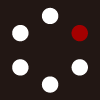
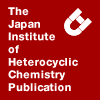
HETEROCYCLES
An International Journal for Reviews and Communications in Heterocyclic ChemistryWeb Edition ISSN: 1881-0942
Published online by The Japan Institute of Heterocyclic Chemistry
e-Journal
Full Text HTML
Received, 22nd June, 2012, Accepted, 2nd August, 2012, Published online, 3rd August, 2012.
DOI: 10.3987/COM-12-S(N)48
■ SYNTHESIS OF p-CHLOROAZACALIX[5]ARENE PENTAMETHYL ETHER: RING SIZE-DEPENDENT DEPROTECTION OF N-BENZYL GROUPS
Hirohito Tsue,* Kazuyuki Miyata, Daisuke Takahashi, Hiroki Takahashi, Kohei Sasaki, and Rui Tamura
Department of Interdisciplinary Environment, Graduate School of Human and Environmental Studies, Kyoto University, Kyoto 606-8501, Japan
Abstract
Syntheses of chlorinated azacalix[4]arene and azacalix[5]arene have been investigated. While the preparation of the former failed in the final N-debenzylation step, the latter was successfully obtained. The observed ring size-dependent deprotection was interpreted by steric effect.Azacalixarenes have recently emerged as a new family of calixarene, and their intriguing host-guest chemistry based on the introduction of nitrogen atoms as the bridging units are reported.1 Their complexation phenomena particularly in the solid state are of great interest because the bridging nitrogen atoms act as conjugation sites with the aromatic system to increase the electron density of the π-cloud, thereby boosting intermolecular interactions with guest species. Indeed, we thus far reported that purely organic crystals of azacalixarenes 1–42 rapidly and selectively captured CO2, a greenhouse gas of environmental importance. Among them, azacalix[5]arene 2 exhibits the selective sorption of CO2 even at ambient temperature and pressure conditions.2c,2d Besides, the smallest homologue 1 deposits single crystals showing highly selective CO2 uptake, and its CO2 sorption state is successfully analyzed at an atomic level by means of X-ray crystallography, demonstrating that CH/O interactions between the tert-butyl groups of 1 and a molecule of CO2 trapped in the crystal lattice play an important role in the observed highly selective CO2 uptake.2e
In this context, we anticipate that the replacement of tert-butyl groups attached at para positions of 1 and 2 with halogen atoms leads to the enhancement of intermolecular interactions with CO2 because of the formation of halogen bond.3 In conventional p-tert-butylcalixarenes with methylene bridges, halogenated derivatives are easily available via a post halogenation method involving retro Friedel-Crafts de-tert-butylation, followed by halogenation.4 In azacalix[4]arene 1, however, O-demethylation that is essential before carrying out retro Friedel-Crafts reaction was found to be challenging because of the low stability of the demethylated products to oxidation.5 In the present study, to establish synthetic pathway for halogenated derivatives 1’ and 2’, we have selected chlorine as a halogen atom and employed a convergent stepwise approach which is applied by us to the syntheses of tert-butyl derivatives 1 and 2.2d As an outcome, a larger derivative 2’ was successfully obtained, whereas the synthesis of 1’ failed because the final N-debenzylation step never proceeded in spite of our various attempts. Here we report the ring size-dependent N-debenzylation reaction observed in the syntheses of 1’ and 2’.
We first investigated the preparation of 1’ by modifying our previously reported procedure for the synthesis of tert-butyl derivative 1.2d As shown in Scheme 1, Buchwald-Hartwig aryl amination reaction of monomers 5 and 6 was carried out to prepare liner trimer 7, which was subsequently reacted with Boc-monoprotected monomer 8 to yield linear tetramer 9. The resulting product 9 was subjected to the additional Boc protection of the terminal amino group, N-benzylation of the bridging nitrogen atoms, and deprotection of Boc groups
to give linear tetramer 11. Tribenzylated azacalix[4]arene 126 was obtained in 43% yield by intramolecular cyclization of 11 using a Pd(0)-catalyzed Buchwald–Hartwig aryl amination reaction. Despite our numerous efforts, however, the final deprotection of N-benzyl groups of 12 was not accomplished, and this experimental result was in a sharp contrast to the same reaction for tert-butyl derivative 13 of which the N-benzyl groups were smoothly cleaved in quantitative yield by using 20% Pd(OH)2/C as a catalyst under a hydrogen atmosphere.2d All attempted hydrogenolysis reactions of chlorinated derivative 12 under essentially the same conditions using PtO2, 10% Pd/C, or 20% Pd(OH)2/C in the absence and presence of acetic acid as an additive resulted in the recovery of unreacted 12.7 This was also the case for the homogeneous N-debenzylation reaction of 12 with TMSI, though successful deprotection was achieved by this reagent in the synthesis of a larger homologue 2’ (vide infra).
To explore a reason for the observed unsuccessful N-debenzylation reaction of 12, DFT calculations at a B3LYP/6-31G(d,p) level were carried out for chlorinated derivative 12 and tert-butyl derivative 13. As shown in Figure 1, a large difference in electrostatic potentials was found for their bridging nitrogen atoms. Negative areas at the nitrogen atoms of 12 are reduced as compared to those of 13, suggesting that coordination to catalyst is less effective in 12 than 13. Besides, unlike conventional methylene-bridged calix[4]arenes,
molecular framework of azacalix[4]arenes is too rigid to permit ring inversion even in solution.8 Accordingly, it seems likely that, because of electronic and steric effects, both heterogeneous catalysts and TMSI uneasily approach the relevant reaction sites of 12 and thus lead to no debenzylation reaction.
Contrary to the unsuccessful synthesis of 1’, we succeeded in preparing a larger homologue 2’ by modifying our synthetic procedure established for tert-butyl derivative 2.2d As shown in Scheme 2, liner pentamer 17 was obtained by Buchwald-Hartwig aryl amination reaction of monomers 5 and 6, followed successively by N-benzylation of the bridging nitrogen atoms of 14, by Pd(0)-catalyzed aryl amination reaction of 15 with benzophenone imine, and finally by hydrolysis of the resultant ketimine 16 with HCl. Subsequent intramolecular ring-closing reaction of 17 was achieved by a Buchwald-Hartwig aryl amination reaction to give tetrabenzylated azacalix[5]arene 18 in 65% yield. As a final step, all N-benzyl groups of 18 were smoothly deprotected by TMSI to afford 2’9 in 76% yield. The successful synthesis of 2’ was unambiguously confirmed by X-ray crystallographic analysis10 (Figure 2), implying that the final deprotection reactions of N-benzyl groups of 12 and 18 were dependent on their ring size. In other words, it is very likely that azacalix[4]arene 12 blocks any reagents from approaching the reaction sites because of steric hindrance arising from its rigid framework,8,11 and more flexible azacalix[5]arene 18 allows enough space to promote N-debenzylation reaction to successfully produce 2’.
In conclusion, we have investigated the syntheses of 1’ and 2’ in which tert-butyl groups of 1 and 2 are replaced by chlorine atoms. A larger derivative 2’ was successfully synthesized, whereas the preparation of 1’ failed because the final N-debenzylation reaction never happened. It has been drawn from the present study that, judging from the successful synthesis of 2’, steric hindrance rather than
electronic effect plays a major role in the observed ring size-dependent deprotection of N-benzyl groups. Investigations on the gas sorption behaviors of 2’ are in progress in our laboratory to gain a further insight into the host–guest chemistry of this new calixarene family in the solid state.
ACKNOWLEDGEMENTS
This work was supported by a Grant-in-Aid for Scientific Research (C) (No. 23550052) from Japan Society for the Promotion of Science (JSPS).
References
1. H. Tsue, K. Ishibashi, and R. Tamura, in 'Heterocyclic Supramolecules I', ed. by K. Matsumoto, Springer-Verlag, Berlin Heidelberg, 2008, pp. 73–96; CrossRef (b) M.-X. Wang, Chem. Commun., 2008, 4541; CrossRef (c) H. Tsue, K. Ishibashi, and R. Tamura, J. Synth. Org. Chem. Jpn., 2009, 67, 898; CrossRef (d) M.-X. Wang, Acc. Chem. Res., 2012, 45, 182. CrossRef
2. (a) H. Tsue, K. Ishibashi, S. Tokita, H. Takahashi, K. Matsui, and R. Tamura, Chem. Eur. J., 2008, 14, 6125; CrossRef (b) H. Tsue, K. Matsui, K. Ishibashi, H. Takahashi, S. Tokita, K. Ono, and R. Tamura, J. Org. Chem., 2008, 73, 7748; CrossRef (c) H. Tsue, K. Ishibashi, S. Tokita, and K. Sakai, Jpn. Unexam. Pat. Appl. Publ., 2010-174002; (d) H. Tsue, K. Ono, S. Tokita, K. Ishibashi, K. Matsui, H. Takahashi, K. Miyata, D. Takahashi, and R. Tamura, Org. Lett., 2011, 13, 490; CrossRef (e) H. Tsue, H. Takahashi, K. Ishibashi, R. Inoue, S. Shimizu, D. Takahashi, and R. Tamura, CrystEngComm, 2012, 14, 1021. CrossRef
3. C. D. Gutsche, 'Calixarenes Revisited', ed. by J. F. Stoddart, Royal Society of Chemistry, Cambridge, 1998, pp. 104–107.
4. P. Metrangolo and G. Resnati, Chem. Eur. J., 2001, 7, 2511. CrossRef
5. K. Ishibashi, Thesis, Kyoto University, 2008.
6. 12: a light orange solid. mp 201–203 ℃; 1H NMR (CDCl3, 500 MHz) δ 7.28–7.18 (m, 15H, Ar-H), 6.74 (s, 4H, Ar-H), 6.58 (d, J = 2.5 Hz, 2H, Ar-H), 6.41 (d, J = 2.5 Hz, 2H, Ar-H), 5.11 (d, J = 16.5 Hz, 2H, ArCH2), 5.09 (d, J = 16.5 Hz, 1H, ArCH2), 4.88 (s, 1H, NH), 4.64 (d, J = 16.5 Hz, 2H, ArCH2), 4.59 (d, J = 16.5 Hz, 1H, ArCH2), 3.07 (s, 6H, OMe), 2.95 (s, 6H, OMe); 13C NMR (CDCl3, 125 MHz) δ 147.7, 144.8, 143.3, 142.9, 141.9, 138.4, 137.9, 128.8, 128.7, 127.6, 127.5, 127.4, 127.2, 115.9, 115.8, 113.3, 113.1, 69.6, 60.2, 59.6, 59.2, 58.7, 53.4, 31.9, 29.8, 29.4; IR (KBr) ν 3404 cm-1 (νN-H); HRMS (ESI) Calcd for C49H42Cl4N4O4Na [M+Na]+: m/z 913.1852. Found: m/z 913.1851; Anal. Calcd for C49H42Cl4N4O4: C, 65.93; H, 4.74; N, 6.28. Found: C, 65.71; H, 4.83; N, 6.28.
7. No cleavage of the C(sp2)-Cl bonds of 12 was observed after the heterogeneous hydrogenolysis reactions. For reference, no dechlorination of 12 proceeded even by using a catalytic system of [Cp*RhCl2]2 and 2-propanol (K. Fujita, M. Owaki, and R. Yamaguchi, Chem. Commun., 2002, 2964). CrossRef
8. (a) H. Tsue, K. Ishibashi, S. Tokita, K. Matsui, H. Takahashi, and R. Tamura, Chem. Lett., 2007, 36, 1374; CrossRef (b) K. Ishibashi, H. Tsue, H. Takahashi, and R. Tamura, Tetrahedron: Asymmetry, 2009, 20, 375. CrossRef
9. 2’: a light orange solid. mp >300 ℃; 1H-NMR (CDCl3, 400 MHz) δ 6.90 (s, 10H, Ar-H), 6.27 (br s, 5H, NH), 3.27 (s, 15H, OMe); 13C-NMR (CDCl3, 100 MHz) δ 141.2, 138.7, 129.1, 114.0, 59.8; HRMS (ESI) Calcd for C35H31Cl5N5O5 [M+H]+: m/z 776.0762. Found: m/z 776.0810; Anal. Calcd for C35H30Cl5N5O5·0.75CH2Cl2: C, 51.02; H, 3.77; N, 8.32. Found: C, 51.30; H, 3.88; N, 8.13.
10. Crystal data for 2’: monoclinic, space group C2/c, a = 17.89(2) Å, b = 16.24(1) Å, c = 24.09(2) Å, β = 92.41(1)°, V = 6991(10) Å3, Z = 8, Dc = 1.478 g cm-3, µ = 0.466 mm-1, T = 123(2) K, 7889 independent reflections, 475 refined parameters, R1 = 0.1199 (I > 2σ(I)), R1 = 0.1759 (all reflections), wR2 = 0.3006, S = 1.166. CCDC-886591 contains the supplementary crystallographic data for this paper. Free copy of the data can be obtained via http://www.ccdc.cam.ac.uk/conts/retrieving.html (or from the Cambridge Crystallographic Data Centre, 12 Union Road, Cambridge, CB2 1EZ, UK; Fax: +44 1223 336033; e-mail: deposit@ccdc.cam.ac.uk).
11. (a) K. Ishibashi, H. Tsue, N. Sakai, S. Tokita, K. Matsui, J. Yamauchi, and R. Tamura, Chem. Commun., 2008, 2812; CrossRef (b) K. Ishibashi, H. Tsue, H. Takahashi, S. Tokita, K. Matsui, and R. Tamura, Heterocycles, 2008, 76, 541. CrossRef
12. L. J. Farrugia, J. Appl. Crystallogr., 1997, 30, 565. CrossRef