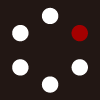
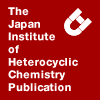
HETEROCYCLES
An International Journal for Reviews and Communications in Heterocyclic ChemistryWeb Edition ISSN: 1881-0942
Published online by The Japan Institute of Heterocyclic Chemistry
e-Journal
Full Text HTML
Received, 26th October, 2012, Accepted, 14th November, 2012, Published online, 28th November, 2012.
DOI: 10.3987/COM-12-12610
■ Preparation of 6-Ethynylpteridine Derivatives by Sonogashira Coupling
Winston Nxumalo* and Andrew Dinsmore
Department of Chemistry, University of Limpopo, Private Bag X 1106, Sovenga 0727, South Africa
Abstract
Sonogashira coupling of 6-sulfonyloxypteridine with various acetylenes is reported. Hydrolysis of the protecting groups with 1M NH3 gave the 6-subustituted 2,4-diaminopteridine while hydrolysis with 1M NaOH gave the 6-subsituted pterin.INTRODUCTION
Pterins are incorporated into a number of redox active cofactors which are essential to all forms of life, examples include tetrahydrobiopterin (BH4), the molybdenum cofactors (Moco), flavin and folic acid (Figure 1). Deficiency in these essential cofactors results in severe birth defects and in most cases death. The first total synthesis of pterins was reported in the 1940s and since then a number of natural occurring pterins and their derivatives have been synthesized.1,2 However, most of the synthesized pterins are folic acid derivatives and few synthetic routes in making non-folic acid derivatives exist.
The current methods of preparing non-folic acid derivatives include the oxidation of pterin with trifluoroacetic acid/hydrogen peroxide (TFA/H2O2) and treatment of the resultant 8-oxide with trifluoroacetic acid/acetyl chloride (TFA/AcCl) to give 6-chloropterin in an unusual Katada rearrangement (Scheme 1).3 The chloride is displaced by thiols via nucleophilic substitution reactions and acetylenes under Sonogashira coupling conditions, giving 6-substituted pterins. Other methods include the condensation of pyrimidone or pyrimidine ring with a substituted glyoxal, α-carbonylmethylene group and α-haloketones to give the corresponding 6-substituted pterin and pteridine rings, respectively (Scheme 2).4-6 A number of natural pterin compounds have been synthesised following these procedures, however, a number of problems such as selectivity of 6- vs 7-position and difficulty with certain chemical transformations, have been reported.
The challenge now lies in developing a synthetic method which is selective and versatile. Work reported by Joule et al.7 demonstrated the preparation of 6-tosyloxypteridine, selectively, in three steps starting from tetraaminopyrimidine and glyoxilic acid. The 6-tosyloxypteridine was subsequently shown to undergo Stille coupling reaction (Scheme 3).
In this paper we report Sonogashira coupling reactions on 6-benzenesulfonyloxypteridine and selective hydrolysis of the protecting groups to give exclusively the 6-substituted 2,4-diaminopteridines and 6-substituted pterins.
RESULTS AND DISCUSSION
Treating 2,4,5,6-tetraaminopyrimidine and glyoxylic acid in 2M H2SO4 gave 2,4-diaminopteridin-6-one 1 in 74% yield as described by Pfleiderer et al.8 The addition of Bredereck’s reagent9 afforded the more soluble 2,4-bis(N,N-dimethylaminomethyleneamino)pteridin-6-one7 2 in 91% yield which was treated with benzenesulfonyl chloride to give 2,4-bis(N,N-dimethylaminomethyleneamino)-6-benzenesulfonyloxypteridine 3 in 75% yield. With the protected 6-sulfonyloxypteridine 3 in hand, we were in a position to attempt the Sonogashira coupling reactions. Subjecting 3 and phenylacetylene under Sonogashira coupling conditions, after two hours, gave a mixture of two close running new products which were characterised to be the desired Sonogashira product, 2,4-bis(N,N-dimethylaminomethyleneamino)-6-(phenylethynyl)pteridine 4 along with the mono-deprotected product, 4-amino-2-(N,N-dimethylaminomethyleneamino)-6-(phenylethynyl)pteridine 5 in a ratio of ~1:1 (Scheme 4). Work by Joule et al.7 also reported the product mixture of desired Stille coupling product and mono-deprotected product. Further purification of the two close running products, by flash chromatography, gave a product distribution that favoured the mono-deprotected product 5 in ~1:9 ratio.
By monitoring the reaction with HPLC-UV-APCI-MS, we discovered that the desired product 4 was the only product when the reaction was quenched and the mono-deprotected 5 only formed after purification. We postulated that the deprotection step was facilitated by the solvent system employed (5-10% MeOH/DCM). Dissolving the 6-sulfonyloxypteridine 3 in DCM did not show any deprotection upon standing for 24 hours, however, by dissolving 3 in methanol we observed two peaks at RT 8.43 and 8.87 min corresponding to the mono-deprotected and fully-deprotected 6-sulfonyloxypteridine, respectively (Figure 2). It was therefore concluded that methanol is responsible for the mono-deprotection of the Sonogashira product 4. We were unable to develop a methanol-free solvent system to purify our products and the product mixture was carried into the next reaction without further purification.
Having shown that 6-sulfonyloxypteridine 3 can undergo Sonogashira coupling reactions, we investigated varying the acetylenes. Treating 3 with ethynyltrimethylsilane under the Sonogashira coupling conditions gave a mixture of two products which we assigned to be the desired coupling product 6 along with the mono-deprotected product 7 in ~1:1 ratio (Figure 3). Further purification gave a product distribution in favour of 7 in ~1:9 ratios.
When treating 6-sulfonyloxypteridine 3 with propargyl alcohol no desired product was observed, instead we obtained the mono-deprotected starting material. Having discovered that methanol is responsible for deprotection, we methylated the propargyl alcohol and proceeded with the Sonogashira coupling and obtained the desired product 8 along with the mono-deprotected product 9 in 9:1 ratio in favour of 8 (Scheme 5).
We intended that, under appropriate conditions, hydrolysis could be tuned to give initially the 2,4-diaminopteridine and under stronger hydrolytic conditions the corresponding pterin could be obtained. Treating 6-ethynylpteridine 5 with 1M NH3 solution, under gentle reflux, gave the desired 2,4-diamino-6-(phenylethynyl)pteridine10 10 in 77% yield. Similarly 6-ethynylpteridine 7, and 6-(methoxypropynyl)pteridine 8, gave the corresponding 2,4-diamino-6-ethynylpteridine 11 in 76% yield and 2,4-diamino-6-(3-methoxypropynyl)pteridine 12 in 74% yield, respectively (Scheme 6).10
Treating 6-(phenylethynyl)pteridine 5 with 1M NaOH/ethanol solution under gentle reflux afforded the desired 6-(phenylethynyl)pterin10 13 in 73% yield (Scheme 6). However, when using the same conditions on the TMS-ethynylpteridine 7, and the 6-(methoxypropynyl)pteridine 9, the corresponding pterins were not obtained.
We then turned our attention into monitoring the unsuccessful hydrolysis of TMS-pteridine 7, using HPLC-MS over 12 hours. The first step in the reaction involves the removal of both the protecting groups and the TMS group to give 2,4-diamino-6-ethynylpteridine 11, within the first hour. Formation of the desired 6-ethynylpterin 14 is observed after two hours, however substantial amounts of 11 is still present. Nucleophillic addition of an ethoxide group, presumably at the triple bond of 11 and 14, is observed after 3 hours with an addition of a second ethoxide group observed after 4 hours. The resulting pterin-ketals undergo an unusual C-C bond cleavage reaction to give 6-methylpterin 15 (Scheme 7). When substituting ethanol with methanol, similar nucleophillic additions of two methoxide groups were observed, as judged by HPLC-MS where a 15 amu difference was observed.
These results suggest that the ethynyl group is reactive towards nucleophiles, in our case ethoxide or methoxide, in a Michael addition-type reaction. Upon heating the acetal group cleaves off in a mechanism unknown to us but most likely involves breaking of the required C-C bond through anomeric assistance of an acetal group, as illustrated in Scheme 8. The leaving group is then the tautomer of the 6-methylpterin. We found little precedent for this mechanism. In a possible related report, a methoxide nucleophile add to a 6-ethynylpurine.11 The intermediate acetal, which was not isolated, then cleaves to give a 6-methylpurine.
In conclusion, we have demonstrated that a 6-sulfonyloxypteridine 3 is a useful substrate for Sonogashira coupling reactions using simple Pd catalyzed conditions.
EXPERIMENTAL
Melting points were obtained using a Stuart melting point apparatus, and are uncorrected. 1H and 13C spectra were reported on Bruker Avance-300 spectrometer at 300 MHz. Chemical shifts are reported in parts per million (ppm) relative to tetramethylsilane as internal standard (in the case of the 1H NMR at 7.27 ppm for CDCl3 and 2.45 ppm for DMSO, and in the case of 13C NMR at 77 ppm). Infrared spectra were obtained on a Bruker Tensor 27 spectrometer. Samples were placed on a diamond thin film. The absorptions are reported on the wave number (cm-1) scale, in the range 400 – 4000 cm-1. High resolution mass spectra were recorded on a Thermo-Finigan DFS instrument. Data are quoted : m/z value (relative abundance). Low resolution mass spectra were recorded on Thermo-Finigan LXQ instrument using either atmospheric pressure chemical ionization (APCI) or electro spray ionization (ESI) techniques. HPLC was run on a Thermo-Finigan instrument using a C18 (2) Phenomenex column with 150 x 4.60 mm dimensions. The samples were eluted with a water-methanol solvent system over 10 min using a gradient program: starting with 95% water for 2 min, changing to 100% methanol over the next 4 minutes and running for 4 min with 100% methanol. Detection of eluting components was achieved by a photodiode array and APCI-MS detector.
Synthesis of 2,4-diaminopteridin-6-one (1)8
In a 1L flask fitted with a stirrer bar, was added 2M H2SO4 (550 mL) and the flask warmed to 80 oC. To the flask was added 2,4,5,6-tetraaminopyrimidine (21.17 g, 0.136 mol) and stirring continued for 10 min. Glyoxylic acid (16.35 g, 0.272 mol, 2 eq.) was added, the solution stirred for a further 15 min then allowed to cool to rt and left to stir for 2 h. The resultant suspension was filtered, and the mustard solid washed with water (3 x 50 mL). The solid was suspended in water (150 mL) and neutralized with saturated aqueous NaHCO3 (cautiously added in 10 mL portions until effervescence stopped, ca. ~150 mL was required), the solid filtered and washed with water (5 x 60 mL) then MeOH (3 x 50 mL), air dried, and then dried under reduced pressure over P2O5 to give 2,4-diaminopteridin-6-one (17.9 g, 74%) as a mustard solid. mp (dec) >300 oC; m/z (ESI) 179 (M+H+, 100); λmax (1M NaOH) 410 and 265 nm. Physical and spectroscopic data agree with those reported elsewhere.8
Synthesis of 2,4-bis(N,N-dimethylaminomethyleneamino)pteridin-6-one (2)7
2,4-Diaminopteridin-6-one (4.02 g, 0.0225 mol) and Bredereck’s reagent9 (14 mL, 0.0675 mol, 3 eq.) were stirred together in DMF (50 mL), under an Ar atmosphere and warmed to 65 oC for 3 h, and allowed to cool to rt. The yellow precipitates were filtered, washed with little cold DMF then Et2O, air dried and finally dried under reduced pressure over P2O5 to give 2,4-bis(N,N-dimethylaminomethyleneamino)pteridin-6-one (6.20 g, 91%); mp 270 oC (dec); δH (300 MHz, CDCl3) 3.07 (3H, s), 3.08 (3H, s), 3.10 (3H, s), 3.15 (3H, s), 8.15 (1H, s), 8.48 (1H, s) and 8.76 ppm (1H, s); m/z (EI) 288 (M+, 100), 273 (45), 244 (19) and 232 (45). Spectroscopic data agree with those reported elsewhere.7
Synthesis of 2,4-bis(N,N-dimethylaminomethyleneamino)-6-benzenesulfonyloxypteridine (3)
2,4-Bis(N,N-dimethylaminomethyleneamino)pteridin-6-one (4.16 g, 14.5 mmol), DMAP (0.20 g, 1.45 mmol) and benzenesulfonyl chloride (3.8 mL, 29 mmol, 2 eq.) were dissolved in DCM (40 mL) and cooled to 0 oC. Et3N (3.7 mL, 29 mmol, 2 eq.) was added drop-wise into the mixture, and the final solution was allowed to warm to rt and stirred for 1 h. The reaction was quenched with saturated aqueous NaHCO3 (15 mL), the organic layer separated and the aqueous layer washed with DCM (2 x 20 mL). The combined organic layers were dried over MgSO4, and the solvent evaporated to dryness to give a dark yellow solid which was recrystallized from EtOH to give 2,4-bis(N,N-dimethylaminomethyleneamino)-6-benzenesulfonyloxypteridine (4.62 g, 75%) as a yellow solid; mp 203 – 205 oC (dec); δH (CDCl3, 300 MHz) 3.19 (3H, s), 3.22 (3H, s), 3.23 (3H, s), 3.30 (3H, s), 7.53 – 7.79 (3H, m), 8.25 – 8.28 (2H, dd, J = 7.8, 0.8 Hz), 8.56 (1H, s) and 8.95 – 8.97 ppm (2H, overlapping singlet); δC (CDCl3, 100 MHz) 35.4, 41.4, 125.2, 129.0, 129.4, 134.4, 136.3, 144.4, 148.6, 155.6, 158.3, 159.8, 166.5 and 168.5 ppm; m/z (EI) 428 (M+, 21%), 413 (40), 288.2 (100), 273.1 (45), 232 (40), 217 (80) and 162.9 (35) (HREI found: 428.13775. C18H20N8O332S requires 428.13791).
Synthesis of 4-amino-2-(N,N-dimethylaminomethyleneamino)-6-(phenylethynyl)pteridine (5)
In a 2-neck 50 mL round bottom flask, equipped with a magnetic stirrer bar and positive pressure of argon, 2,4-bis(N,N-dimethylaminomethyleneamino)-6-benzenesulfonyloxypteridine (1.00 g, 2.34 mmol), PdCl2(PPh3)2 (80 mg, 0.11 mmol), phenylacetylene (350 µL, 2.808 mmol) and Et3N (0.5 mL, 4.68 mmol, 2 eq.) were dissolved in DMF (15 mL), warmed to 80 oC and stirred for 2 h. The flask was cooled to rt, filtered through celite, concentrated in vacuo and purified on flash silica (eluting with 5% MeOH/DCM) to give two close running fractions (650 mg); m/z (APCI) 373 [M+H+, 60%], 318 [M-55, 70%]+; LCRT 7.01 and 7.05 min. Further purification of the close running fractions by flash chromatography on flash silica gave 4-amino-2-(N,N-dimethylaminomethyleneamino)-6-(phenylethynyl)pteridine (550 mg, 74%) as a yellow solid; mp 201 – 203 oC (dec); Rf 0.25; δH (DMSO-d6, 300 MHz) 3.01 (3H, s), 3.12 (3H, s), 7.46 – 7.51 (3H, m), 7.58 – 7.69 (2H, m), 8.03 (2H, broad s), 8.76 (1H, s) and 9.06 ppm (1H, s); m/z (EI) 317 (M+, 30%), 302 (50) and 262 (100) (HREI found: 317.13849. C17H15N7 requires 317.13889).
4-Amino-2-(N,N-dimethylaminomethyleneamino)-6-(trimethylsilylethynyl)pteridine (7)
In a 2-neck 50 mL round bottom flask, equipped with a magnetic stirrer bar and positive pressure of argon, 2,4-bis(N,N-dimethylaminomethyleneamino)-6-benzenesulfonyloxypteridine (1.0 g, 2.34 mmol), PdCl2(PPh3)2 (80 mg, 0.11 mmol), TMS-Cl (395 µL, 2.808 mmol) and Et3N (0.5 mL, 4.68 mmol, 2 eq.) were dissolved in DMF (15 mL), warmed to 80 oC and stirred for 2 h. The flask was cooled to rt, filtered through celite, concentrated in vacuo and purified on flash silica (eluting with 5% MeOH/DCM) to give two close running fractions (650 mg) as a dark yellow solid; m/z (APCI) 369 [M+H+, 45%], 314 [M-55]+; LCRT 7.34 and 7.45 min. Further purification by flash chromatography on flash silica gave 4-amino-2-(N,N-dimethylaminomethyleneamino)-6-(trimethylsilylethynyl)pteridine (460 mg, 63%) as a yellow solid; mp 253 oC (dec); Rf 0.29; δH (DMSO-d6, 300 MHz) 0.29 (9H, s), 3.23 (3H, s), 3.29 (3H, 1H), 8.88 (1H, s) and 9.03 ppm (1H, s); m/z (EI) 313 (M+, 90%), 298 (30), 269 (70), 258 (94), 243 (100) and 201 (40) (HREI Found: 313.14542. C14H19N728Si requires 313.14712).
Synthesis of 2,4-bis(N,N-dimethylaminomethyleneamino)-6-(3-methoxyprop-1-yn-1-yl)pteridine (8)
In a 2-neck 50 mL round bottom flask, equipped with a magnetic stirrer bar and positive pressure of argon, 2,4-bis(N,N-dimethylaminomethyleneamino)-6-benzenesulfonyloxypteridine (1.0 g, 2.34 mmol), PdCl2(PPh3)2 (80 mg, 0.11 mmol), propargyl alcohol (198 mg, 2.808 mmol) and Et3N (0.5 mL, 4.68 mmol, 2 eq.) were dissolved in 15 mL DMF, warmed to 80 oC and stirred for 2 h. The flask was cooled to rt, filtered through celite, concentrated and purified on flash silica (eluting with 5% MeOH/DCM) to give 2,4-bis(N,N-dimethylaminomethyleneamino)-6-(3-methoxyprop-1-yn-1-yl)pteridine (500 mg, 63%) as a yellow solid; mp 196 oC (dec); Rf 0.20; δH (CDCl3, 300 MHz) 3.19 – 3.22 (12H, overlapping s), 3.50 (3H, s), 4.40 (2H, s), 8.88 (1H, s) and 9.01 – 9.03 ppm (2H, overlapping s); δC (CDCl3, 100 MHz) 35.4, 35.8, 41,4, 41.6, 58.0, 60.2, 84.2, 88.8, 123.5, 128.8, 135.1, 153.6, 158.5, 162.5, 167.1 and 168.6 ppm; m/z (APCI) 341.2 [M + H+, 100%]; (HRESI found: 341.18460, C16H21N8O requires 341.1833).
Synthesis of 2,4-diamino-6-(phenylethynyl)pteridine (10)10
4-Amino-2-(N,N-dimethylaminomethyleneamino)-6-(phenylethynyl)pteridine (150 mg, 0.473 mmol) was treated with 10 mL EtOH/1M NH3 (1:1) mixture, gently refluxed for 16 h, filtered, the solid washed sequentially with water (5 mL), EtOH (5 mL) and Et2O (5 mL), and dried under Hi-vac. at 70 oC to give 2,4-diamino-6-(phenylethynyl)pteridine (95 mg, 77%) as a yellow solid; mp >300 oC (dec); δH (DMSO-d6, 300 MHz) 6.91 – 7.01 (2H, broad s), 7.46 – 7.48 (3H, m), 7.61 – 7.62 (2H, m), 7.84 – 7.87 (2H, broad s) and 8.86 ppm (1H, s); m/z (APCI) 263.1 [M + H+, 100] (HRESI found: 263.10340, C14H10N6 requires 263.10397). Spectroscopic data agree with those reported elsewhere.10
Synthesis of 2,4-diamino-6-ethynylpteridine (11)
4-Amino-2-(N,N-dimethylaminomethyleneamino)-6-(trimethylsilylethynyl)pteridine (200 mg, 0.64 mmol) was suspended in 25% NH3 solution (10 mL) and gently refluxed for 18 h, filtered through celite, concentrated to a small volume (ca.~3 mL), acidified with glacial AcOH (~1 mL) and stored at 2 oC for 24 h. The precipitates were collected by centrifugation, washed sequentially with water (5 mL), acetone (5 mL) and Et2O (5 mL) to give 2,4-diamino-6-ethynylpteridine (70 mg, 76%) as yellow solid; mp >300 oC (dec); vmax /cm-1 3410 (-NH), 3310 (-NH2), 3016, 2926; δH (DMSO-d6, 300 MHz) 7.09 – 7.11 (2H, broad s), 7.95 – 8.17 (2H, broad s) and 9.16 ppm (1H, s); m/z (APCI) 186 [M + H+, 100]. Attempts to get high resolution mass were unsuccessful in both HREI and HRESI instruments.
Synthesis of 2,4-diamino-6-(3-methoxyprop-1-yn-1-yl)pteridine (12)10
2,4-Bis(N,N-dimethylaminomethyleneamino)-6-(3-methoxyprop-1-yn-1-yl)pteridine (130 mg, 0.381 mmol) was dissolved in 8 mL EtOH/1M NH3 (1:1) mixture, gently refluxed for 8 h, allowed to cool to rt, acidified with AcOH and stored at 2 oC for 24 h. The precipitates were filtered, washed sequentially with water (5 mL), EtOH (5 mL) and Et2O (5 mL), and dried under High vac. at 60 oC to give 2,4-diamino-6-(3-methoxyprop-1-yn-1-yl)pteridine (65 mg, 74%) as a yellow solid; mp >300 oC (dec); δH (DMSO-d6, 300 MHz) 3.35 (3H, s), 4.40 (2H, s), 6.87 – 6.99 (2H, broad s), 7.83 (2H, broad s) and 8.74 ppm (1H, s); δC (DMSO-d6, 100 MHz) 57.2, 59.3, 83.4, 87.9, 122.5, 129.5, 152.6, 154.8, 163.4 and 172.3 ppm; m/z (APCI) 231 [M + H+, 100] (HRESI found: 231.0995, C10H10N6O requires 231.09888). Spectroscopic data agree with those reported elsewhere.10
Synthesis of 6-(phenylethynyl)pterin (13)10
4-Amino-2-(N,N-dimethylaminomethyleneamino)-6-(phenylethynyl)pteridine (200 mg, 0.631 mmol) was treated with 10 mL EtOH/1M NaOH (1:1) mixture, gently refluxed for 16 h, filtered through celite, concentrated to small volume (~3 mL), acidified with AcOH (~1.5 mL), and stored at 2 oC for 18 h. The yellow precipitates were collected by centrifugation, washed sequentially with water (5 mL), acetone (5 mL) and Et2O (5 mL), and dried under reduced pressure at 60 oC to give 6-(phenylethynyl)pterin (120 mg, 73%) as a yellow solid; mp >300 oC (dec); vmax /cm-1 1737 (C=O); δH (DMSO-d6, 300 MHz) 7.34 – 7.48 (3H, m), 7.63 (2H, m) and 8.72 ppm (1H, s); m/z (APCI) 264 [M + H+, 100] (HREI Found: 263.08092. C14H9N5O requires 263.08071). Spectroscopic data agree with those reported elsewhere.10
ACKNOWLEDGEMENTS
The authors gratefully acknowledge the National Research Foundation (NRF, South Africa), and University of Witwatersrand for financial support.
References
1. D. J. Brown, Heterocyclic compounds, Fused Pyrimidines, John Wiley and sons, 1988, pp. 1-4. CrossRef
2. R. Purrmann, Justus Liebigs Ann. Chem., 1940, 544, 182. CrossRef
3. E. C. Taylor and R. Kobylecki, J. Org. Chem., 1978, 43, 680. CrossRef
4. T. Hanaya, H. Baba, and H. Yamamoto, Carbohyd. Res., 2007, 342, 2159. CrossRef
5. S. Goswami and A. K. Adak, Tetrahedron Lett., 2002, 43, 8371. CrossRef
6. E. C. Taylor, K. L. Perlman, I. P. Sword, M. Sequin-Frey, and P. A. Jacobi, J. Am. Chem. Soc., 1973, 95, 6407. CrossRef
7. A. Dinsmore, D. C. Garner, and J. A. Joule, Tetrahedron, 1998, 54, 9559. CrossRef
8. G. Konrad and W. Pfleiderer, Chem. Ber., 1970, 103, 735. CrossRef
9. H. Bredereck, G. Simchen, H. Hoffman, P. Horn, and R. Wahl, Angew. Chem., 1967, 79, 311. CrossRef
10. E. C. Taylor and P. S. Ray, J. Org. Chem., 1987, 52, 3997. CrossRef
11. M. Kuchar, R. Pohl, I. Votruba, and M. Hocek, Eur. J. Org. Chem., 2006, 5083. CrossRef