 (2H, s), 5.33 (1H, dd, J = 1.2, 11.0), 5.69 (1H, dd, J = 1.2, 17.4), 7.06 (1H, dd, J = 11.0, 17.4), 7.15-7.35 (3H, m), 7.52 (1H, d, J = 6.7). 13C NMR: 36.2 (CH3), 53.7 (CH2), 115.9 (CH2), 125.8 (CH), 127.4 (CH), 127.7 (CH), 129.7 (CH), 134.2 (CH), 136.9 (C), 137.2 (C). IR: 3325, 3063, 3024, 2932, 2847, 2793, 1628, 1481, 1442. EIMS m/z: 147 (M+), 132 (M+ – CH3).
General procedure of asymmetric hydroamination. 2-Allyl-1-benzylisoindoline (2d) (Table 1, entry 4): To a solution of 3c (0.53 g, 2.0 mmol) and i-Pr2NH (0.64 mL, 4.5 mmol) in toluene (40 mL) was added n-BuLi (1.57 M in hexane, 0.72 mL, 1 mmol) over 1 min at –78 °C under argon atmosphere. After stirring for 10 min, 1d (1.24 g, 5.0 mmol) in toluene (10 mL) was added over 10 min. The brown solution was stirred for 2 h at –40 °C, and then quenched with satd NH4Cl (10 mL) and 10% K2CO3 (10 mL). The separated aqueous layer was extracted with Et2O (50 + 25 + 25 mL). The combined organic layers were washed with brine (30 mL), dried over K2CO3, and concentrated. Column chromatography (AcOEt/hexane 1/12) gave 2d8 (1.22 g, 98%) as a pale yellow oil of [α]25D + 44.4 (c 1.08, CHCl3). Ee was determined by HPLC analysis to be 83% (Daicel Chiralpak AS-H×2, hexane/i-PrOH/Et3N 200/1/0.1, 1 mL/min, 254 nm, major 8.4 min and minor 8.9 min).
2-(4-Methoxybenzyl)-1-benzyl-1,2,3,4-tetrahydroisoquinoline (2c): a colorless oil. [α]25D +0.48 (c 1.04, CHCl3). 11% ee (HPLC, Daicel Chiralcel OJ-H, hexane/i-PrOH 100/1, 1.0 mL/min, 254 nm, minor 25.6 min and major 35.4 min). 1H NMR: 2.52-2.56 (1H, m), 2.83-2.89 (2H, m), 2.94-3.00 (1H, m), 3.09-3.13 (1H, m), 3.30-3.36 (1H, m), 3.65 (1H, d, J = 13.2), 3.70 (1H, d, J = 13.2), 3.79 (3H, s), 3.87 (1H, m), 6.74 (2H, d, J = 8.6), 6.83-6.84 (1H, m), 7.00 (2H, d, J = 8.6), 7.06-7.15 (5H, m), 7.19-7.26 (3H, m). 13C NMR: 24.4, 42.4, 42.8, 55.2, 57.2, 62.4, 113.5, 125.5, 125.8, 126.1, 127.9, 128.3, 129.0, 129.8, 131.5, 134.7, 138.2, 140.3, 158.5. IR: 3024, 2932, 2831, 1612, 1512. FABMS m/z: 344 (M+H+). Anal. Calcd for C24H25NO: C, 83.93; H, 7.34; N, 4.08. Found: C, 84.15; H, 7.41; N, 3.98.
1-Allyl-2-benzylpyrrolidine (2e): a colorless oil. [α]25D −13.6 (c 1.15, CHCl3). 18% ee (HPLC, Daicel Chiralcel OJ-H, hexane/i-PrOH 100/1, 0.5 mL/min, 254 nm, major 4.9 min and minor 4.4 min). 1H NMR: 1.54 (1H, m), 1.64 (1H, m), 1.67-1.78 (2H, m), 2.21 (1H, m), 2.45 (1H, dd, J = 13.1, 9.5), 2.56 (1H, m), 2.89 (1H, dd, J = 13.2, 7.6), 3.05 (1H, dd, J = 13.1, 4.0), 3.12 (1H, m), 3.55 (1H, dd, J = 13.2, 5.5), 5.12 (1H, d, J = 10.1), 5.22 (1H, dd, J = 17.1, 1.2), 5.96 (1H, m), 7.15-7.20 (3H, m), 7.25-7.29 (2H, m). 13C NMR: 21.5 (CH2), 30.2 (CH2), 40.6 (CH2), 53.8 (CH2), 57.1 (CH2), 65.2 (CH), 116.6 (CH2), 125.7 (CH), 128.0 (CH), 129.0 (CH), 135.9 (CH), 140.0 (C). IR: 2963, 2793, 1643, 1450, 1157, 1119, 995, 918, 748. EIMS: 201 (M+). Anal. Calcd for C14H19N: C, 83.53; H, 9.51; N, 6.96. Found: C, 83.81; H, 9.63; N, 6.93.
1,2-Dimethylisoindoline (2f)15 a colorless oil. [α]25D +19.0 (c 0.66, CHCl3). 43% ee (HPLC, Daicel Chiralcel AD-H, hexane/i-PrOH 100/1, 0.5 mL/min, 254 nm, major 11.2 min and minor 12.6 min). 1H NMR: 1.43 (3H, d, J = 6.1), 2.57 (3H, s), 3.64 (2H, m), 4.24 (1H, d, J = 11.6), 7.09-7.14 (1H, m), 7.17-7.25 (3H, m). 13C NMR: 18.0 (CH3), 39.8 (CH3), 60.5 (CH2), 65.3 (CH), 121.6 (CH), 122.0 (CH), 126.8 (CH x 2), 139.4 (C), 144.9 (C). IR: 3387, 3040, 2770, 1697, 1458. EIMS m/z: 147 (M+), 132 (M+ – CH3).
2-Methyl-1,2,3,4-tetrahydroisoquinoline (14f)16: a colorless oil. 1H NMR: 2.46 (3H, s), 2.68 (2H, t, J = 6.2), 2.93 (2H, t, J = 6.2), 3.58 (2H, s), 7.00-7.03 (1H, m), 7.09-7.16 (3H, m). 13C NMR: 29.1 (CH2), 46.1 (CH3), 52.8 (CH2), 57.9 (CH2), 125.6 (CH), 126.0 (CH), 126.4 (CH), 128.6 (CH), 133.8 (C), 134.7 (C).
IR: 3364, 3024, 2924, 2777, 1705, 1589, 1458. EIMS m/z: 147 (M+).
N-Methyl-2-(2-((methylamino)methyl)phenyl)-N-(2-vinylbenzyl)ethanamine (15): a colorless oil. 1H NMR: 1.58 (1H, br s), 2.30 (3H, s), 2.44 (3H, s), 2.60-2.64 (2H, m), 2.86-2.90 (2H, m), 3.57 (2H, s), 3.69 (2H, s), 5.25 (1H, m), 5.64 (1H, m), 7.09-7.53 (9H, m). 13C NMR: 30.2 (CH2), 36.2 (CH3), 42.0 (CH3), 53.2 (CH2), 58.9 (CH2), 60.0 (CH2), 115.1 (CH2), 125.6 (CH), 126.1 (CH), 127.1 (CH), 127.3 (CH), 128.3 (CH), 129.0 (CH), 129.8 (CH), 130.3 (CH), 134.7 (CH), 136.0 (C), 137.6 (C), 137.9 (C), 138.8 (C). IR: 3325, 3063, 3017, 2939, 2839, 2785, 1628, 1450. HRMS-FAB m/z: [M+H]+ calcd for C20H27N2, 295.2169; found, 295.2177.
Determination of the absolute configuration of 2d.
(S)-1-Benzylisoindoline (16): Under argon atmosphere, to a solution of 2d (500 mg, 2.0 mmol, 83% ee) in MeCN/water (4/1, 25 mL) was added RhCl(PPh3)3 (32 mg, 0.1 mmol). The mixture was heated at reflux for 2 h and diluted with AcOEt. To the mixture was added water (20 mL), and the whole was extracted with AcOEt (30+20+10 mL). The combined organic layers were washed with brine and dried over K2CO3. Concentration and column chromatography (hexane/AcOEt/Et3N 10/2/1) gave 168 mg (40% yield) of 1-benzylisoindoline 16 as a pale yellow oil. [α]25D −22.7 (c 1.00, CHCl3). 1H NMR: 1.94 (1H, br s), 2.87 (1H, dd, J = 13.6, 8.4), 3.13 (1H, dd, J = 13.6, 5.2), 4.15 (1H, d, J = 13.7), 4.20 (1H, d, J = 13.7), 4.65 (1H, dd, J = 8.4, 5.2), 7.09 (1H, d, J = 6.7), 7.17-7.24 (6H, m), 7.28-7.31 (2H, m). 13C NMR: 42.7 (CH2), 51.3 (CH2), 64.6 (CH), 122.4 (CH), 122.5 (CH), 126.3 (CH), 126.5 (CH), 127.0 (CH), 128.4 (CH), 129.3 (CH), 139.0 (C), 141.6 (C), 144.0 (C). IR: 3063, 3024, 2916, 2839, 1597, 1458. EIMS m/z: 209 (M+). Anal. Calcd for C15H15N: C, 86.08; H, 7.22; N, 6.69. Found: C, 85.88; H, 7.46; N, 6.71.
(S)-1-Benzylisoindoline-2-carbaldehyde (17): A solution of 16 (100 mg, 0.48 mmol) in ethylformate (7.7 mL, 48 mmol) was heated at reflux for 0.5 h. Concentration and column chromatography (hexane/AcOEt 1/1) gave 105 mg (93% yield) of 17 as a red oil. [α]25D +6.53 (c 1.00, CHCl3). The rotamers with 70:30 ratio were observed in 1H NMR. 1H NMR: Major Rotamer: 3.01 (1H, dd, J = 13.8, 6.7), 3.16 (1H, dd, J = 13.8, 5.2), 4.32 (1H, d, J = 15.6), 4.84 (1H, d, J = 15.6), 5.31 (1H, dd, J = 6.7, 5.2), 6.90 (1H, m), 7.04-7.32 (8H, m), 8.02 (1H, s); Minor Rotamer: 3.24 (1H, dd, J = 13.2, 2.8), 3.32 (1H, dd, J = 13.2, 7.0), 4.13 (1H, d, J = 13.4), 4.64 (1H, d, J = 13.4), 5.58 (1H, m), 7.04-7.32 (9H, m), 8.41 (1H, s). 13C NMR: 39.3 (CH2), 44.5 (CH2), 49.2 (CH2), 51.4 (CH2), 62.3 (CH), 64.3 (CH), 122.5 (CH), 123.0 (CH), 123.1 (CH), 123.5 (CH), 126.4 (CH), 127.0 (CH), 127.5 (CH), 127.7 (CH), 127.8 (CH), 127.9 (CH), 128.2 (CH), 128.6 (CH), 129.8 (CH), 130.0 (CH), 135.8 (C), 136.1 (C), 136.5 (C), 139.3 (C), 139.6 (C), 161.5 (CH), 161.7 (CH). IR: 1651. FABMS m/z: 238 [M+H]+. Anal. Calcd for C16H15NO: C, 80.98; H, 6.37; N, 5.90. Found: C, 80.90; H, 6.34; N, 5.95.
(S)-1-Benzyl-2-methylisoindoline (2b): Under argon atmosphere, to a solution of 17 (100 mg, 0.42 mmol) in THF (4.7 mL) was added BH3–THF solution (0.9 N in THF, 2.3 mL, 2.1 mmol) at room temperature over 2 min. The mixture was heated at reflux for 15 min and added water (4.7 mL), and then heated at reflux for 2.5 h. The whole was extracted with AcOEt (10 + 5+ 5 mL). The combined organic layers were washed with brine and dried over K2CO3. Concentration and column chromatography (hexane/AcOEt 1/1) gave 92 mg (98% yield) of (S)-2b13 as a colorless oil. [α]25D +75.5 (c 1.05, CHCl3). 1H NMR: 2.47 (3H, s), 3.04 (1H, dd, J = 14.1, 7.1), 3.12 (1H, dd, J = 14.1, 5.8), 3.67 (1H, d, J = 13.1), 4.01 (1H, dd, J = 7.1, 5.8), 4.28 (1H, d, J = 13.1), 6.88 (1H, d, J = 7.7), 7.09-7.33 (8H, m). 13C NMR: 41.2, 41.3, 60.9, 71.4, 122.1, 122.6, 126.2, 126.6, 126.9, 128.4, 129.6, 139.4, 139.7, 143.7. IR: 3026, 2925, 2771, 1601, 1454, 1354, 1151. FABMS m/z: 224 [M+H]+.
Asymmetric total synthesis of (S)-laudanosine.
(E)-2-(3,4-Dimethoxystyryl)-4,5-dimethoxybenzaldehyde (24): Under argon atmosphere, to a suspension of K3PO4 (50 g, 236 mmol), 22 (41.3 g, 169 mmol) and 23 (111 g, 674 mmol) in N,N-dimethylacetamide (339 mL) was added Pd(OAc)2 (3.79 g, 16.9 mmol). The mixture was heated at 140 °C for 0.5 h. Additional Pd(OAc)2 (1.26 g, 5.63 mmol) was added and the mixture was stirred for 0.5 h at the same temperature. The mixture was poured onto ice water, filtered through a Celite pad and extracted with CHCl3. The organic extracts were washed with water and brine, and dried over Na2SO4. Concentration and column chromatography (AcOEt/hexane 10/1, then AcOEt) followed by recrystallization from AcOEt/hexane gave 24 (49 g, 89%) as yellow needles of mp 158–159 °C. 1H NMR: 3.92, 3.96, 3.97, and 4.03 (each 3H, s), 6.89 (1H, d, J = 8.3), 6.91 (1H, d, J = 15.9), 7.09-7.11 (3H, m), 7.38 (1H, s), 7.75 (1H, d, J = 15.9), 10.33 (1H, s). 13C NMR: 55.86, 55.91, 56.0, and 56.1 (CH3 x 4), 108.7 (CH), 109.0 (CH), 111.1 (CH), 111.3 (CH), 119.2 (C), 120.3 (CH), 121.6 (CH), 126.4 (C), 130.0 (C), 133.2 (CH), 136.0 (C), 149.3 (C), 149.5 (C), 153.8 (C), 190.2 (CH). IR (nujor): 1663, 1597, 1512. EIMS m/z: 328 (M+). Anal. Calcd for C19H20O5: C, 69.50; H, 6.14. Found: C, 69.69; H, 6.08.
4-(4,5-Dimethoxy-2-((E)-2-nitrovinyl)styryl)-1,2-dimethoxybenzene (25)17: A solution of 24 (656 mg, 2 mmol) and NH4OAc (154 mg, 2 mmol) in dry nitromethane (3.8 mL) was stirred at 100 °C for 2 h and then concentrated. The residue was partitioned between AcOEt and water. The aqueous layer was extracted with AcOEt, and the combined organic extracts were washed with water and brine, and dried over Na2SO4. Concentration and column chromatography (AcOEt/hexane 2/1) followed by recrystallization from AcOEt/hexane gave 25 (712 mg, 96%) as red needles of mp 180–181 °C. 1H NMR: 3.93, 3.95, 3.97, and 4.00 (each 3H, s), 6.86 (1H, d, J = 15.9), 6.90 (1H, d, J = 8.3), 6.96 (1H, s), 7.07 (1H, d, J = 2.2), 7.08 (1H, s), 7.11 (1H, dd, J = 2.2, 8.3), 7.24 (1H, d, J = 15.9), 7.51 (1H, d, J = 13.2), 8.45 (1H, d, J = 13.2). 13C NMR: 55.90 (CH3), 55.91 (CH3), 56.0 (CH3 x 2), 109.1 (CH), 109.2 (CH), 109.4 (CH), 111.3 (CH), 120.2 (CH), 120.3 (C), 122.3 (CH), 129.8 (C), 133.1 (CH), 134.5 (C), 136.0 (CH), 136.7 (CH), 148.9 (C), 149.3 (C), 149.6 (C), 152.7 (C). IR: 3109, 1620, 1597, 1516. EIMS m/z: 371 (M+). Anal. Calcd for C20H21NO6: C, 64.68; H, 5.70; N, 3.77. Found: C, 64.47; H, 5.78; N, 3.60.
(E)-2-(2-(3,4-Dimethoxystyryl)-4,5-dimethoxyphenyl)ethanamine (26): To a suspension of LiAlH4 (5.28 g, 139 mmol) in dry THF (150 mL) was added a solution of 25 (12.9 g, 34.8 mmol) in dry Et2O (550 mL) under ice-water bath. After stirring at rt for 6 h, the excess of LiAlH4 was successively quenched with water (5.3 mL), 10% NaOH (5.3 mL) and water (15.9 mL). After filtration, the filtrate was dried over K2CO3. Concentration and column chromatography (AcOEt, then CHCl3/EtOH/25% aq. NH3 160/9/1) gave 26 (4.11 g, 86%) as a right brown oil. 1H NMR: 1.25 (2H, br s), 2.88 (2H, t, J = 6.7), 2.95 (2H, t, J = 6.7), 3.89, 3.90, 3.938, and 3.943 (each 3H, s), 6.70 (1H, s), 6.85 (1H, d, J = 15.9), 6.87 (1H, d, J = 8.3), 7.03-7.09 (2H, m), 7.11 (1H, s), 7.17 (1H, d, J = 15.9). 13C NMR: 37.0 (CH2), 43.4 (CH2), 55.84 (CH3), 55.87 (CH3), 55.88 (CH3), 55.92 (CH3), 108.6 (CH), 109.3 (CH), 111.3 (CH), 113.1 (CH), 119.2 (CH), 124.2 (CH), 128.3 (CH), 128.8 (C), 130.2 (C), 130.9 (C), 147.7 (C), 148.5 (C), 148.8 (C), 149.1 (C). IR: 3368, 3306, 1605, 1516. EIMS m/z: 343 (M+). Anal. Calcd for C20H25NO4: C, 69.95; H, 7.34; N, 4.08. Found: C, 69.80; H, 7.44; N, 4.02.
(E)-N-(2-(3,4-Dimethoxystyryl)-4,5-dimethoxyphenyl)formamide (27): A mixture of 26 (31 g, 90 mmol), HCO2H (100 mL, 2.7 mol) and Ac2O (86 mL, 0.9 mol) was stirred at rt for 2 h. The mixture was diluted with water, basified with 10% NaOH and extracted with CHCl3. The organic extracts were washed with brine and dried over Na2SO4. Concentration and column chromatography (AcOEt/CHCl3 1/1, then CHCl3/EtOH/25% aq. NH3 80/9/1) followed by recrystallization from AcOEt/hexane gave 27 (29.4 g, 88%) as colorless needles of mp 131.5–132.5 °C. 1H NMR: 2.98 and 3.52 (each 2H, t, J = 7.1), 3.90, 3.91, 3.95, and 3.99 (each 3H, s), 5.64 (1H, br s), 6.68 (1H, s), 6.87 (1H, d, J = 15.9), 6.88 (1H, d, J = 8.3), 7.09 (1H, dd, J = 1.6, 8.3), 7.14 (1H, s), 7.16 (1H, d, J = 1.6), 7.27 (1H, d, J = 15.9), 8.16 (1H, s). 13C NMR: 32.7 (CH2), 39.6 (CH2), 55.93 (CH3), 55.97 (CH3), 55.99 (CH3), 56.00 (CH3), 108.5 (CH), 109.0 (CH), 111.3 (CH), 113.0 (CH), 119.8 (CH), 123.6 (CH), 128.8 (CH), 128.9 (C), 129.2 (C), 130.7 (C), 148.1 (C), 148.7 (C), 149.0 (C), 149.3 (C), 161.3 (CH). IR: 3364, 1674, 1605, 1516. EIMS m/z: 371 (M+). Anal. Calcd for C21H25NO5: C, 67.91; H, 6.78; N, 3.77. Found: C, 67.69; H, 6.81; N, 3.73.
(E)-2-(2-(3,4-Dimethoxystyryl)-4,5-dimethoxyphenyl)-N-methylethanamine (28)18: To a 1.0 M THF solution of LiAlH4 (17.8 mL, 17.8 mmol) was added 27 (1.65 g, 4.46 mmol) in dry THF (80 mL) under ice-water bath. The mixture was stirred for 48 h at rt and then quenched with water (0.7 mL), 10% NaOH (0.7 mL) and water (2.1 mL) at 0 °C. After filtration, the filtrate was dried over K2CO3 and concentrated. Column chromatography (AcOEt/CHCl3 1/1, then CHCl3/ethanol/25% aq. NH3 80/9/1) followed by recrystallization from AcOEt/hexane gave 28 (1.47 g, 92%) as colorless needles of mp 108–109 °C. 1H NMR: 1.51 (1H, br s), 2.46 (3H, s), 2.82 (2H, t, J = 7.1), 2.93 (2H, t, J = 7.1), 3.90, 3.91, 3.94, and 3.95 (each 3H, s), 6.72 (1H, s), 6.86 (1H, d, J = 15.9), 6.87 (1H, d, J = 8.2), 7.05 (1H, d, J = 1.8), 7.08 (1H, dd, J = 1.8, 8.2), 7.11 (1H, s), 7.20 (1H, d, J = 15.9). 13C NMR: 33.3, 36.3, 53.0, 55.80, 55.82, 55.85, 108.5, 109.1, 111.3, 113.0, 119.3, 124.0, 128.2, 128.6, 130.3, 130.9, 147.6, 148.5, 148.7, 149.1. IR: 3317, 1605, 1516. EIMS m/z: 357 (M+).
(S)-(+)-Laudanosine (29)19: Under argon atmosphere, to a solution of 3a (147 mg, 0.5 mmol) and i-Pr2NH (0.04 mL) in toluene (5 mL) was added n-BuLi (1.6 N in hexane, 0.09 mL, 0.15 mmol) at –78 °C, and the solution was stirred for 10 min. A solution of 28 (179 mg, 0.5 mmol) in toluene (10 mL) was added over 2.5 h by a syringe pump at –30 °C. After addition of n-BuLi (1.6 N in hexane, 0.09 mL, 0.15 mmol), another solution of 28 (179 mg, 0.5 mmol) in toluene (10 mL) was added over 2.5 h. Additional n-BuLi (1.6 N in hexane, 0.09 mL, 0.15 mmol) was added and then the solution was stirred for 21 h at the same temperature.20 The reaction mixture was quenched with satd. aq. NH4Cl (5 mL) and basified with 10% aqueous K2CO3. The whole was extracted with AcOEt. The organic extracts were washed with brine and dried over K2CO3. Concentration and column chromatography (AcOEt/hexane 1/1, then AcOEt) gave 29 (344 mg, 96%) as a pale yellow solid of mp 88–90 °C and [α]25D +35.9 (c 1.05, CHCl3) and 3a (146 mg) quantitatively. 76% ee (HPLC, Daicel Chiralcel OD-H, hexane/i-PrOH 7/3, 1.0 mL/min, 254 nm, major 7.2 min and minor 17.9 min). Recrystallization of 29 (76% ee) from AcOEt/hexane afforded 29 (220 mg, 64%) of 99% ee as a white solid of mp 85–86 °C and [α]25D +57.3 (c 0.98, CHCl3).19 (Lit.19b: mp 87–88 °C and [α]29D +54.4 (c 0.20, CHCl3). 1H NMR: 2.54 (3H, s), 2.58 (1H, m), 2.73-2.86 (3H, m), 3.12-3.20 (2H, m), 3.57 (3H, s), 3.69 (1H, m), 3.79 (3H, s), 3.84 (3H, s), 3.85 (3H, s), 6.07 (1H, s), 6.56 (1H, s), 6.60 (1H, d, J = 1.8), 6.64 (1H, dd, J = 1.8, 8.0), 6.77 (1H, d, J = 8.0). 13C NMR: 25.5 (CH2), 40.8 (CH2), 42.6 (CH3), 46.9 (CH2), 55.5 (CH3), 55.68 (CH3), 55.73 (CH3), 55.8 (CH3), 64.8 (CH), 111.0 (CH), 111.1 (CH), 111.2 (CH), 113.0 (CH), 121.8 (CH), 126.1 (C), 129.3 (C), 132.5 (C), 146.3 (C), 147.26 (C), 147.31 (C), 148.6 (C). IR: 2932, 2839, 2793, 1605, 1512. EIMS m/z: 357 (M+).
ACKNOWLEDGEMENTS
This research was partially supported by Grant-in-Aid for Scientific Research (A) and Grant-in-Aid for Young Scientists (B) from JSPS.
References
1. T. E. Müller, K. C. Hultzsch, M. Yus, F. Foubelo, and M. Tada, Chem. Rev., 2008, 108, 3795. CrossRef
2. T. Ogata, A. Ujihara, S. Tsuchida, T. Shimizu, A. Kaneshige, and K. Tomioka, Tetrahedron Lett., 2007, 48, 6648. CrossRef
3. For chiral lithium amide-catalyzed intramolecular asymmetric hydroamination: (a) P. H. Martinez, K. C. Hultzsch, and F. Hampel, Chem. Commun., 2006, 2221; CrossRef (b) J. Deschamp, C. Olier, E. Schulz, R. Guillot, J. Hannedouche, and J. Collin, Adv. Synth. Catal., 2010, 352, 2171; CrossRef (c) J. Deschamp, J. Collin, J. Hannedouche, and E. Schulz, Eur. J. Org. Chem., 2011, 3329. CrossRef
4. Racemic intramolecular hydroamination for the synthesis of tetrahydroisoquinolines: W. A. L. Otterlo, R. Pathak, C. B. Koning, and M. A. Fernandes, Tetrahedron Lett., 2004, 45, 9561. CrossRef
5. (a) Q. Yao, E. P. Kinney, and Z. Yang, J. Org. Chem., 2003, 68, 7528; CrossRef (b) M. Dhar and A. Bhattacharjya, Indian. J. Chem., Sect. B, 2001, 40, 1140.
6. A. Poschalko, S. Welzig, M. Treu, S. Nerdinger, K. Mereiter, and U. Jordis, Tetrahedron, 2002, 58, 1513. CrossRef
7. F. D. Lewis, D. M. Bassani, E. L. Burch, B. E. Cohen, J. A. Engleman, G. D. Reddy, S. Schneider, W. Jaeger, P. Gedeck, and M. Gahr, J. Am. Chem. Soc., 1995, 117, 660. CrossRef
8. S. Tsuchida, A. Kaneshige, T. Ogata, H. Baba, Y. Yamamoto, and K. Tomioka, Org. Lett., 2008, 16, 3635. CrossRef
9. A. Briot, C. Baehr, R. Brouillard, A. Wagner, and C. Mioskowski, J. Org. Chem., 2004, 69, 1374. CrossRef
10. (a) J. Pietruszka and N. Schoene, Eur. J. Org. Chem., 2004, 5011; CrossRef (b) A. Joosten, E. Lambert, J. Vasse, and J. Szymoniak, Org. Lett., 2010, 12, 5128. CrossRef
11. (a) S. Hibino, E. Sugino, Y. Adachi, K. Nomi, K. Sato, and K. Fukumoto, Heterocycles, 1989, 28, 275; CrossRef (b) W.-Y. Qi, T.-S. Zhu, and M.-H. Xu, Org. Lett., 2011, 13, 3410; CrossRef (c) N. Philippe, F. Denivet, J.-L. Vasse, J. S. O. Santos, V. Levacher, and G. Dupas, Tetrahedron, 2003, 59, 8049; CrossRef (d) J. B. Edson, I. Keresztes, E. B. Lobkovsky, and G. W. Coates, ChemCatChem, 2009, 1, 122. CrossRef
12. In our previous report (ref. 8), aminolithiation–carbolithiation tandem double cyclization reaction of 1d was carried out by using 1.5 equiv of bulky t-BuTrNLi in THF at room temperature. However, no double cyclization product was observed in the reaction conditions described in this paper, i.e., catalytic amount of LDA and 3 in toluene at low temperature. This is because the carbolithiation would require higher temperature.
13. A. Ariffin, A. J. Blake, M. R. Ebden, W. S. Li, N. S. Simpkins, and D. N. A. Fox, J. Chem. Soc., Perkin Trans. 1, 1999, 2439. CrossRef
14. For an asymmetric synthesis of laudanosine: (a) M. Chrzanowska and M. D. Rozwadowska, Chem. Rev., 2004, 104, 3341; CrossRef (b) N. Blank and T. Opatz, J. Org. Chem., 2011, 76, 9777; CrossRef (c) A. L. Zein, L. N. Dawe, and P. E. Georghiou, J. Nat. Prod., 2010, 73, 1427; CrossRef (d) D. Mujahidin and S. Doye, Eur. J. Org. Chem., 2005, 2689; CrossRef (e) E. Anakabe, L. Carrillo, D. Badía, J. L. Vicario, and M. Villegas, Synthesis, 2004, 1093; CrossRef (f) R. Pedrosa, C. Andrés, and J. M. Iglesias, J. Org. Chem., 2001, 66, 243; CrossRef (g) D. L. Comins, P. M. Thakker, M. F. Baevsky, and M. M. Badawi, Tetrahedron, 1997, 53, 16327; CrossRef (h) Z. Czarnocki, J. B. Mieczkowski, and M. Ziólkowski, Tetrahedron: Asymmetry, 1996, 7, 2711; CrossRef (i) M. Kitamura, Y. Hsiao, T. Ohta, M. Tsukamoto, T. Ohta, H. Takaya, and R. Noyori, J. Org. Chem., 1994, 59, 297; CrossRef (j) D. L. Comins and M. M. Badawi, Tetrahedron Lett., 1991, 32, 2995; CrossRef (k) L. Gottlieb and A. I. Meyers, J. Org. Chem., 1990, 55, 5659; CrossRef (l) R. E. Gawley and G. A. Smith, Tetrahedron Lett., 1988, 29, 301; CrossRef (m) A. I. Meyers, D. A. Dickman, and M. Boes, Tetrahedron, 1987, 43, 5095; CrossRef (n) R. Noyori, M. Ohta, Y. Hsiao, M. Kitamura, T. Ohta, and H. Takaya, J. Am. Chem. Soc., 1986, 108, 7117; CrossRef (o) M. P. Cava and A. Afzali, J. Org. Chem., 1975, 40, 1553; CrossRef (p) S. Yamada, M. Konda, and T. Shioiri, Tetrahedron Lett., 1972, 22, 2215. CrossRef
15. M. R. Ebden and N. S. Simpkins, Tetrahedron, 1998, 54, 12923. CrossRef
16. J. Almena, F. Foubelo, and M. Yus, Tetrahedron, 1996, 52, 8545. CrossRef
17. A. Poschalko, S. Welzig, M. Treu, S. Nerdinger, K. Mereiter, and U. Jordis, Tetrahedron, 2002, 58, 1513. CrossRef
18. J. B. Bremner and L. V. Thuc, Aust. J. Chem., 1980, 33, 379. CrossRef
19. (a) G. M. Coppola, J. Heterocycl. Chem., 1991, 28, 1769; CrossRef (b) S. Kanokmedhakul, K. Kanokmedhakul, and R. Lekphrom, J. Nat. Prod., 2007, 70, 1536. CrossRef
20. Due to poor solubility in toluene, 28 was added portionwise to avoid its precipitation. Portionwise addition of n-BuLi was also required for complete consumption of 28, indicating that reactive anionic species were gradually protonated during the reaction. Chiral ligand 3c was used in slight excess with respect to n-BuLi, because reduced amount of chiral ligand often resulted in decreased enantioselectivity: H. Doi, T. Sakai, M. Iguchi, K. Yamada, and K. Tomioka, J. Am. Chem. Soc., 2003, 125, 2886. CrossRef