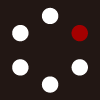
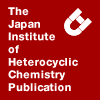
HETEROCYCLES
An International Journal for Reviews and Communications in Heterocyclic ChemistryWeb Edition ISSN: 1881-0942
Published online by The Japan Institute of Heterocyclic Chemistry
e-Journal
Full Text HTML
Received, 10th July, 2012, Accepted, 17th August, 2012, Published online, 24th August, 2012.
DOI: 10.3987/COM-12-S(N)95
■ Mn(OAc)3-Based α’-Oxidative Acetoxylation of N-Trifluoroacetyl Vinylogous Amides
Hong-Yu Lin and Barry B. Snider*
Department of Chemistry MS 015, Brandeis University, 415 South Street, Waltham, MA 02454-9110, U.S.A.
Abstract
Vinylogous amides 1 and 11 are oxidized by dried Mn(OAc)3 in benzene at 90 °C to pyridines 5 and 12, respectively, whereas the analogous N-trifluoroacetyl vinylogous amides 6 and 13 are oxidized by dried Mn(OAc)3 in benzene at 120 °C to give acetates 7 and 14. The α' oxo radicals that are generated in this oxidation cyclize to proximal double bonds to give tricylic alkenes such as 20.INTRODUCTION
The lycopodium alkaloid sauroine (7,8-dihydroxylycopodine, 4) was recently shown to improve memory retention in male Wistar rats making it a significant synthetic target (see Scheme 1).1 We chose to first address the synthesis of the simpler, related alkaloid 7-hydroxylycopodine (3) and recently reported a six step synthesis of 3 from vinylogous amide 1.2 For the synthesis of sauroine (4) we needed to hydroxylate the vinylogous amide 1 in the α'-position to obtain 2, which could then be elaborated to sauroine (4) by the route that was used to prepare 3 from 1.
Unfortunately, a variety of standard hydroxylation procedures failed to convert 1 to 2 indicating that the chemistry of vinylogous amides such as 1 is quite different from that of enones.2b For instance, Watt and Demir developed Mn(OAc)3-based α'-acetoxylations of enones that proceed in high yield with dried Mn(OAc)3 in benzene at reflux.3 They found that the use of both Mn(OAc)3•2H2O that had been dried in vacuum over P2O5 for several days and benzene at reflux as the solvent was crucial for the success of the α'-acetoxylation. Although only 2 equiv of dried Mn(OAc)3 are required by the stoichiometry, the reaction is heterogeneous and in practice requires 4-6 equiv of dried Mn(OAc)3. This procedure also works well on β-alkoxyenones (vinylogous esters),3a but we found that it converted vinylogous amide 1 to pyridine 5 in 65% yield, rather than the desired acetate ester of 2 (see Scheme 2).2b Clearly oxidation proceeds at the nitrogen rather than by enolization and formation of the α'-oxo radical. We thought that acylation of the nitrogen should both prevent this undesired oxidation and favor the formation of the required α'-oxo radical by increasing the acidity of the α'-proton.
RESULTS AND DISCUSSION
Electron deficient acyl groups should be the most effective at increasing the acidity of the α'-proton. We therefore treated 1 with trifluoroacetic anhydride and triethylamine in CH2Cl2 for 1 h at 0 °C to generate N-trifluoroacetyl vinylogous amide 6 in 95% yield.4 To our delight, we found that oxidation of 6 with dried Mn(OAc)3 in benzene in a sealed tube at 120 °C for 30 h cleanly afforded the desired acetate 7 as an inseparable ~1:1 mixture of isomers. This reaction is considerably slower than the α'-acetoxylations of β-alkoxyenones (vinylogous esters), which require 20-48 h in benzene at reflux. The rate determining step is formation of the Mn(III) enolate and the α'-protons of vinylogous imide 6 are probably less acidic than those of vinylogous esters. Hydrolysis of both the acetate and trifluoroacetamide of 7 with potassium carbonate in MeOH at 25 °C for 6 h gave 31% of the desired trans alcohol 2 and 28% of the undesired cis alcohol 8. The stereochemistry of the trans isomer 2 was established by the 11.2 Hz coupling between the diaxial methine hydrogens indicating that both the hydroxyl and methyl groups are equatorial.5 The coupling constant between the methine hydrogens in the cis isomer 8 is 5.2 Hz.5 The absence of a large coupling constant between the methine hydrogen adjacent to the methyl group and either adjacent methylene hydrogen indicates that in the preferred conformation of 8 the methyl group is axial and the alcohol is equatorial and probably hydrogen bonded to the adjacent carbonyl group.
Reaction of 3-bromopropylamine hydrobromide (9), dimedone (10), and 2,6-lutidine in EtOH in a sealed tube at 140 °C for 2.5 h gave the known vinylogous amide 116 in 61% yield (see Scheme 3). As expected from the conversion of 1 to 5, oxidation of the unprotected vinylogous amide 11 with dried Mn(OAc)3 in benzene at 90 °C for 20 min afforded pyridine 127 in 65% yield. Trifluoroacetylation of 11 afforded 13 (95%), which was oxidized with dried Mn(OAc)3 in benzene at 120 °C for 96 h to give the desired acetate 14 in 65% yield. The oxidation of 13 is considerably slower than that of 6, probably as a result of increased steric hindrance due to the second alkyl substituent. Demir recently reported that α'-acetoxylation of enones in 9:1 benzene/HOAc is much faster than in benzene.8 α'-Acetoxylation of 13 in 9:1 benzene/HOAc is complete in 6 h at 120 °C and 21 h at 90 °C, but is not quite as clean as the slower α'-acetoxylation in benzene at 120 °C.
In 1996 we reported that cyclization of the α'-keto radical formed by oxidation of enones with Mn(OAc)3 to a proximal double bond is faster than acetoxylation.9 We therefore investigated whether this was also the case with N-trifluoroacetyl vinylogous amides. Reaction of 3-bromopropylamine hydrobromide (9), cyclohexanedione 15,10 and 2,6-lutidine in EtOH in a sealed tube at 140 °C for 8 h gave vinylogous amide 16 in 52% unoptimized yield (see Scheme 4). Trifluoroacetylation afforded 17 (93%), which was oxidized with dried Mn(OAc)3 in benzene at 130 °C for 132 h to give the expected tricyclic alkene 20 in 61% yield and tertiary acetate 21 in 5% yield. Oxidation presumably generates α'-oxo radical 18, which undergoes the expected 5-exo cyclization with the alkene on the convex face to give cis fused tertiary radical 19, which is oxidized to the cation that loses a proton to yield 20 and reacts with acetate to form 21. The stereochemistry of the major product 20 was confirmed by the NOEs shown in Scheme 4 and by the coupling pattern of the allylic methine hydrogen (ddd, J = 11.2, 10.4, 7.2 Hz), which is consistent with that expected for this isomer, but not the other three diastereomers. The pyrrolidine-catalyzed intramolecular Michael reaction of ethyl (E)-5-(l-methyl-3-oxocyclohexyl)-2-pentenoate gave a single diastereomer corresponding to 20 providing further support for the structure assignment.11
In conclusion, we have shown that vinylogous amides 1 and 11 are oxidized by dried Mn(OAc)3 in benzene at 90 °C to pyridines 5 and 12, respectively, whereas the analogous N-trifluoroacetyl vinylogous amides 6 and 13 are oxidized by dried Mn(OAc)3 in benzene at 120 °C to give acetates 7 and 14. The α'-oxo radicals such as 18 that are generated in this oxidation cyclize to proximal double bonds to give tricyclic alkenes such as 20. We are currently exploring the elaboration of hydroxy vinylogous amide 2 to sauroine (4).
EXPERIMENTAL
General Experimental Methods. Reactions were conducted in flame- or oven-dried glassware under a nitrogen atmosphere and were stirred magnetically. The phrase "concentrated" refers to removal of solvents by means of a rotary-evaporator attached to a diaphragm pump (15-60 Torr) followed by removal of residual solvents at < 1 Torr with an vacuum pump. Flash chromatography was performed on silica gel 60 (230-400 mesh). Et3N, pyridine, MeCN and benzene were distilled from CaH2. Mn(OAc)3•2H2O was dried over P2O5 under vacuum for several days.3 1H and 13C NMR spectra were obtained on a 400 MHz spectrometer in CDCl3 with TMS as internal standard unless specifically indicated. Chemical shifts are reported in δ (ppm downfield from tetramethylsilane). Coupling constants are reported in Hz with multiplicities denoted as s (singlet), d (doublet), t (triplet), q (quartet), m (multiplet), and br (broad). IR spectra were acquired on an FT-IR spectrometer and are reported in wave numbers (cm-1). High resolution mass spectra were obtained using electrospray ionization (ESI).
2,3,4,6,7,8-Hexahydro-7-methyl-1-trifluoroacetyl-5(1H)-quinolinone (6). Trifluoroacetic anhydride (0.56 mL, 4.0 mmol, 2.0 equiv) and NEt3 (0.67 mL, 5.0 mmol, 2.5 equiv) were added to a solution of 1 (330 mg, 2.0 mmol) in 6 mL of CH2Cl2 at 0 °C. The resulting mixture was stirred at 0 °C for 1 h. The reaction was quenched by addition of water (12 mL). The mixture was extracted with CH2Cl2 (12 mL × 3). The combined organic layers were washed with water and brine, dried over Na2SO4, and concentrated. Flash chromatography of the residue on silica gel (6:1 hexanes/EtOAc) gave 496 mg (95%) of 6 as a colorless oil: 1H NMR 3.90 (br ddd, 1, J = 12.8, 3.6, 3.6, H-2), 3.43 (ddd, 1, J = 12.8, 10.4, 2.8, H-2), 2.75 (dddd, 1, J = 17.6, 10.4, 3.2, 3.2, H-8), 2.65 (br d, 1, J = 17.6, H-8), 2.54 (dd, 1, J = 12.8, 1.8, H-6), 2.43 (dddd, 1, J = 18.4, 6.8, 3.4, 3.4, H-4), 2.30 (ddddd, 1, J = 18.4, 8.4, 8.4, 2.4, 2.4, H-4), 2.24-2.13 (m, 1, H-7), 2.14 (dd, 1, J = 12.8, 12.8, H-6), 2.08-1.98 (m, 1, H-3), 1.90-1.78 (m, 1, H-3), 1.08 (d, 3, J = 6.4); 13C NMR 198.5, 155.8 (q, 1, J = 36.4, CF3CO), 153.2, 124.6, 115.9 (q, 1, J = 287, CF3), 45.4, 45.2 (q, 1, J = 4.0, CF3CONCH2), 37.3, 30.6, 22.2, 21.0, 20.1; IR 1702, 1663, 1623 (wk), 1202, 1140, 913, 748.
trans-2,3,4,6,7,8-Hexahydro-6-hydroxy-7-methyl-5(1H)-quinolinone (2) and cis-2,3,4,6,7,8-Hexahydro-6-hydroxy-7-methyl-5(1H)-quinolinone (8). A solution of 6 (496 mg, 1.9 mmol) in 20 mL of benzene and dried Mn(OAc)3 (1.98 g, 8.6 mmol, 4.5 equiv) were added to a resealable tube. The reaction mixture was sealed and heated at 120 °C for 30 h. The reaction mixture was cooled to room temperature and 10% aq. NaHSO3 solution (50 mL) was added. The mixture was extracted with EtOAc (50 mL × 3). The combined organic layers were washed with brine, dried over Na2SO4, and concentrated to give crude acetate 7 as a ~1:1 mixture of isomers.
To a solution of the residue in 12 mL of MeOH was added powdered K2CO3 (1.05 g, 4 equiv). The reaction mixture was stirred at room temperature for 6 h and concentrated. To the residue was added water (30 mL). The mixture was extracted with EtOAc (30 mL × 4). The combined organic layers were washed with brine, dried over Na2SO4, and concentrated. Flash chromatography of the residue on silica gel (100:1:1 EtOAc/MeOH/NEt3) gave 106 mg (31% from 6) of 2 as a white solid, followed by 96 mg (28% from 6) of 8 as a colorless oil.
Data for 2: mp 212-213 °C; 1H NMR 4.88 (br, 1, NH), 4.30 (br, 1, OH), 3.62 (d, 1, J = 11.2, H-6), 3.36-3.20 (m, 2, H-2), 2.45 (br ddd, 1, J = 16.0, 6.0, 6.0, H-4), 2.29 (br dd, 1, J = 13.6, 4.8, H-8), 2.24 (dd, 1, J = 13.6, 13.6, H-8), 2.20 (br dd, 1, J = 16.0, 4.8, H-4), 2.04-1.92 (m, 1, H-7), 1.90-1.70 (m, 2, H-3), 1.19 (d, 3, J = 6.8); 13C NMR 193.4, 158.9, 101.5, 76.1, 41.5, 36.8, 35.9, 20.6, 18.9, 18.4; IR 3423, 3264, 3108, 1602 (wk), 1570 (wk), 1513, 1264, 978, 749; HRMS (ESI) calcd for C10H16NO2 (MH+) 182.1181, found 182.1186.
Data for 8: 1H NMR 4.87 (br, 1, NH), 4.20 (br, 1, OH), 4.18 (d, 1, J = 5.2, H-6), 3.37-3.21 (m, 2, H-2), 2.78 (br dd, 1, J = 16.0, 5.2, H-8), 2.65-2.53 (m, 1, H-7), 2.50 (br ddd, 1, J = 16.0, 5.6, 5.6, H-4), 2.25 (br ddd, 1, 16.0, 6.8, 6.8, H-4), 2.09 (br d, 1, J = 16.0, H-8), 1.90-1.70 (m, 2, H-3), 0.92 (d, 3, J = 6.8); 13C NMR 192.1, 157.0, 101.1, 73.1, 41.5, 34.9, 33.3, 20.6, 18.6, 11.6; IR 3262 (br), 3089, 1569 (wk), 1514, 1343, 1260, 913, 748; HRMS (ESI) calcd for C10H16NO2 (MH+) 182.1181, found 182.1179.
2,3,4,6,7,8-Hexahydro-7,7-dimethyl-5(1H)-quinolinone (11). A solution of 5,5-dimethylcyclohexane-1,3-dione (10) (630 mg, 4.5 mmol), 3-bromopropylammonium bromide (9) (1.02 g, 4.7 mmol, 1.04 equiv), and 2,6-lutidine (1.6 mL, 13.5 mmol, 3.0 equiv) in 4 mL of EtOH was added to a resealable tube, which was sealed and heated at 140 °C for 2.5 h. The reaction mixture was cooled to room temperature and 1 M NaOH solution (30 mL) was added. The mixture was extracted with CH2Cl2 (30 mL × 3). The combined organic layers were washed with brine, dried over Na2SO4, and concentrated to about 5 mL. MeCN (30 mL × 2) was added and the resulting solution was concentrated again. Flash chromatography of the residue on silica gel (100:1:1 EtOAc/MeOH/NEt3) gave 491 mg (61%) of 11 as a white solid: mp 180-181 °C; 1H NMR 4.41 (br s, 1, NH), 3.26 (br t, 2, J = 6.4, H-2), 2.35 (br t, 2, J = 6.4, H-4), 2.20 (br s, 2, H-6 or 8), 2.12 (br s, 2, H-6 or 8), 1.80 (tt, J = 6.4, 6.4, H-3), 1.04 (s, 6); 13C NMR 193.7, 157.3, 103.4, 50.2, 43.1, 41.6, 32.4, 28.4 (2 C), 21.1, 18.7; IR 3265 (br), 1721, 1668, 1622, 1518 (wk), 1275, 1135, 913, 748. The 1H NMR spectral data are identical to those previously reported.6
7,8-Dihydro-7,7-dimethyl-5(6H)-quinolinone (12). A solution of 11 (50 mg, 0.28 mmol) in 4 mL of benzene and dried Mn(OAc)3 (292 mg, 1.26 mmol, 4.5 equiv) were added to a resealable tube. The resulting mixture was sealed and heated at 90 °C for 20 min. The reaction mixture was cooled to room temperature and 10% aq. NaHSO3 solution (15 mL) was added. The mixture was extracted with EtOAc (15 mL × 3). The combined organic layers were washed with brine, dried over Na2SO4, and concentrated. Flash chromatography of the residue on silica gel (100:1:1 EtOAc/MeOH/NEt3) gave 32 mg (65%) of 12 as a pale yellow oil: 1H NMR 8.71 (dd, 1, J = 4.8, 1.8), 8.27 (dd, 1, J = 8.0, 1.8), 7.30 (dd, 1, J = 8.0, 4.8), 3.05 (s, 2), 2.58 (s, 2), 1.12 (s, 6); 13C NMR 198.1, 162.2, 153.8, 134.5, 127.1, 122.1, 51.9, 46.3, 32.9, 28.2 (2 C); IR, 3070, 1686, 1582, 1300, 1282, 916, 821, 796, 742, 718. The 1H NMR, 13C NMR, and IR spectral data are identical to those previously reported.7
2,3,4,6,7,8-Hexahydro-7,7-dimethyl-1-trifluoroacetyl-5(1H)-quinolinone (13). Trifluoroacetic anhydride (0.56 mL, 4.0 mmol, 2.0 equiv) and NEt3 (0.67 mL, 5.0 mmol, 2.5 equiv) were added to a solution of 11 (360 mg, 2.0 mmol) in 6 mL of CH2Cl2 at 0 °C. The resulting mixture was stirred at 0 °C for 1 h. The reaction was quenched by addition of water (12 mL). The mixture was extracted with CH2Cl2 (12 mL × 3). The combined organic layers were washed with water and brine, dried over Na2SO4, and concentrated. Flash chromatography of the residue on silica gel (6:1 hexanes/EtOAc) gave 523 mg (95%) of 13 as a colorless oil: 1H NMR 3.66 (dd, 2, J = 5.6, 5.6, H-2), 2.70 (dd, 2, J = 2.0, 2.0, H-8), 2.38 (dddd, 2, J = 6.8, 6.8, 2.0, 2.0, H-4), 2.32 (s, 2, H-6), 1.99-1.91 (m, 2, H-3), 1.06 (s, 6); 13C NMR 198.5, 155.9 (q, 1, J = 36.8, CF3CO), 151.7, 124.2, 115.9 (q, 1, J = 287, CF3), 50.8, 45.2 (q, 1, J = 3.8, CF3CONCH2), 42.8, 33.7, 27.9 (2 C), 22.4, 20.0; IR 1702, 1664, 1627 (wk), 1199, 1143, 993, 913, 751.
6-Acetoxy-2,3,4,6,7,8-hexahydro-7,7-dimethyl-1-trifluoroacetyl-5(1H)-quinolinone (14). A solution of 13 (523 mg, 1.9 mmol) in 20 mL of benzene and dried Mn(OAc)3 (1.98 g, 8.6 mmol, 4.5 equiv) were added to a resealable tube. The reaction mixture was sealed and heated at 120 °C for 96 h. The reaction mixture was cooled to room temperature and 10% aq. NaHSO3 solution (50 mL) was added. The mixture was extracted with EtOAc (50 mL × 3). The combined organic layers were washed with brine, dried over Na2SO4, and concentrated. Flash chromatography of the residue on silica gel (6:1 hexanes/EtOAc) gave 26 mg (5%) of recovered 13, followed by 412 mg (65%) of 14 as a white solid: mp 104-105 °C; 1H NMR 5.27 (s, 1, H-6), 3.89 (ddd, 1, J = 12.8, 4.0, 4.0, H-2), 3.45 (ddd, 1, J = 12.8, 10.4, 2.4, H-2), 3.21 (ddd, 1, J = 18.0, 3.4, 3.4, H-8), 2.61 (dd, 1, J = 18.0, 1.8, H-8), 2.46 (ddddd, 1, J = 18.4, 8.0, 8.0, 2.4, 2.4, H-4), 2.32 (dddd, 1, J = 18.4, 6.8, 3.6, 3.6, H-4), 2.22 (s, 3), 2.09-1.99 (m, 1, H-3), 1.93-1.81 (m, 1, H-3), 1.13 (s, 3), 1.00 (s, 3); 13C NMR 192.6, 170.5, 156.0 (q, 1, J = 37.2, CF3CO), 150.7, 123.8, 115.8 (q, 1, J = 287, CF3), 80.1, 45.3 (q, 1, J = 3.8, CF3CONCH2), 42.7, 38.2, 27.2, 22.0, 20.6, 20.2, 19.4; IR 1748, 1705, 1681, 1628 (wk), 1204, 1152, 913, 749; HRMS (ESI) calcd for C15H19F3NO4 (MH+) 334.1266, found 334.1272.
2,3,4,6,7,8-Hexahydro-7-methyl-7-(4-methyl-3-penten-1-yl)-5(1H)-quinolinone (16). A solution of 5-methyl-5-(4-methyl-3-penten-1-yl)cyclohexane-1,3-dione (15)10 (400 mg, 1.9 mmol), 3-bromopropylammonium bromide (9) (440 mg, 2.0 mmol, 1.05 equiv), and 2,6-lutidine (0.66 mL, 5.7 mmol, 3.0 equiv) in 1.4 mL of EtOH was added to a resealable tube, which was sealed and heated at 140 °C for 8 h. The reaction mixture was cooled to room temperature and 1 M NaOH solution (15 mL) was added. The mixture was extracted with CH2Cl2 (15 mL × 3). The combined organic layers were washed with brine, dried over Na2SO4, and concentrated to about 5 mL. MeCN (15 mL × 2) was added and the resulting solution was concentrated again. Flash chromatography of the residue on silica gel (25:75:1 hexanes/EtOAc/NEt3) gave 247 mg (52%) of 16 as a pale yellow oil: 1H NMR 5.07 (br t, 1, J = 6.8), 4.72 (br, 1, NH), 3.27 (br t, 2, J = 6.0, H-2), 2.37-2.33 (m, 2, H-4), 2.26 (br d, 1, J = 16.0, H-6 or 8), 2.26 (br d, 1, J = 16.0, H-6 or 8), 2.18 (br d, 1, J = 16.0, H-6 or 8), 2.03 (br d, 1, J = 16.0, H-6 or 8), 1.98-1.91 (m, 2), 1.80 (tt, 2, J = 6.0, 6.0, H-3), 1.67 (br s, 3), 1.59 (br s, 3), 1.37-1.33 (m, 2), 1.03 (s, 3); 13C NMR 193.5, 157.2, 131.6, 124.3, 103.5, 48.5, 41.7, 41.6, 41.5, 35.0, 25.6, 24.8, 22.4, 21.1, 18.7, 17.5; IR 3261 (br), 3072, 1722, 1655, 1571 (wk), 1519, 913, 754; HRMS (ESI) calcd for C16H26NO (MH+) 248.2014, found 248.2019.
2,3,4,6,7,8-Hexahydro-7-methyl-7-(4-methyl-3-penten-1-yl)-1-trifluoroacetyl-5(1H)-quinolinone (17). Trifluoroacetic anhydride (0.17 mL, 1.2 mmol, 2.0 equiv) and NEt3 (0.20 mL, 1.5 mmol, 2.5 equiv) were added to a solution of 16 (147 mg, 0.60 mmol) in 3 mL of CH2Cl2 at 0 °C. The resulting mixture was stirred at 0 °C for 1 h. The reaction was quenched by addition of water (8 mL). The mixture was extracted with CH2Cl2 (8 mL × 3). The combined organic layers were washed with water and brine, dried over Na2SO4, and concentrated. Flash chromatography of the residue on silica gel (6:1 hexanes/EtOAc) gave 190 mg (93%) of 17 as a colorless oil: 1H NMR 5.05 (br t, 1, J = 6.8), 3.73-3.61 (m, 2, H-2), 2.81 (br d, 1, J = 17.6, H-8), 2.62 (br d, 1, J = 17.6, H-8), 2.41-2.34 (m, 2, H-4), 2.38 (d, 1, J = 16.4, H-6), 2.31 (d, 1, J = 16.4, H-6), 2.02-1.86 (m, 4, H-3 and CH2CH=C), 1.67 (br s, 3), 1.58 (br s, 3), 1.40-1.36 (m, 2), 1.04 (s, 3); 13C NMR 198.4, 155.9 (q, 1, J = 36.4, CF3CO), 151.5, 131.2, 124.5, 123.9, 115.9 (q, 1, J = 286, CF3), 49.3, 45.2 (q, 1, J = 3.8, CF3CONCH2), 41.1, 40.8, 36.4, 25.6, 24.7, 22.4, 22.3, 20.0, 17.5; IR 3010, 1738, 1704, 1666, 1628 (wk), 1204, 1143, 914, 752.
(±)-(5aR,6S,8aR)-2,3,4,5a,7,8,8a,9-Octahydro-8a-methyl-6-(1-methylethenyl)-1-trifluoroacetyl-6H-cyclopenta[g]quinolin-5(1H)-one (20) and (±)-(5aR,6S,8aR)-2,3,4,5a,7,8,8a,9-Octahydro-8a-methyl-6-(1-acetoxy-1-methylethyl)-1-trifluoroacetyl-6H-cyclopenta[g]quinolin-5(1H)-one (21). A solution of 17 (103 mg, 0.30 mmol) in 4 mL of benzene and dried Mn(OAc)3 (313 mg, 1.35 mmol, 4.5 equiv) were added to a resealable tube. The reaction mixture was sealed and heated at 130 °C for 132 h. The reaction mixture was cooled to room temperature and 10% aq. NaHSO3 solution (10 mL) was added. The mixture was extracted with EtOAc (10 mL × 3). The combined organic layers were washed with brine, dried over Na2SO4, and concentrated. Flash chromatography of the residue on silica gel (9:1 hexanes/EtOAc) gave 62 mg (61%) of 20 as a colorless oil, followed by 8 mg (8%) of recovered 17, and 6 mg (5%) of 21 as a white solid.
Data for 20: 1H NMR 4.79-4.73 (m, 2), 3.92 (br ddd, 1, J = 12.8, 4.0, 4.0, H-2), 3.39 (ddd, 1, J = 12.8, 10.4, 2.6, H-2), 3.16 (ddd, 1, J = 17.6, 3.0, 3.0, H-9), 2.91 (ddd, 1, J = 11.2, 10.4, 7.2, H-6), 2.40-2.32 (m, 2, H-4), 2.35 (d, 1, J = 11.2, H-5a), 2.28 (d, 1, J = 17.6, H-9), 2.15-1.98 (m, 2, H-3, H-7), 1.91-1.79 (m, 1, H-3), 1.77 (br s, 3), 1.74-1.64 (m, 2, H-7, H-8), 1.64-1.52 (m, 1, H-8), 1.14 (s, 3); 13C NMR 199.3, 156.0 (q, 1, J = 36.4, CF3CO), 150.4, 145.3, 123.0, 115.9 (q, 1, J = 287, CF3), 111.9, 59.0, 50.6, 45.5, 45.2 (q, 1, J = 3.8, CF3CONCH2), 39.1, 37.1, 29.3, 25.4, 22.5, 20.1, 18.9; IR 3070, 3007, 1737 (wk), 1703, 1660, 1628 (wk), 1203, 1145, 913, 750; HRMS (ESI) calcd for C18H23F3NO2 (MH+) 342.1681, found 342.1674. A 2D NOESY experiment showed strong NOEs between H-5a at δ 2.35 and both the alkenyl hydrogens at δ 4.79-4.73 and the upfield methyl group at δ 1.14 and a weak NOE between the alkenyl hydrogens and the upfield methyl group.
Data for 21: mp 91-92 °C; 1H NMR 3.80 (br ddd, 1, J = 13.2, 5.2, 3.0, H-2), 3.50 (ddd, 1, J = 13.2, 9.2, 2.8, H-2), 3.07 (ddd, 1, J = 18.0, 3.0, 3.0, H-9), 2.88 (ddd, 1, J = 10.4, 10.4, 7.2, H-6), 2.57-2.45 (m, 1, H-4), 2.41 (br d, 1, J = 18.0, H-9), 2.33 (d, 1, J = 10.4, H-5a), 2.25-2.15 (m, 1, H-4), 2.04-1.86 (m, 3, 2 H-3, H-7), 1.92 (s, 3), 1.72-1.56 (m, 2, H-7, H-8), 1.49 (s, 3), 1.47 (s, 3), 1.5-1.4 (m, 1, H-8), 1.13 (s, 3); 13C NMR 201.3, 170.5, 156.0 (q, 1, J = 37.2, CF3CO), 149.6, 123.1, 115.9 (q, 1, J = 287, CF3), 83.8, 57.4, 51.8, 45.9, 45.2 (q, 1, J = 3.4, CF3CONCH2), 38.7, 36.6, 26.0, 25.3, 24.9, 23.0, 22.5, 22.4, 20.5; IR 1731, 1708, 1666, 1632 (wk), 1205, 1160, 918, 749; HRMS (ESI) calcd for C20H27F3NO4 (MH+) 402.1892, found 402.1886.
References
1. M. G. Vallejo, M. G. Ortega, J. L. Cabrera, V. P. Carlini, S. Rubiales de Barioglio, R. S. Almiron, O. A. Ramírez, and A. M. Agnese, J. Nat. Prod., 2009, 72, 156. CrossRef
2. (a) H.-Y. Lin and B. B. Snider, Org. Lett., 2011, 13, 1234; CrossRef (b) H.-Y. Lin, R. Causey, G. E. Garcia, and B. B. Snider, J. Org. Chem., 2012, 77, 7143. CrossRef
3. (a) A. S. Demir, T. Sayrac, and D. S. Watt, Synthesis, 1990, 1119; CrossRef (b) C. Tanyeli, D. Özermirhan, and B. Sezen, Tetrahedron, 2002, 58, 9983; CrossRef (c) A. S. Demir, Z. Caliskan, and E. Sahin, J. Mol. Catal. B, 2007, 44, 87; CrossRef (d) A. S. Demir and M. Emrullahoglu, Curr. Org. Syn., 2007, 4, 223. CrossRef
4. For related N-acylations of vinylogous amides see: (a) Y. Tamura, Y. Kita, and J. Uraoka, Chem. Pharm. Bull., 1972, 20, 876; CrossRef (b) H. M. Sklenicka, R. P. Hsung, M. J. McLaughlin, L.-l. Wei, A. I. Gerasyuto, and W. B. Brennessel, J. Am. Chem. Soc., 2002, 124, 10435; CrossRef (c) G. Li, L. J. Carlson, I. K. Sagamanova, B. W. Slafer, R. P. Hsung, C. Gilardi, H. M. Sklenicka, and N. Sydorenko, Synthesis, 2009, 2905. CrossRef
5. For spectral data of related 6-hydroxy-5-methyl-2-cyclohexenones, see: (a) G. M. Rubottom and J. M. Gruber, J. Org. Chem., 1978, 43, 1599; CrossRef (b) A. K. Musser and P. L. Fuchs, J. Org. Chem., 1982, 47, 3121. CrossRef
6. (a) H. Reinshagen, Angew. Chem., Int. Ed. Engl., 1964, 3, 807; CrossRef (b) J. C. Rohloff, N. H. Dyson, J. O. Gardner, T. V. Alfredson, M. L. Sparacino, and R. Robinson III, J. Org. Chem., 1993, 58, 1935. CrossRef
7. F. Liéby-Muller, C. Allais, T. Constantieux, and J. Rodriguez, Chem. Commun., 2008, 4207. CrossRef
8. A. S. Demir, Ö. Reis, and A. C. Igdir, Tetrahedron, 2004, 60, 3427. CrossRef
9. (a) B. McCarthy-Cole, L. Han, and B. B. Snider, J. Org. Chem., 1996, 61, 7832; CrossRef (b) B. B. Snider and E. Y. Kiselgof, Tetrahedron, 1996, 52, 6073. CrossRef
10. G. Mehta and M. K. Bera, Tetrahedron Lett., 2008, 49, 1417. CrossRef
11. (a) B. Chenera, C.-P. Chuang, D. J. Hart, and C.-S. Lai, J. Org. Chem., 1992, 57, 2018; CrossRef See also: (b) A.-C. Guevel and D. J. Hart, J. Org. Chem., 1996, 61, 465; CrossRef (c) A.-C. Guevel and D. J. Hart, J. Org. Chem., 1996, 61, 473; CrossRef (d) Y.-L. Kuo, M. Dhanasekaran, and C.-K. Sha, J. Org. Chem., 2009, 74, 2033. CrossRef