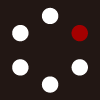
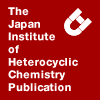
HETEROCYCLES
An International Journal for Reviews and Communications in Heterocyclic ChemistryWeb Edition ISSN: 1881-0942
Published online by The Japan Institute of Heterocyclic Chemistry
e-Journal
Full Text HTML
Received, 6th March, 2013, Accepted, 9th April, 2013, Published online, 16th April, 2013.
DOI: 10.3987/COM-13-12695
■ Unexpected Behaviour of 6-Cyanopurines Towards Secondary Amines
Amal Al-Azmi* and K. Anita Kumari
Department of Chemistry, Faculty of Science, Kuwait University, Safat 13060, Kuwait
Abstract
Reaction of 6-cyanopurines with excess dimethylamine and excess piperidine at room temperature or by reflux-respectively-yielded a mixture of 6-purinecarboximidamides and N,N-dialkylpurines or piperidin-1-yl(purin-6-yl)methanimine and (piperidin-1-yl)-9H-purine, respectively. 6-Purinecarboximidamides were initially formed from the reaction, whereas N,N-dialkylpurines were detected in the mixture 30 min afterward. Both purines appeared to be generated from different mechanistic pathways.Over the last few decades, a large number of 6-substituted purines, including N,N-dialkylpurine derivatives, have been produced for their various biological activities1-23 with their activities depending mainly on the type and position of substituents available. Several N,N-dialkylpurine derivatives have been reported in the literature to act as anti-inflammatory, anti-allergic, and antirhinoviral agents whereas others have been reported to act as antibacterial agents for Mycobacterium turberculosis strain H37Rv. Other derivatives have been used successfully for the treatment of Alzheimer’s disease.24,25
Proneça23,24 described two different synthetic routes for N,N-dialkylpurinederivatives: (1) reaction of 5-amino-4-cyanoformimidoylimidazole 2 and N,N-dimethylformamide diethyl acetal followed by addition of excess secondary alkylamine or (2) treatment of 5-amino-4-cyanoformimidoylimidazole 2 with a secondary alkylamine and then addition of either N,N-dimethylformamide diethyl acetal, salicyladehyde, or 4-hydroxybenzaldehyde.
Over the last few years, our group has investigated the reactions of 6-cyanopurines with primary amines.4,26 6-Cyanopurine 1 has proven to be a fruitful precursor for new and novel transformations (Scheme 1).
However, to the best of our knowledge, the direct reaction of 6-cyanopurines with secondary amines has yet to be examined. This paper describes the reaction of 6-cyanopurine 1 with dimethylamine and piperidine.
When a mixture of 1a and excess dimethylamine was stirred at room temperature, formation of a product occurred within 1 min to 2 min, as shown by TLC. Stirring was continued to drive the reaction to completion. Approximately 30 min later, TLC again indicated the formation of another product. The starting material was fully consumed within 5 d, and the two products were separated by column chromatography. Reaction of 6-cyanopurine 1a with dimethylamine was carried out in an attempt to isolate pyrimidine 10, as shown in Scheme 3, Route A. Dimethylamine was assumed to behave in a manner similar to methylamine by opening the imidazole ring.4,15 IR and 13C NMR spectra of the isolated solids showed the disappearance of the cyano group. Both products were fully characterised by spectroscopic analyses and identified as N6,N6-dimethyl-9-(4-methoxyphenyl)-9H-6-purinecarboximidamide (Rf = 0.78) 11a, and N-[9-(4-methoxyphenyl)-9H-6-purinyl]-N,N-dimethylamine 12a (Rf = 0.53) in 2:1 (EtOAc:hexane). Another derivative, 1b, was also made to react with excess dimethylamine, generating the purines 11b and 12b, as shown in Scheme 2, Table 1.
Isolation of purines 11a and 11b suggested that the previously reported mechanism for the reaction of methylamine with 6-cyanopurines could be interpreted via a different approach.
Previous papers4,15 reported that imidazole ring opening by methylamine and attack on the electrophilic carbon of the cyano group are necessary to generate pyrimidopyrimidine. However, isolation of 11a and 11b indicated that the mechanism may actually start by nucleophilic attack of the amine on the cyano group (Scheme 3, Route B).
Purines 11 and 12 were clearly produced by two reaction intermediates. Formation of purine 11 represents an example of a nucleophilic addition reaction, whereas formation of purine 12 shows an example of a typical SNAr reaction. Purines 12a and 12b were previously believed to form when excess dimethylamine reacts with purines 11a and 11b, causing displacement of the carboximidine moiety. Purines 11a and 11b were stirred with excess dimethylamine at room temperature for 7 d, and their reaction was monitored by TLC. No change in the composition of the primary reaction mixture was observed.
To generalise this observation, another two reactions were carried out and it was found that both purines 1a,b furnished the two expected products 13a,b and 14a,b, when refluxed with piperidine (Scheme 4, Table 1).
Like the previous reactions in Scheme 2, the SNAr products 14a,b were the major for both 1a and 1b.
In conclusion, this paper describes a mild, efficient, and direct method for preparing two 6-substituted purines from easily made 6-cyanopurines. The products obtained showed the presence of two competing reaction mechanisms, affording two different purines with acceptable yields.
EXPERIMENTAL
General
6-Cyanopurines 1a and 1b were prepared according to literature procedures.4 1H NMR spectra were recorded on a Bruker DPX 400 spectrometer at 400 MHz. CDCl3 or DMSO-d6 was used as the solvent, and tetramethylsilane was used as the internal standard. Chemical shifts are reported in δ units (ppm). 13C NMR spectra were obtained using a Bruker DPX 400 spectrometer at 100 MHz. Mass spectra were recorded on a VG autospec Q spectrometer with digital data output. IR spectra were recorded on Ftir-jASCO-ft/ir-6300 instrument using KBr discs. The (νmax) is recorded in cm-1. Melting points were determined by a Gallenkamp melting point apparatus and are reported uncorrected. TLC was performed on 0.25 mm pre-coated silica gel plates (Merck).
Reaction of 6-cyanopurine 1a with dimethylamine
Excess dimethylamine (20 mmol) was added to 6-cyanopurine 1a (0.28 g, 1.12 mmol), and the suspension was stirred at room temperature. After a few minutes, the mixture yielded a clear solution. TLC showed the presence of two products: one (Rf = 0.78) appearing 1 min to 2 min after stirring and the other (Rf = 0.53) (EtOAc:hexane, 2:1) appearing 30 min later. Stirring was continued until TLC indicated completion of the reaction 5 d. The resulting solids, which were composed of the two products, were filtered off, and products were separated by column chromatography starting with a mobile phase of 1:9 EtOAc:hexane, gradually increasing to 100% EtOAc.
N6,N6-Dimethyl-9-(4-methoxyphenyl)-9H-6-purinecarboximidamide 11a: Yellow needles (0.11 g, 0.37 mmol, 33%), mp 160-163 °C [Found accurate mass: 296.1380; m/z (EI) (M+) 296, 100%; C15H16N6O requires: 296.1380; M 296]; δH 400 MHz (DMSO-d6, TMS) 3.32 (s, 6H, N(CH3)2), 3.75 (s, 3H, OCH3), 6.93 (d, 2H, J 8.8 Hz, ArH), 7.85 (d, 2H, J 9.2 Hz, ArH), 8.50 (s, 1H, CH), 8.53 (s, 1H, CH), 9.82 (s, 1H, NH); δC 100 MHz (DMSO-d6, TMS) 158.30, 156.69, 155.63, 153.51, 151.80, 134.12, 133.07, 131.65, 123.01, 113.70, 55.24, 40.82; νmax: (KBr) 3318, 2925, 1605, 1541, 1411, 1240, 1180, 1008, 835 cm−1.
N-[9-(4-Methoxyphenyl)-9H-6-purinyl]-N,N-dimethylamine 12a: Cream powder (0.11 g, 0.40 mmol, 36%), mp 166-169 °C [Found accurate mass: 269.1271; m/z (EI) (M+) 269, 100%; C14H15N5O requires: 269.1271; M 269]; δH 400 MHz (DMSO-d6, TMS) 3.32 (s, 6H, N(CH3)2), 3.82 (s, 3H, OCH3), 7.12 (d, 2H, J 9.2 Hz, ArH), 7.71 (d, 2H, J 9.2 Hz, ArH), 8.24 (s, 1H, CH), 8.46 (s, 1H, CH); δC 100 MHz (DMSO-d6, TMS) 158.56, 154.48, 152.35, 150.08, 138.77, 127.88, 125.08, 119.56, 114.58, 55.54, 40.93; νmax: (KBr) 3090, 2921, 1598, 1521, 1430, 1258, 1183, 1070, 970, 821 cm−1.
Reaction of 6-cyanopurine 1b with dimethylamine
A methanolic solution of dimethylamine (10 mL, 20 mmol) was added to 6-cyanopurine 1b (0.47 g, 2.0 mmol), and the suspension was stirred at room temperature. After 10 min to 15 min, the reaction mixture yielded a light brown clear solution. Stirring was continued until the reaction reached completion, approximately 2 d to 3 d. The reaction mixture revealed the presence of two solids. Addition of MeOH dissolved one of the solids, leaving the other product undissolved for collection by filtration. The second product was obtained by concentration of the MeOH layer followed by crystallisation from acetone.
N6,N6-Dimethyl-9-(4-methylphenyl)-9H-6-purinecarboximidamide 11b: Light brown crystals, (0.22 g, 0.78 mmol, 39%), mp 161-165 °C [Found accurate mass: 280.1430; m/z (EI) (M+) 280, 100%; C15H16N6 requires: 280.1430; M 280]; δH 400 MHz (DMSO-d6, TMS) 2.29 (s, 3H, CH3), 3.35 (s, 6H, N(CH3)2), 7.16 (d, 2H, J 8.4 Hz, ArH), 7.85 (d, 2H, J 8.4 Hz, ArH), 8.52 (s, 1H, CH), 8.53 (s, 1H, CH), 9.78 (s, 1H, NH); δC 100 MHz (DMSO-d6, TMS) 158.26, 156.67, 153.56, 151.67, 136.11, 134.10, 133.11, 132.60, 128.97, 121.18, 40.86, 20.55; νmax: (KBr) 3329, 1598, 1576, 1525, 1447, 1409, 1359, 1234, 1003, 822, 626 cm−1.
N,N-Dimethyl-N-[9-(4-methylphenyl)-9H-6-purinyl]amine 12b: Off-white powder (0.27 g, 1.06 mmol, 54%), mp 136-139 °C [Found accurate mass: 253.1321; m/z (EI) (M+) 253, 100%; C14H15N5 requires: 253.1321; M 253]; δH 400 MHz (DMSO-d6, TMS) 2.38 (s, 3H, CH3), 3.35 (s, 6H, N(CH3)2), 7.37 (d, 2H, J 8.4 Hz, ArH), 7.71 (d, 2H, J 8.4 Hz, ArH), 8.25 (s, 1H, CH), 8.51 (s, 1H, CH); δC 100 MHz (DMSO-d6, TMS) 154.48, 152.39, 149.99, 138.58, 137.10, 132.54, 129.86, 123.23, 121.21, 119.72, 37.96, 20.64; νmax: (KBr) 3100, 2923, 1598, 1517, 1421, 1296, 974, 810, 641 cm−1.
Reaction of 6-cyanopurine 1a and 1b with piperidine
Excess of piperidine was added to 6-cyanopurine 1a (0.6 g, 2.39 mmol), or 1b (0.52 g, 2.21 mmol). The mixture was refluxed for 1 h (1a) and 3 h (1b). Two new spots were detected using TLC and the reaction reached completion. The mixture was concentrated and the residue was dissolved with a minimum amount of DCM, then excess of hexane and left to precipitate (24 h). The solids formed were filtered off, and separated by column chromatography starting with a mobile phase of hexane, and gradually increasing to 100% EtOAc.
(9-(4-Methoxyphenyl)-9H-purin-6-yl)(piperidin-1-yl)methanimine 13a: Cream powder (0.23 g, 0.68 mmol, 29%), mp 173-176 °C [Found accurate mass: 336.1694; m/z (EI) (M+) 336, 42%; C18H20N6O requires: 336.1693; M 336]; δH 400 MHz (DMSO-d6, TMS) 1.65 (s, 6H, 3CH2), 3.76 (s, 3H, OCH3), 4.35 (s, 4H, 2CH2), 6.94 (d, 2H, J 8.8 Hz, ArH), 7.85 (d, 2H, J 9.2 Hz, ArH), 8.50 (s, 1H, CH), 8.53 (s, 1H, CH), 9.85 (s, 1H, NH); δC 100 MHz (DMSO-d6, TMS) 157.50, 156.91, 155.65, 153.54, 151.78, 134.40, 132.94, 131.59, 123.05, 113.68, 55.23, 48.04, 26.01, 24.19; νmax: (KBr) 3447, 3344, 3102, 3043, 3019, 2940, 2917, 2853, 1601, 1578, 1543, 1517, 1505 cm−1.
9-(4-Methoxyphenyl)-6-(piperidin-1-yl)-9H-purine 14a: light red crystals (0.28 g, 0.9 mmol, 38%), mp 151-155 °C [Found accurate mass: 309.1583; m/z (EI) (M+) 309, 100%; C17H19N5O requires: 309.1584; M 309]; δH 400 MHz (DMSO-d6, TMS) 1.56 (m, 4H, 2CH2), 1.65 (m, 2H, CH2), 3.83 (s, 3H, OCH3), 4.23 (s, 4H, 2CH2), 7.11 (d, 2H, J 8.8 Hz, ArH), 7.70 (d, 2H, J 8.8 Hz, ArH), 8.24 (s, 1H, CH), 8.45 (s, 1H, CH), δC 100 MHz (DMSO-d6, TMS) 158.56, 153.30, 152.40, 150.32, 138.65, 127.82, 125.12, 119.22, 114.54, 55.51, 45.72, 25.72, 24.29; νmax: (KBr) 3445, 3103, 3073, 3023, 2978, 2927, 2915, 2847, 1921, 1888, 1698, 1650, 1576, 1558, 1521 cm−1.
Piperidin-1-yl(9-p-tolyl-9H-purin-6-yl)methanimine 13b: off-white powder (0.082 g, 0.25 mmol, 12%), mp 169-172 °C [Found accurate mass: 320.1743; m/z (EI) (M+) 320, 100%; C18H20N6 requires: 320.1743; M 320]; δH 400 MHz (DMSO-d6, TMS) 1.64 (m, 6H, 3CH2), 2.29 (s, 3H, CH3), 4.33 (s, 4H, 2CH2), 7.16 (d, 2H, J 8.4 Hz, ArH), 7.85 (d, 2H, J 8.4 Hz, ArH), 8.52 (s, 1H, CH), 8.53 (s, 1H, CH), 9.81 (s, 1H, NH); δC 100 MHz (DMSO-d6, TMS) 157.48, 156.90, 153.59, 151.66, 136.03, 134.38, 132.99, 132.64, 128.92, 121.24, 48.03, 26.00, 24.15, 20.51; νmax: (KBr) 3349, 3038, 2963, 2919, 2855, 1902, 1598, 1536, 1443 cm−1.
6-(Piperidin-1-yl)-9-p-tolyl-9H-purine 14b: light yellow powder (0.44 g, 1.5 mmol, 69%), mp 141-145 °C [Found accurate mass: 293.1634; m/z (EI) (M+) 293, 100%; C17H19N5 requires: 293.1634; M 293]; δH 400 MHz (DMSO-d6, TMS) 1.60 (m, 4H, 2CH2), 1.69 (m, 2H, CH2), 2.39 (s, 3H, CH3), 4.23 (s, 4H, 2CH2), 7.37 (d, 2H, J 8 Hz, ArH), 7.71 (d, 2H, J 8.4 Hz, ArH), 8.26 (s, 1H, CH), 8.51 (s, 1H, CH); δC 100 MHz (DMSO-d6, TMS) 153.31, 152.45, 150.24, 138.49, 137.12, 132.47, 129.82, 123.31, 119.36, 45.66, 25.72, 24.28, 20.62; νmax: (KBr) 3447, 3106, 3081, 3044, 3021, 2924, 2862, 2848, 1590, 1578, 1554, 1524 cm−1.
ACKNOWLEDGMENTS
The authors gratefully acknowledge Kuwait University and SAF Facility for financially supporting their work through Research Grant No. SC04/09 and Project Nos. GS 01/01 and GS 03/01.
References
1. J. H. Lister, in The Chemistry of Heterocyclic Compounds, Vol. 24 (II); Fused Pyrimidines, Part II, Purines, ed. by E. C. Taylor and A. Weissberger, Wiley-Interscience, New York, 1971, 380.
2. K. T. Potts, in Comprehensive Heterocyclic Chemistry, ed. by A. R. Katritzky and C. W. Rees, Pergamon Press, 1984, 5, 548.
3. C. A. Ramsden, in Comprehensive Heterocyclic Chemistry II, ed. by A. R. Katritzky, C. W. Rees, and E. F. V. Scriven, Pergamon, 1996, 7, 414.
4. A. Al-Azmi, B. L. Booth, R. A. Carpenter, A. Carvalho, E. Marrelec, R. G. Pritchard, and F. J. R. P. Proença, J. Chem. Soc., Perkin Trans. 1, 2001, 2532. CrossRef
5. M. A. Carvalho, T. M. Esteves, M. F Proença, and B. L. Booth, Org. Biorg. Chem., 2004, 2, 1019. CrossRef
6. a) M. A. Carvalho, S. Esperança, T. M. Esteves, and M. F. Proença, Eur. J. Org. Chem., 2007, 1324; CrossRef b) M. J. Alves, B. L. Booth, M. A. Carvalho, R. G. Pritchard, and F. J. R. P. Proença, J. Heterocycl. Chem., 1997, 34, 739. CrossRef
7. M. Hocek, M. Masojidkova, A. Holy, A. Graciela, R. Snoeck, J. Balzarini, and E. DeClerq, Collect. Czech. Chem. Commun., 1996, 61, 1525. CrossRef
8. T. E. Mabry, C. D. Jones, T. S. Chou, J. M. Colacino, G. B. Grindey, J. F. Worzalla, and H. L. Pearce, Nucleosides Nucleotides, 1994, 13, 1125. CrossRef
9. J. L. Kelley, D. C. Wilson, V. L. Styles, F. S. Soroko, and B. R. Cooper, J. Heterocycl. Chem., 1995, 32, 1417. CrossRef
10. A. Al-Azmi, P. George, and O. M. E. El. Dusouqui, J. Heterocycl. Chem., 2007, 44, 515. CrossRef
11. W. Pendergast and W. R. Hall, J. Heterocycl. Chem., 1989, 26, 1863. CrossRef
12. A. Al-Azmi, A. A.-Elassar, and B. L. Booth, Tetrahedron, 2003, 59, 2749. CrossRef
13. A. Al-Azmi, J. Chem. Res., 2005, 8, 530. CrossRef
14. A. Al-Azmi, B. L. Booth, R. G. Pritchard, and F. J. R. P. Proença, J. Chem. Soc., Perkin Trans. 1, Comm., 2001, 485. CrossRef
15. M. J. Alves, B. L. Booth, M. A. Carvalho, R. G. Pritchard, and F. J. R. P. Proença, J. Heterocycl. Chem., 1997, 34, 739. CrossRef
16. M. A. Carvalho, M. E. A Zaki, Y. Álvares, M. F. Proença, and B. L. Booth, Org. Biomol. Chem., 2004, 2, 2340. CrossRef
17. B. L. Booth, A. M. Dias, and M. F. Proença, J. Chem. Soc., Perkin Trans. 1, 1992, 2119. CrossRef
18. M. J. Alves, B. L. Booth, and M. F. Proença, J. Chem. Soc., Perkin Trans. 1, 1990, 1705. CrossRef
19. M. J. Alves, B. L. Booth, A. P. Freitas, and M. F. Proença, J. Chem. Soc., Perkin Trans. 1, 1992, 913. CrossRef
20. A. P. Freitas, M. F. Proença, and B. L. Booth, J. Heterocycl. Chem., 1995, 32, 457. CrossRef
21. M. E. A. Zaki and M. F. Proença, Tetrahedron, 2007, 63, 3745. CrossRef
22. M. E A. Zaki, M. F. Proença, and B. L Booth, J. Org. Chem., 2003, 6, 276. CrossRef
23. M. E. A. Zaki, M. F. Proença, and B. L. Booth, Synlett, 2005, 2429. CrossRef
24. M. J. Alves, M. A. Carvalho, S. Carvalho, A. M. Dias, F. H. Fernandes, and M. F. Proneça, Eur. J. Org. Chem., 2007, 4881. CrossRef
25. C. Correis, M. A. Carvalho, and M. F. Proneça, Tetrahedron, 2009, 65, 6903. CrossRef
26. A. Al-Azmi and K. Anita Kumari, Heterocycles, 2009, 78, 2245. CrossRef