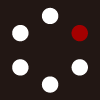
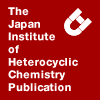
HETEROCYCLES
An International Journal for Reviews and Communications in Heterocyclic ChemistryWeb Edition ISSN: 1881-0942
Published online by The Japan Institute of Heterocyclic Chemistry
e-Journal
Full Text HTML
Received, 7th January, 2013, Accepted, 30th January, 2013, Published online, 12th February, 2013.
DOI: 10.3987/COM-13-12662
■ PREPARATION OF IMIDES vIA THE PALLADIUM-CATALYZED COUPLING REACTION OF ORGANOSTANNANES WITH METHYL N-[METHOXY(METHYLTHIO)METHYLENE]CARBAMATE
Kohei Orimoto, Takuhei Tomizawa, Yuki Namera, Harufumi Oyama, Takashi Niwa, and Masahisa Nakada*
Department of Chemistry and Biochemistry, School of Advanced Science and Engineering, Waseda University, 3-4-1 Okubo, Shinjuku-ku, Tokyo 169-8555, Japan
Abstract
The preparation of imides via the palladium-catalyzed coupling reaction of organostannanes is described. The palladium-catalyzed coupling reaction of aryl-, heteroaryl-, and alkenyl(tributyl)stannanes with methyl N-[methoxy(methylthio)methylene]carbamate in the presence of Cu(I) thiophene-2-carboxylate (CuTC) affords imino ethers, which are converted to the corresponding imides in high yield through acid hydrolysis.α,β-Unsaturated imides have been used in the catalytic asymmetric conjugate additions,1 and their transformation into various functional groups has been studied,1 suggesting their potential utility in organic synthesis. Typically, imides are prepared through the acylation of amides with carboxylic acid derivatives by bond connection at A2 or B3 in Scheme 1. These reactions require the corresponding carboxylic acids or their derivatives as starting materials, which limits their versatility. Alternatively, the reaction of nucleophiles with acylated isocyanates affords imides through bond connection at C (Scheme 1).4 However, this method is limited to reactions of electron-rich nucleophiles such as organometalic reagents; electron-deficient alkenes are generally inert toward the electrophiles.
The palladium-catalyzed C–C cross-coupling reactions of organoboranes5a,b or organostannanes5c with thioesters, known as the Liebeskind–Srogl coupling reaction, are highly attractive and beneficial for the synthesis of complex natural products because the coupling reactions afford ketones under neutral conditions. This C–C bond-forming reaction has been extended to cross-coupling reactions between a variety of organosulfur and organometallic reagents.5d Among them, cross-coupling reactions using organostannanes are useful for the synthesis of complex natural products because most organostannanes and organosulfur compounds are stable to air and moisture, and can be purified by silica gel chromatography. Moreover, a variety of organostannanes can be prepared through various reactions.6
A one-carbon elongation reaction is a useful and important transformation in organic synthesis. As palladium-catalyzed one-carbon elongation reactions utilizing organostannanes, reactions of organic halides with carbon monoxide and tin hydride,7 and reactions of organostannanes with carboxylic acid chlorides,8 chloroformates, and carbamoyl chlorides9 have been reported. However, to the best of our knowledge, the palladium-catalyzed one-carbon elongation reaction of organostannane to afford the imide product has never reported.
Recently, we found that the palladium-catalyzed coupling reaction of phenylboronic acid (1) with methyl N-[methoxy(methylthio)-methylene]carbamate (2) affords the corresponding imino ether 3a,10 and subsequent acid hydrolysis gives the imide 4a11 in 88% overall yield (Scheme 2).12 This palladium-catalyzed reaction also work with alkenyl- and alkylborons indicating its wide applicability.12
We also reported the preparation of imide 6a by the palladium-catalyzed coupling reaction of alkenyl(tributyl)stannane 5a with 2 and subsequent conversion of the resultant imino ether (Scheme 3).13
Some reactive imides were prepared in high yields through this method.13 Therefore, to verify the scope and limitations of the method, we studied the preparation of imides via the palladium-catalyzed coupling reactions of aryl(tributyl)stannanes and alkenyl(tributyl)stannanes with 2; the results are reported herein.
We first examined the palladium-catalyzed coupling reaction of tributyl(phenyl)stannane 7a with the imide 8a under the conditions reported by Liebeskind and Srogl (Scheme 4).5c,d However, no products were obtained, and only the consumption of 8a was observed. This result suggested that 8a decomposed under the reaction conditions, as observed previously in the reaction between 1 and 2a.12 The reaction of 7a with 8a-1, which has a methoxymethyl (MOM) group on the nitrogen atom, gave the same result.
Consequently, we examined the palladium-catalyzed coupling reaction of 7a with 2, which was successfully used in the reaction with 1 (Table 1). Usually, palladium-catalyzed coupling reactions of alkylthio esters with organostannanes require copper(I) salt as an additive,5c,d and likewise, the reaction of 7a with 2 did not proceed in the absence of copper(I) salt (entry 1). The reaction of 7a using a catalytic amount of Pd(PPh3)4 in the presence of CuI afforded 3a (30%, entry 2), and the reaction using CuBr·SMe2 improved the yield to 50% (entry 3).
The use of Cu(I) thiophene-2-carboxylate (CuTC) in THF gave a better yield (81%, entry 4). The use of Pd2(dba)3 and TFP as a ligand did not improve the yield (49%, entry 5), but the reaction using Pd2(dba)3 and Ph3As proceeded faster, and the yield was improved to 91% (entry 6). The reaction with a reduced amount of CuTC (1.5 equiv) was slow and the yield was reduced to 62% (entry 7).
The preparation of imides 4b-f through the palladium-catalyzed coupling reactions of other aryl(tributyl)stannanes 7b-f with 2 and the subsequent hydrolysis of imino ethers 3b-f were examined (Table 2). The coupling reaction of tributyl(p-tolyl)stannane 7b with 2 afforded 3b in 99% yield, and its hydrolysis using 1 N HCl in THF at room temperature gave 4b14 in 95% yield (entry 2). The reactions of tributyl(p-methoxyphenyl)stannane 7c and tributyl(p-nitrophenyl)stannane 7d with 2 also gave the corresponding imino ethers, which were subjected to acid hydrolysis to give 4c and 4d, respectively, in high yields (90%, 2 steps, entries 3 and 4).
The reaction of heteroarylstannanes was also examined. The reaction of tributyl(2-thienyl)stannane 7e with 2 was completed within 1 h to afford 3e quantitatively, and subsequent acid hydrolysis gave 4e in 95% yield (entry 5). The palladium-catalyzed coupling reaction of 7e with 2 was superior to the reaction of 2-thienylboronic acid with 2 (cf. 12 h, 60% (3e)).12
The reaction of 2-furanboronic acid 7f with 2 and subsequent acid hydrolysis also proceeded smoothly to afford imide 4f in 91% overall yield (entry 6). As summarized in Table 2, the palladium-catalyzed coupling reactions of aryl(tributyl)stannanes 7a-f with 2 were completed within four hours.
The palladium-catalyzed coupling reactions of alkenyl(tributyl)stannanes 5a-j were examined next (Table 3). We reported the palladium-catalyzed coupling reactions of 5a and 5b with 2, but 20 mol % of palladium reagent was used for the reaction.13 Therefore, the reactions of 5a and 5b with 2 were carried out under the optimized reaction conditions, using 10 mol % of palladium reagent. Transformation of the imino ethers to 6a-j was achieved using TMSCl and NaI in acetonitrile at room temperature, which are the optimized reaction conditions that we found.
As a result, the yield of 6a was reduced from 93% to 87% (2 steps) (entry 1), and that of 6b was improved from 59% to 69% (2 steps) (entry 2), but no starting material remained in either reaction. Hence, further optimizations could improve the yields, and these results indicate 10 mol % of palladium reagent is sufficient for the catalytic reaction.
Next, the palladium-catalyzed coupling reaction of 5c was performed. Compound 5c was prepared through the palladium-catalyzed coupling reaction of the known 5c-115 (Scheme 5) and hexabutyldistannane.6e,g Compound 5c contains dienyl ether and lactone in the molecule, and is therefore sensitive to the reaction conditions, but subsequent treatment with TMSCl and NaI afforded 6c in 66% yield (2 steps) (entry 3).
Compounds 5d was prepared starting from known 5d-116 (Scheme 5), which was converted to 5d-2 by carbometallation and subsequent acid-catalyzed lactonization. Compound 5d-2 was subjected to the halogen-metal exchange reaction using isopropylmagnesium bromide,17 and subsequent reaction with tributyltin chloride afforded 5d.
Compound 5e was prepared from the corresponding alkenyl iodide18 according to the procedure for the preparation of 5c. Compounds 5d (entry 4) and 5e (entry 5) are tetrasubstituted alkenylstannanes, and hence, these compounds were surmised to be affected by steric interactions during the coupling reactions. However, the reactions proceeded smoothly and were completed within 90 min, and subsequent treatment with TMSCl and NaI afforded 6d and 6e, respectively, in high yields.
Compounds 5f19 (entry 6) and 5g20 (entry 7) are known acyclic trisubstituted alkenylstannanes, and isomerization of the double bond was suspected to occur during the palladium-catalyzed coupling reaction and subsequent transformation. However, no isomerization was observed during the two-step sequence, and the corresponding imides 6f and 6g, respectively, were afforded in good to high yields.
Compound 5h (entry 8) was prepared through the reaction of the corresponding alcohol21 with chloromethyl methyl ether, which has no conjugated carbonyl group. The coupling reaction of 5h proceeded smoothly, and subsequent acid hydrolysis of the imino ether with pyridinium p-toluenesulfonate (PPTS) and H2O in THF afforded 6h in 69% yield (2 steps). Mild acid hydrolysis conditions were required in this case owing to the acid-sensitive MOM ether in the molecule.
The coupling reaction of compound 5i22 (entry 9) also proceeded without problem to afford the corresponding imide in 93% yield. However, as is well known, the allylic TBS ether was labile even under these mild acidic conditions (PPTS, THF, and H2O). Therefore, yield of 6i was 53% (2 steps), but the reaction of compound 5j23 (entry 10) bearing an acid-resistant benzyl group provided 6j1p in a higher yield. No isomerization of the alkene was observed in the reactions of 5h, 5i, and 5j.
In summary, we have developed a new imide-preparation method via the palladium-catalyzed coupling reaction of organostannanes with methyl N-[methoxy(methylthio)methylene]carbamate. This method involves a one-carbon elongation that does not require the use of toxic carbon monoxide. The palladium-catalyzed coupling reaction is broadly applicable to aryl-, heteroaryl-, and alkenylstannanes, affording a variety of imino ethers, which are easily converted to the corresponding imides in high to excellent yields under mild acidic conditions. Organostannanes can be prepared by a variety of reactions, as described above, and furthermore, most organostannanes are usually stable in air and moisture, and can be purified by silica gel chromatography. Therefore, the developed method could be useful for the preparation of a variety of imides.
EXPERIMENTAL
1H and 13C NMR spectra were recorded on a JEOL AL-400 spectrometer. 1H and 13C chemical shifts are reported in ppm downfield from tetramethylsilane (TMS, δ scale) with the solvent resonances as internal standards. The following abbreviations were used to explain the multiplicities: s, singlet; d, doublet; t, triplet; q, quartet; m, multiplet; band, several overlapping signals; br, broad. IR spectra were recorded on a JASCO FT/IR-8300. Optical rotations were measured using a 2 mL cell with a 1 dm path length on a JASCO DIP-1000. Mass spectra and elemental analyses were provided at the Materials Characterization Central Laboratory, Waseda University. All reactions were carried out under an argon atmosphere with dry, freshly distilled solvents under anhydrous conditions, unless otherwise noted. Melting point (mp) is uncorrected and recorded on a Yamato capillary melting point apparatus. All reactions were monitored by thin-layer chromatography carried out on 0.25 mm E. Merck silica gel plates (60F-254) using UV light as visualizing agent and phosphomolybdic acid and heat as developing agents. Kanto Chemical Silica Gel 60N (spherical, neutral, 63-210 μm or 40-50 μm partial size) was used for flash chromatography. Preparative thin-layer chromatography (PTLC) separations were carried out on self-made 0.3 mm E. Merck silica gel plates (60F-254). In general, reactions were carried out in dry solvents under an argon atmosphere. Unless otherwise noted, materials obtained from commercial suppliers were used without further purification. Acetonitrile was distilled from calcium hydride before use. Tetrahydrofuran (THF) were distilled from sodium/benzophenone ketyl. Toluene was distilled from sodium.
Preparation of methyl N-[methoxy(methylthio)methylene]carbamate (2).13 To a flame-dried 300 mL round-bottomed flask equipped with stir bar were added KSCN (2.77 g, 28.5 mmol, 1.1 equiv), THF (120 mL), and then methyl chloroformate (2.45 g, 25.9 mmol) at 0 °C. The reaction mixture was stirred at room temperature for 4 h, and then MeOH (1.78 mL, 44.0 mmol, 1.7 equiv) was added to the reaction mixture at room temperature. After 4 h, to the reaction mixture were added dimethyl sulfate (2.694 mL, 28.5 mmol, 1.1 equiv) and K2CO3 (7.15 g, 51.8 mmol, 2.0 equiv) at room temperature. The reaction mixture was stirred at room temperature for 12 h. After the starting material disappeared, the mixture was filtered through a plug of Celite, and the filtrate was concentrated under reduced pressure. The residue was purified by recrystallization to afford 2 (1.99 g, 47%): Rf = 0.50 (hexane/EtOAc = 2/1); mp 62 °C; 1H NMR (400 MHz,CDCl3) δ 3.96 (s, 3H), 3.76 (s, 3H), 2.37 (s, 3H); 13C NMR (100 MHz,CDCl3) δ 174.8 (C), 161.2 (C), 57.6 (CH3), 53.2 (CH3), 13.8 (CH3); IR (neat) νmax 3038, 2958, 1672, 1533, 1437, 1279, 1182, 1054 cm–1; HRMS (ESI) [M+Na]+ calcd for C5H9NO3NaS: 186.02008, found: 186.02088.
General procedure for the preparation of imides.
Methyl methoxy(phenyl)methylenecarbamate (3a). To a stirred solution of 1a (39.8 mg, 0.206 mmol) in THF (2 mL) was added Pd2(dba)3 (9.4 mg, 0.010 mmol, 0.05 equiv), Ph3As (18.9 mg, 0.062 mmol, 0.3 equiv), CuTC (0.118 g, 0.619 mmol, 3.0 equiv) and methyl N-[methoxy(methylthio)methylene]carbamate (67.2 mg, 0.412 mmol, 2.0 equiv) at room temperature, and the resultant solution was stirred at 60 oC. After the starting material disappeared, the mixture was filtered through a Celite pad, and the filtrate was concentrated under reduced pressure. The residue was purified by flash chromatography (hexane/EtOAc = 20/1) to afford 3a10 (33.7 mg, 91%): 1H NMR (400 MHz, CDCl3) δ 7.64-7.59 (m, 2H), 7.53-7.38 (m, 3H), 3.93 (s, 3H), 3.73 (s, 3H).
Methyl benzoylcarbamate (4a). To a stirred solution of 3a (33.7 mg, 0.514 mmol) in THF (2 mL) was added 1 N-HCl (3 drops) at room temperature. After the reaction was completed, H2O (2 mL) was added to the reaction mixture, and the aqueous layer was extracted with CH2Cl2 (5 mL×2). The combined organic layers were washed with brine (2 mL×1), dried (Na2SO4), filtered, and concentrated under reduced pressure. The residue was purified by flash chromatography (hexane/EtOAc = 10/1) to afford 4a11 (30.5 mg, 97%): 1H NMR (400 MHz, CDCl3) δ 8.03 (s, 1H), 7.81 (d, J = 7.3 Hz, 2H), 7.60 (t, J = 7.3 Hz, 1H), 7.81 (t, J = 7.3 Hz, 2H) 3.87 (s, 3H).
Methyl methoxy(p-tolyl)methylenecarbamate (3b): Rf = 0.50 (hexane/EtOAc = 2/1); 1H NMR (400 MHz, CDCl3) δ 7.46 (d, J = 8.3 Hz, 2H), 7.15 (d, J = 8.3 Hz, 2H), 3.86 (s, 3H), 3.68 (s, 3H), 2.33 (s, 3H); 13C NMR (100 MHz, CDCl3) δ 163.2 (C), 161.5 (C), 142.3 (C), 129.2 (CH), 128.0 (CH), 127.8 (C), 54.9 (CH3), 53.3 (CH3), 21.4 (CH3); IR (neat) νmax 3024, 2952, 1713, 1662, 1264, 1179, 1108 cm–1; HRMS (ESI) [M+Na]+ calcd for C11H13NO3Na: 230.0800, found: 230.0793.
Methyl 4-methylbenzoylcarbamate (4b):14 1H NMR (400 MHz, CDCl3) δ 8.03 (s, 1H), 7.71 (d, J = 7.6 Hz, 2H), 7.60 (d, J = 7.6 Hz, 2H), 3.86 (s, 3H), 2.42 (s, 3H).
Methyl 4-methoxybenzoylcarbamate (4c): 1H NMR (400 MHz, CDCl3) δ 8.25 (bs, 1H), 7.83 (d, J = 8.8 Hz, 2H), 6.95 (d, J = 8.8 Hz, 2H), 3.87 (s, 3H), 3.84 (s, 3H); 13C NMR (100 MHz, CDCl3) δ 164.1, 163.4, 151.9, 129.8, 125.0, 114.0, 55.5, 53.1; IR(neat) νmax 3279, 2955, 2842, 1753, 1603, 1490, 1169, 776 cm–1; HRMS (ESI) [M+Na]+ calcd for C10H11NO4Na: 232.05858, found: 232.05837.
Methyl 4-nitrobenzoylcarbamate (4d): 1H NMR (400 MHz, CDCl3) δ 8.34 (d, J = 8.3 Hz, 2H), 8.05 (bs, 1H), 7.96 (d, J = 8.3 Hz, 2H), 3.89 (s, 3H); 13C NMR (100 MHz, CDCl3) δ 167.6, 166.9, 128.9, 125.3, 124.0, 116.4, 53.5; IR(neat) νmax 3383, 3117, 3074, 2959, 2922, 2853, 1770, 1515, 1351cm–1; HRMS (ESI) [M+Na]+ calcd for C9H8N2O5Na: 247.03309, found: 247.03398.
Methyl thiophene-2-carbonylcarbamate (4e): Rf = 0.20 (hexane/EtOAc = 2/1); 1H NMR (400 MHz, CDCl3) δ 7.96 (s, 1H), 7.65-7.62 (m, 2H), 7.14 (dd, J = 3.9 Hz, 3.9 Hz, 1H), 3.87 (s, 3H); 13C NMR (100 MHz, CDCl3): δ 158.7 (C), 151.7 (C), 137.3 (C), 133.1 (CH), 130.4 (CH), 128.1 (CH), 53.3 (CH3); IR(neat) νmax 3273, 2955, 2926, 1749, 1502, 1183 cm–1; HRMS (ESI) [M+Na]+ calcd for C7H7NO3NaS: 208.0044, found: 208.0040.
Methyl furan-2-carbonylcarbamate (4f): 1H NMR (400 MHz, CDCl3) δ 8.35 (bs, 1H), 7.54 (d, J = 1.7 Hz, 1H), 7.35 (d, J = 3.7 Hz, 1H), 6.59 (dd, J = 1.7, 3.7 Hz, 1H), 3.87 (s, 3H); 13C NMR (100 MHz, CDCl3) δ 154.4, 151.1, 146.2, 145.2, 117.7, 113.1, 53.2; IR(neat) νmax 3275, 3135, 2957, 1758, 508, 1205, 1017, 771 cm–1; HRMS (ESI) [M+Na]+ calcd for C7H7NO4Na: 192.02728, found: 192.02745.
Methyl 2-oxo-5,6-dihydro-2H-pyran-3-carbonylcarbamate (6a). To a stirred solution of 5a (50.6 mg, 0.131 mmol) in THF (2 mL) was added Pd2(dba)3 (6.0 mg, 0.007 mmol, 0.05 equiv), Ph3As (12.0 mg, 0.039 mmol, 0.3 equiv), CuTC (74.8 mg, 0.392 mmol, 3.0 equiv) and methyl N-[methoxy(methylthio)methylene] carbamate (42.6 mg, 0.261 mmol, 2.0 equiv) at room temperature, and the resultant solution was stirred at 60 °C. After stirring at 60 °C for 1 h, the reaction mixture was cooled to room temperature, the mixture was filtered through a Celite pad, and the filtrate was concentrated under reduced pressure. The residue was filtered through a short plug of silica gel to afford the imino ether, which was used for the next step without further purification.
To a stirred solution of the imino ether obtained as above in MeCN (3 mL) was added TMSCl (0.03 mL, 0.261 mmol, 2.0 equiv), NaI (58.8 mg, 0.392 mmol, 3.0 equiv) at room temperature. After the reaction was completed, H2O (2 mL) was added to the reaction mixture, and the aqueous layer was extracted with EtOAc (5 mL × 2). The combined organic layers were washed with brine (2 mL), dried (Na2SO4), filtered, and concentrated under reduced pressure. The residue was purified by flash chromatography (CH2Cl2/MeOH = 10/1) to afford 6a (22.6 mg, 87% (2 steps)): Rf = 0.10 (hexane/EtOAc = 1/1); 1H NMR (400 MHz, CDCl3) δ 10.71 (bs, 1H), 8.29 (t, J = 4.4 Hz, 1H), 4.50 (t, J = 6.1 Hz, 2H), 3.82 (s, 3H), 2.78 (dt, J = 6.1, 4.4 Hz, 2H); 13C NMR (100 MHz, CDCl3) δ 164.0, 159.1, 157.2, 151.1, 122.1, 66.3, 52.9, 25.1; IR(neat) νmax 3266, 1778, 1713, 1499, 1188, 1012, 800 cm–1; mp 115 °C; HRMS (ESI) [M+Na]+ calcd for C8H9NO5Na: 222.0378, found: 222.0383.
Methyl 6-oxocyclohex-1-enecarbonylcarbamate (6b): Rf = 0.20 (hexane/EtOAc = 1/1); 1H NMR (400 MHz, CDCl3) δ 10.9 (br, 1H), 8.43 (t, J = 4.4 Hz, 1H), 3.81 (s, 3H), 2.67 (dt, J = 6.8, 4.4 Hz, 2H), 2.59 (t, J = 6.8 Hz, 2H), 2.09 (tt, J = 6.8, 6.8 Hz, 2H); 13C NMR (100 MHz, CDCl3) δ 199.6, 164.4, 160.6, 151.4, 130.0, 52.7, 38.6, 27.0, 21.8; IR(neat) νmax 3235, 2955, 1786, 1715, 1660, 1506, 1229, 1186 cm–1; mp = 87–88 °C; HRMS(FAB) [M+H]+ calcd for C9H12NO4: 198.0766, found: 198.0776.
3-(Tributylstannyl)-2H-pyran-2-one (5c): Rf = 0.80 (hexane/EtOAc = 10/1); 1H NMR (400 MHz, CDCl3) δ 7.43 (dd, J = 2.2, 5.1 Hz, 1H), 7.38 (dd, J = 2.2, 5.9 Hz, 1H), 6.14 (dd, J = 5.1, 5.9 Hz, 1H), 1.55-1.46 (m, 6H), 1.39-1.24 (m, 6H), 1.10-1.02 (m, 6H), 0.90-0.84 (m, 9H); 13C NMR (100 MHz, CDCl3) δ 164.9, 151.6, 151.1, 133.0, 106.6, 28.9, 27.3, 13.6, 9.72; IR(neat) νmax 2954, 2919, 2870, 2851, 1701, 1525, 1081, 957, 776 cm–1; HRMS (ESI) [M+Na]+ calcd for C17H30O2NaSn: 401.11917, found: 401.11950.
Methyl 2-oxo-2H-pyran-3-carbonylcarbamate (6c): Rf = 0.50 (EtOAc); 1H NMR (400 MHz, CDCl3) δ 10.8 (br, 1H), 8.63 (dd, J = 2.2, 6.8 Hz, 1H), 7.80 (dd, J = 2.2, 4.9 Hz, 1H), 6.63 (dd, J = 4.9, 6.8 Hz, 1H), 3.85 (s, 3H); 13C NMR (100 MHz, CDCl3) δ 161.9, 159.4, 156.2, 151.0, 150.2, 117.9, 107.6, 53.0 ; IR(neat) νmax 3239, 3099, 2960, 1779, 1710, 1506, 1280, 1196, 784 cm–1; HRMS (ESI) [M+Na]+ calcd for C8H7NO5Na: 220.02219, found: 220.02181.
4-Methyl-3-(tributylstannyl)-5,6-dihydropyran-2-one (5d): Rf = 0.20 (hexane/EtOAc = 10/1); 1H NMR (400 MHz, CDCl3) δ 4.30 (t, J = 6.1 Hz, 2H), 2.37 (t, J = 6.1 Hz, 2H), 2.03 (s, 3H), 1.59-1.45 (m, 6H), 1.38-1.29 (m, 6H), 1.10-1.01 (m, 6H), 0.94-0.86 (m, 9H); 13C NMR (100 MHz, CDCl3) δ 167.6, 165.0, 130.4, 65.3, 31.0, 28.7, 27.0, 13.4, 13.3, 11,1; IR(neat) νmax 2954, 2922, 2870, 2852, 1706, 1686, 1278, 1153, 766, 668 cm–1; HRMS (ESI) [M+Na]+ calcd for C18H34O2NaSn: 417.15047, found: 417.14964.
Methyl 4-methyl-2-oxo-5,6-dihydro-2H-pyran-3-carbonylcarbamate (6d): Rf = 0.60 (EtOAc); 1H NMR (400 MHz, CDCl3); δ 9.58 (br, 1H), 4.41 (t, J = 6.1 Hz, 2H), 3.78 (s, 3H), 2.62 (t, J = 6.1 Hz, 2H), 2.32 (s, 3H); 13C NMR (100 MHz, CDCl3) δ 163.4, 162.8, 151.6, 130.0, 122.7, 64.9, 52.9, 32.1, 22.5; IR(neat) νmax 3265, 2999, 2957, 1756, 1695, 1496, 1404, 1208, 801 cm–1; HRMS (ESI) [M+Na]+ calcd for C9H11NO5Na: 236.05349, found: 236.05453.
3-Methyl-2-(tributylstannyl)cyclohex-2-enone (5e): Rf = 0.50 (hexane/EtOAc = 10/1); 1H NMR (400 MHz, CDCl3) δ 2.36 (t, J = 6.1 Hz, 2H), 2.34 (t, J = 6.1 Hz, 2H), 2.01 (s, 3H), 1.95 (tt, J = 6.1, 6.1 Hz, 2H), 1.52-1.44 (m, 6H), 1.36-1.27 (m, 6H), 1.00-0.96 (m, 6H), 0.94-0.88 (m, 9H); 13C NMR (100 MHz, CDCl3) δ 202.7, 170.0, 140.5, 36.8, 33.5, 29.0, 27.2, 26.3, 22.5, 13.5, 11.5; IR(neat) νmax 2953, 2922, 2869, 2852, 1646, 1590, 1269, 1188, 669 cm–1; HRMS (ESI) [M+Na]+ calcd for C19H36ONaSn: 45.17121, found: 415.17192.
Methyl 2-methyl-6-oxocyclohex-1-enecarbonylcarbamate (6e): Rf = 0.50 (EtOAc); 1H NMR (400 MHz, CDCl3) δ 9.11 (br, 1H), 3.75 (s, 3H), 2.50 (t, J = 6.6 Hz, 2H), 2.48 (t, J = 6.6 Hz, 2H), 2.13 (s, 3H), 2.03 (tt, J = 6.6, 6.6 Hz, 2H); 13C NMR (100 MHz, CDCl3) δ 196.7, 166.6, 164.9, 151.8, 132.4, 52.8, 37.2, 33.0, 22.8, 21.2; IR(neat) νmax 3265, 2954, 1758, 1693, 1665, 1490, 1201, 775 cm–1; HRMS (ESI) [M+Na]+ calcd for C10H13NO4Na: 2234.07423, found: 234.07524.
(Z)-Methyl 2-(methoxycarbonylcarbamoyl)-3-phenylacrylate (6f): Rf = 0.50 (hexane/EtOAc = 1/1); 1H NMR (400 MHz, CDCl3) δ 8.14 (br, 1H), 7.77 (s, 1H), 7.49 (d, J = 7.6 Hz, 2H), 7.37-7.36 (m, 3H), 3.85 (s, 3H), 3.74 (s, 3H); 13C NMR (100 MHz, CDCl3) δ 164.4, 151.6, 142.0, 132.5, 130.6, 129.7, 128.9, 127.1, 126.8, 53.3, 52.6; IR(neat) νmax 3275, 2954, 1788, 1760, 1685, 1490, 1203, 841, 808 cm–1; HRMS (ESI) [M+Na]+ calcd for C13H13NO5Na: 286.06914, found: 286.06786.
(Z)-Methyl 2-(methoxycarbonylcarbamoyl)-3-(trimethylsilyl)acrylate (6g): Rf = 0.80 (hexane/ EtOAc = 1/1); 1H NMR (400 MHz, CDCl3) δ 9.93 (br, 1H), 7.62 (s, 1H), 3.82 (s, 3H), 3.78 (s, 3H), 0.21 (s, 9H); 13C NMR (100 MHz, CDCl3) δ 165.5, 161.8, 151.5, 124.2, 120.0, 53.0, 52.8, -1.04; IR(neat) νmax 2172, 2955, 1760, 1698, 1489, 1260, 1199, 773, 692 cm–1; HRMS (ESI) [M+Na]+ calcd for C10H17NO5SiNa: 282.07737, found: 282.07832.
(E)-tributyl(1-(methoxymethoxy)but-2-en-2-yl)stannane (5h): Rf = 0.60 (hexane/EtOAc = 20 / 1); 1H NMR (400 MHz, CDCl3) δ 5.70-5.64 (m, 1H), 4.63 (s, 2H), 4.27 (s, 2H), 3.37 (s, 3H), 1.69 (d, J = 6.6 Hz, 3H), 1.51-1.44 (m, 6H), 1.35-1.26 (m, 6H), 0.90-0.84 (m, 15H); 13C NMR (100 MHz, CDCl3) δ 143.7, 134.0, 96.0, 69.1, 55.2, 29.2, 27.4, 15.4, 13.7, 10.0; IR(neat) νmax 2954, 2923, 2871, 2852, 1463, 1153, 1055. 668 cm–1; HRMS (ESI) [M+Na]+ calcd for C18H38O2NaSn: 421.18177, found: 421.18043.
(E)-Methyl 2-((methoxymethoxy)methyl)but-2-enoylcarbamate (6h): Rf = 0.70 (hexane/EtOAc = 20/1); 1H NMR (400 MHz, CDCl3) δ 8.90 (br, 1H), 7.10 (q, J = 7.6 Hz, 1H), 4.68 (s, 2H), 4.35 (s, 2H), 3.80 (s, 3H), 3.43 (s, 3H), 1.91 (d, J = 7.6 Hz, 3H); 13C NMR (100 MHz, CDCl3) δ 164.9, 151.7, 142.7, 130.0, 95.2, 60.2, 55.8, 52.8, 14.0; IR(neat) νmax 3295, 2952, 1763, 1499, 1190, 1147, 1006, 919, 776 cm–1; HRMS (ESI) [M+Na]+ calcd for C9H15NO5Na: 240.08479, found: 240.08462.
(E)-tert-butyldimethyl(3-(tributylstannyl)allyloxy)silane (5i): Rf = 0.70 (hexane/EtOAc = 20 / 1); 1H NMR (400 MHz, CDCl3) δ 6.20 (d, J = 18.8 Hz, 1H), 6.05 (dt, J = 4.1, 18.8 Hz, 1H), 4.21 (d, J = 4.1 Hz, 2H), 1.53-1.46 (m, 6H), 1.35-1.26 (m, 6H), 0.96-0.86 (m, 24H), 0.08 (s, 6H); 13C NMR (100 MHz, CDCl3) δ 147.3, 126.8, 66.7, 29.1, 27.3, 25.9, 18.5, 13.7, 9.4, –5.1; IR(neat) νmax 2954, 2926, 2845, 1462, 1252, 1091, 834, 773 cm–1; HRMS (ESI) [M+Na]+ calcd for C21H46OSiNaSn: 477.22638, found: 2477.22747.
(E)-Methyl 4-(tert-butyldimethylsilyloxy)but-2-enoylcarbamate (6i): Rf = 0.20 (hexane/EtOAc = 2 / 1); 1H NMR (400 MHz, CDCl3) δ 7.65 (br, 1H), 7.18 (dt, J = 3.2, 15.1 Hz, 1H), 7.02 (dt, J = 2.0, 15.1 Hz, 1H), 4.55 (dd, J = 2.0, 3.2 Hz, 2H), 3.80 (s, 3H), 0.94 (s, 9H), 0.1 (s, 6H); 13C NMR (100 MHz, CDCl3) δ 165.6, 152.0, 149.4, 119.5, 622.5, 53.1, 25.8, 18.3, -5.5; IR(neat) νmax 3270, 2954, 2928, 2856, 1770, 1518, 1227, 1064, 835, 777 cm–1; HRMS (ESI) [M+Na]+ calcd for C12H23NO4NaSi: 296.12940, found: 296.13072.
(E)-Methyl 4-(benzyloxy)but-2-enoylcarbamate (6j):1p 1H NMR (400 MHz, CDCl3) δ 7.90 (br, 1H), 7.36-7.26 (m, 5H), 7.14 (dt, J = 3.7, 15.4 Hz, 1H), 7.05 (d, J = 15.4 Hz, 1H). 4.58 (s, 2H), 4.22 (dd, J = 2.2, 3.7 Hz, 2H), 3.79 (s, 3H).
ACKNOWLEDGEMENTS
This work was financially supported in part by The Grant-in-Aid for Scientific Research on Innovative Areas “Organic Synthesis based on Reaction Integration. Development of New Methods and Creation of New Substances” (No. 2105).
References
1. [hydrazoic acid]: a) J. K. Myers and E. N. Jacobsen, J. Am. Chem. Soc., 1999, 121, 8959; CrossRef [activated methylene compounds]: b) M. S. Taylor and E. N. Jacobsen, J. Am. Chem. Soc., 2003, 125, 11204; CrossRef [hydrazoic acid, cyanide, activated methylene compounds]: c) M. S. Taylor, D. N. Zalatan, A. M. Lerchner, and E. N. Jacobsen, J. Am. Chem. Soc., 2005, 127, 1313; CrossRef [malononitrile]: d) Y. Hoashi, T. Okino, and Y. Takemoto, Angew. Chem. Int. Ed., 2005, 44, 4032; CrossRef [activated methylene compounds]: e) T. Inokuma, Y. Hoashi, and Y. Takemoto, J. Am. Chem. Soc., 2006, 128, 9413; CrossRef [cyanide]: f) G. M. Sammis and E. N. Jacobsen, J. Am. Chem. Soc., 2003, 125, 4442; CrossRef g) G. M. Sammis, H. Danjo, and E. N. Jacobsen, J. Am. Chem. Soc., 2004, 126, 9928; CrossRef h) C. Mazet and E. N. Jacobsen, Angew. Chem. Int. Ed., 2008, 47, 1762; CrossRef [hydroxylamine]: i) M. P. Sibi, N. Prabagaran, S. G. Ghorpade, and C. P. Jasperse, J. Am. Chem. Soc., 2003, 125, 11796; CrossRef [oxime]: j) C. D. Vanderwal and E. N. Jacobsen, J. Am. Chem. Soc., 2004, 126, 14724; CrossRef [arylthiol]: k) B.-J. Li, L. Jiang, M. Liu, Y.-C. Chen, L.-S. Ding, and Y. Wu, Synlett, 2005, 603; CrossRef [N-heterocycles]: l) M. Gandelman and E. N. Jacobsen, Angew. Chem., Int. Ed., 2005, 44, 2393; CrossRef [1,3-dipole]: m) M. P. Sibi, Z. Ma, K. Itho, and N. Prabagaran, Org. Lett., 2005, 7, 2349; CrossRef [radical addition]: n) M. P. Sibi, G. Petrpvic, and J. Zimmerman, J. Am. Chem. Soc., 2005, 127, 2390; CrossRef [hydrazine]: o) M. P. Sibi and T. Soeta, J. Am. Chem. Soc., 2007, 129, 4522; CrossRef [amine]: p) L. L. Taylor, F. W. Goldberg, and K. K. Hill, Org. Biomol. Chem., 2012, 10, 4424. CrossRef
2. For example, see: L. Yao, B. Pitta, P. C. Ravikumar, M. Purzycki, and F. F. Fleming, J. Org. Chem., 2012, 77, 3651. CrossRef
3. For example, see: M. P. DeNinno, C. Eller, and J. B. Etienne, J. Org. Chem., 2001, 66, 6988. CrossRef
4. a) H. Bredereck, G. Simchen, and E. Göknel, Angew. Chem., Int. Ed., Engl., 1964, 3, 704; CrossRef b) J. C. Martin, J. L. Chitwood, and P. G. Gott, J. Org. Chem., 1971, 36, 2228; CrossRef c) E. P. Papadopoulos, J. Org. Chem. 1974, 39, 2540; CrossRef d) H. Hagemann, Angew. Chem., Int. Ed. Engl., 1977, 16, 743; CrossRef e) W. Kiemstedt and W. Sundermeyer, Chem. Ber., 1982, 115, 919; CrossRef f) G. R. Lenz, C. M. Woo, and B. L. Hawkins, J. Org. Chem., 1982, 47, 3049; CrossRef g) P. Magnus and B. Mugrage, J. Am. Chem. Soc., 1990, 112, 462. CrossRef
5. a) L. S. Liebeskind and J. Srogl, J. Am. Chem. Soc., 2000, 122, 11260; CrossRef b) R. Wittenberg, J. Srogl, M. Egi, and L. S. Liebeskind, Org. Lett., 2003, 5, 3033; CrossRef c) Y. Yu and L. S. Liebeskind, J. Org. Chem., 2004, 69, 3554; CrossRef d) H. Prokopcov and C. O. Kappe, Angew. Chem. Int. Ed., 2009, 48, 2276. CrossRef
6. a) D. Seyferth and F. G. A. Stone, J. Am. Chem. Soc., 1957, 79, 515; CrossRef b) J. A. Soderquist and A. Hassner, J. Am. Chem. Soc., 1980, 102, 1577; CrossRef c) D. E. Seitz and S.-H. Lee, Tetrahedron Lett., 1981, 22, 4909; CrossRef d) A. Amamria and T. N. Mitchell, J. Organomet. Chem., 1980, 199, 49; CrossRef e) H. Azizian, C. Eaborn, and A. Pidcock, J. Organomet. Chem., 1981, 215, 49; CrossRef f) Y. Ichinose, H. Oda, K. Oshima, and K. Utimoto, Bull. Chem. Soc. Jpn., 1987, 60, 3468; CrossRef g) J. K. Stille, Angew. Chem., Int. Ed. Engl., 1986, 25, 508. CrossRef
7. V. P. Baillargeon and J. K. Stille, J. Am. Chem. Soc., 1983, 105, 7175. CrossRef
8. J. A. Soderquist and W. W.-H. Leong, Tetrahedron Lett., 1983, 24, 2361. CrossRef
9. L. Balas, B. Jousseaume, H. Shin, J.-B. Verlhac, and F. Wallian, Organometallics, 1991, 10, 366. CrossRef
10. F. J. Cuadrado, M. A. Pérez, and J. L. Soto, J. Chem. Soc., Perkin Trans. 1, 1984, 2447. CrossRef
11. C.-K. Pei, X.-C. Zhang, and M. Shi, Eur. J. Org. Chem., 2011, 23, 4479. CrossRef
12. T. Tomizawa, K. Orimoto, H. Oyama, T. Niwa, and M. Nakada, Org. Lett., 2012, 14, 6294. CrossRef
13. K. Orimoto, H. Oyama, Y. Namera, T. Niwa, and M. Nakada, Org. Lett., 2013, 768. CrossRef
14. J. Vidal, S. Damestoy, L. Guy, J.-C. Hannachi, A. Aubry, and A. Collet, Chem. Eur. J., 1997, 3, 1691. CrossRef
15. S. Vijgen, K. Nauwelaerts, J. Wang, A. V. Aerschot, I. Lagoja, and P. Herdewijn, J. Org. Chem., 2005, 70, 4591. CrossRef
16. N. B. Carter, R. Mabon, A. M. E. Richecœur, and J. B. Sweeney, Tetrahedron, 2002, 58, 9117. CrossRef
17. V. A. Vu, L. Berillon, and P. Knochel, Tetrahedron Lett., 2001, 42, 6847. CrossRef
18. E. Negishi, Z. Tan, S. Y. Liou, and B. Liao, Tetrahedron, 2000, 56, 10197. CrossRef
19. P. Zhong, Z.-X. Xiong, and X. Huang, Synth. Commun., 2001, 31, 311. CrossRef
20. H. X. Zhang, F. Guibe, and G. Balavoine, J. Org. Chem., 1990, 55, 1857. CrossRef
21. J. F. Betzer, F. Delaloge, B. Muller, A. Pancrazi, and J. Prunet, J. Org. Chem., 1997, 62, 7768. CrossRef
22. A. Darwish, A. Lang, T. Kim, and J. M. Chong, Org. Lett., 2008, 10, 861. CrossRef
23. Y. Yamamoto, J. Ishihara, N. Kanoh, and A. Murai, Synthesis, 2000, 1894. CrossRef