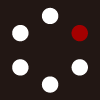
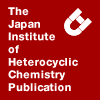
HETEROCYCLES
An International Journal for Reviews and Communications in Heterocyclic ChemistryWeb Edition ISSN: 1881-0942
Published online by The Japan Institute of Heterocyclic Chemistry
e-Journal
Full Text HTML
Received, 20th August, 2013, Accepted, 27th September, 2013, Published online, 16th October, 2013.
DOI: 10.3987/COM-13-12813
■ Synthesis of 1,4-Diarylfluorenone and 1,4-Diarylfluorene
Yanmei Wang, Qiancai Liu,* Xiaoli Xiong, Shiming Deng, Jun Zhang, Min Zhu, and Hangmin Ge
Department of Chemistry, East China Normal University, 500 Dongchuan Rd., Minhang, Shanghai 200241, China
Abstract
A novel synthetic route to 1,4-diarylfluorenone and 1,4-diarylfluorene is presented by starting with ninhydrin. The retro-Diels-Alder cycloaddition was employed as a key step to construct the target fluorenones, while a highly efficient reduction from 1,4-diarylfluorenones to 1,4-diarylfluorenes is developed by using of sodium sulfide nonahydrate as reductant. The final products are confirmed by 1H NMR, 13C NMR and mass spectrometry.Semiconducting -conjugated systems have attracted much attention as futuristic materials for the development and production of the next generation of opt-electrics. A great number of π-conjugated semiconducting materials that have either been discovered or synthesized generate an exciting library of π-conjugated systems for use in the fields of organic light-emitting diodes (OLEDs), organic field-effect transistors (OFETs) and organic solar cells. Fluorene and its derivatives are useful components for construct small organic molecules and polymers suitable for organic optoelectronics. Heterocycles such as thiophene and its derivatives are often contained or incorporated in these π-conjugated systems, and they could play important roles in the properties of the materials for OLEDs, OFET or solar cells.1 2,7-disubstituted-fluorenes are often used as subunit both in polymers and small organic molecules applicable for material chemistry, for example, 2,7-bis(4-bromophenyl)-9,9-dialkyfluorenes and 2,7-bis(5-bromothien-2-yl)-9,9-dialkyfluorenes have been used to afford polymers suitable for organic electroluminescent devices and organic light-emitting diodes (OLEDs), while charge-transporting polymers with high hole-transporting ability, excellent solubility and excellent film-forming properties from the same monomer have also been reported.2 Conventional multistep procedures are often required for synthesis of fluorene-based compounds.3 Some thiophene-fused indeno-spirofluorenes have been reported for the construction of optoelectronic materials in few cases.4 Compared to their 2,7-diarylated congeners, 1,4-diarylfluorenes are barely reported.5 The above reasons led us to initiate our efforts on the study of 1,4-diarylated fluorenes, which might be used as alternative building blocks for further functional transformations.
As a part of our ongoing project on heterocycle-cooperated or substituted fluorenes,6 we describe here the latest progress on 1,4-diarylfluorenones and 1,4-diarylfluorenes through well-defined synthetic routes and utilization of sodium sulfide nonahydrate as reductant for fluorenones. The scope and synthetic applicability are quite versatile for (poly)arylated fluorenones and the results presented here afford an alternative route to fluorene-based materials, which should allow the formation of fluorene-based semiconducting materials.
It is difficult to construct 1,4-diarylfluorenones by regular synthetic route due to the limited availabilty of suitable precursors (e.g. 1,4-dihalogenated florenone or 1,4-dihydroxyfluorenone as substrates for transition metal-catalyzed cross coupling reactions). In order to prepare 1,4-diarylfluorenones and 1,4-diarylfluorenes which might be useful as subunits for material chemistry, 2,8-dioxo-1,3-diaryl-2,8-dihydrocyclopenta[a]indenes (indanocyclones 3a-c) were evaluated as building block because 3a has been used as a promising block to construct substituted fluorenones as reported by us or others.7 For example, we have reported indenospirobifluorenes and dispirobifluorenes through indenofluorenone constructed from the reaction of 3a with suitable dienophiles by using of Diels-Alder cycloaddition as a major tool.7b Bhattacharya et al. have reported that indanocyclone could be used to construct highly arylated fluorenones, which were reduced to the corresponding fluorenes by PhOH/aq. HI/cat. red phosphorous system.5 We have also reported that this type of carbonyl reduction could be finished by Wolff-Kishner-Huang reduction in the case of indenospirbifluorenes.7b
In order to expand the application of these type of precursors to construct different flurenones, the diarylacetones 2b and 2c have been synthesized by the condensation of 4-bromophenylacetic acid or 2-bromophenylacetic acid in the presence of dicyclohexylcarbodiiimide (DCC) and 4-dimethyl- aminopyridine (DMAP) in anhydrous dichloromethane (DCM) by literature report.8 Compounds 3b and 3c could be easily accessible by the reaction of ninhydrin with 1,3-diarylacetone under basic conditions similar to 3a.8 Then the retro-Diels-Alder reaction between indanocyclones (3a, 3b and 3c) and norbonadiene in either refluxing toluene (for 3a and 3b) or xylene (for 3c) afforded compounds 4a-4c (isolated yields 60-75%). Compounds 4d and 4e could be obtained by palladium-catalyzed Suzuki cross-coupling reactions of 4b or 4c with 2-thienylboronic acid. Generally Wolff-Kishner-Huang reduction is considered as one of the most efficient tools to convert carbonyl groups of aromatic ketones (e.g. fluorenone) to methylene, except those with halogenated aromatic rings. We found that sodium sulfide nonahydrate (Na2S∙9H2O) could act as a sulfur surrogate to construct thiophene ring and a reductant for reduction of fluorenone to fluorene albeit in low yield (46%).9 The reason for this reduction might be due to the formation of H2S from sodium sulfide nonahydate in refluxing NMP. The role of H2S as a reductant for aromatic ketone reduction under high pressure with catalyst has been reported before albeit with low yields in general.10 We consider that the reduction with Na2S∙9H2O might also be applicable for the reduction of 1,4-diarylfluorenones. It is proved that Na2S∙9H2O is highly sufficient for the reduction of 1,4-diarylfluorenone (4a-4e) to 1,4-diarylfluorenes (5a-5e) in refluxing N-methl-2- pyrrolidone (NMP). The isolated yields of 5a, 5d and 5e are higher than 70%. While for 5b and 5c, the isolated yields are 29.5% and 26%, respectively.
All of 4a-4e and 5a-5e are confirmed by 1H NMR and 13C NMR and part of them are also proved by mass spectrometry.
In conclusion, we have developed a novel route to 1,4-diraylfluorenones and 1,4-diarylfluorenes, which are barely reported in literatures. The key step for synthesis of 1,4-diraylfluorenones is Diels-Alder reaction between indanocyclones and norbornadiene, and the retro-Diels-Alder reaction of the adduct with the elimination of CO. While an efficient reduction reaction has been developed by employing Na2S∙9H2O in refluxing NMP for reduction of 1,4-diarylfluorenones to 1,4-diarylfluorenes, which might be potentially applicable to reduction of other highly arylated fluorenones with or without halide substituents.
EXPERIMENTAL
1. Instruments and materials
All manipulations for air- and moisture-sensitive operations are conducted by using of standard Schlenk techniques. All chemicals are available from commercial sources and used without further purification. Solvents are purified according to standard methods prior to use. Melting point was measured on an X-4 micrographic melting point apparatus. 1H NMR and 13C NMR spectra were measured on a Bruker DR × 300 MHz spectrometer or 400 MHz spectrometer (1H NMR 300 MHz, 13C NMR 75 MHz or 100 MHz). Mass spectra were measured on micOTOF II (ESI) spectrometer and Agilent 5973N mass spectrometer (EI).
2. Synthesis
1) Synthesis of 1,3-diarylacetones 2b and 2c: Compound 2a is commercially available, and 2b and 2c are prepared as reported.8
a) Preparation of 1,3-di(4-bromophenyl)-2-propanone (2b)8
To a mixture of dicyclohexylcarbodiimide (DCC) (7.7 g, 37.3 mmol) and 4-dimethylaminopyridine (DMAP) (1.145 g, 9.35 mmol) in CH2Cl2 (75 mL) was added slowly a solution of 4-bromophenylacetic acid (8.05 g, 37.45 mmol) in CH2Cl2 (75 mL). After addition, it was stirred at room temperature for 24 h before the solid was filtered. The filtrate was evaporated under vacuum, and the residue was recrystallized from EtOH to afford white crystals (4.33 g, 68%); mp 118-120 °C (lit.,8 mp 116-118 °C); 1H NMR (300 MHz, CDCl3) δ 7.43 (d, J = 8.2 Hz, 4H), 7.00 (d, J = 8.2 Hz, 4H), 3.66 (s, 4H).
b) Preparation of 1,3-di(4-bromophenyl)-2-propanone
It is prepared by essentially the same procedure as described for the preparation of 2c using DCC (15.4 g, 74.6 mmol) and DMAP (2.29 g, 18.7 mmol) in CH2Cl2 (150 ml), and 2-bromophenylacetic acid (16.1g, 74.9 mmol) in CH2Cl2 (150 ml) to afford 2c as white crystals (68%); mp 125-127 °C; 1H NMR (300 MHz, CDCl3) δ 7.57 (d, J = 7.8 Hz, 2H), 7.35- 6.95 (m, 6H), 3.95 (s, 4H).
2) Synthesis of indanocyclone 3a-3c
a) 1,3-Diphenylindeno[a]cyclopenta-2,8-dione (indanocyclone 3a) was synthesized by literature method.8 mp 204-206 °C (lit.,8a mp 204-206 °C), 1H NMR (CDCl3, 500 MHz) δ 8.61 (dd, J = 7.6 Hz, J = 1.0 Hz, 1H), 8.23-8.19 (m, 1H), 8.08-8.0 7(m, 1H), 7.76-7.73 (m, 4H), 7.52-7.48 (m, 6H).
b) 1,3-Di(4-bromphenyl)indeno[a]cyclopenta-2,8-dione (3b)
To a solution of ninhydrin (2.86 g, 14.65 mmol) and 1,3-di(4-bromophenyl)-2-propanone (4.996 g, 13.65 mmol) in hot EtOH (20 mL) was added slowly a solution of KOH in MeOH (10%, 3.5 mL). After addition, the mixture was stirred at 75 °C for 3 h with the color changed from yellow to deep brown. The solid was filtered and crystallized from EtOH to afford deep brown crystals (5.45 g, 81.5%), Rf = 0.59 (PE : DCM = 1 : 1), mp 292-294 °C; 1H NMR (300 MHz, CDCl3, ppm) δ: 8.59-8.56 (m, 2H), 8.19-8.11 (m, 2H), 7.81-7.80 (m, 2H), 7.71-7.60 (m, 6H).
c) 1,3-Di(2-bromphenyl)-indeno[a]cyclopenta-2,8-dione (3c)
3c is prepared essentially the same as 3b by employing ninhydrin (2.86 g, 14.65 mmol) and 1,3-di(2-bromophenyl)-2-propanone (4.996 g, 13.65 mmol) in EtOH (20 mL) and KOH in MeOH (10%, 3.5 mL) to afford deep red crystals (5.35 g, 80%); Rf = 0.57 (PE : DCM = 1 : 1), mp 195-197 °C. 1H NMR (300 MHz, CDCl3, ppm) δ 8.06-8.03 (m, 1H), 7.77-7.71 (m, 5H), 7.51-7.40 (m, 4H), 7.37-7.29 (m, 2H). 13C NMR (100 MHz, CDCl3) δ 197.03, 184.65, 150.44, 146.27, 143.58, 138.57, 135.68, 133.69, 133.42, 132.19, 131.94, 131.81, 131.13, 130.70, 130.40, 127.69, 127.49, 127.15, 124.93, 124.05, 123.73, 121.20.
3) The retro-Diels-Alder reaction between 3a-3c and norbornadiene to 4a-4c
a) Synthesis of 1,4-diphenylfluorenone (4a)
A 50 mL RB flask was added 3a (1.0 g, 2.994 mmol), norbornadiene (0.275 g, 2.994 mmol) and toluene (20 mL). The mixture was refluxed for 12 h and the volatiles were removed under vacuum and the crude product was purified by column chromatography (PE : DCM = 2 : 1) to afford a pale-yellow solid (1.0 g, 70%); Rf = 0.64 (PE : DCM = 1 : 1), mp 127-129 °C. 1H NMR (300 MHz, CDCl3, ppm) δ 7.12-7.03 (m, 10H), 6.94-6.91 (m, 1H), 6.82-6.75 (m, 4H), 6.27 (d, J = 6 Hz, 1H). 13C NMR (100 MHz, CDCl3, ppm) δ 192.98, 143.60, 142.06, 141.27, 139.58, 137.58, 137.26, 136.23, 134.60, 134.17, 131.33, 130.15, 129.22, 128.93, 128.86, 128.80, 128.24, 128.22, 127.93, 123.96. MS (ESI): m/z = 355.1092 [M+Na]+, C25H16NaO required: 355.1093.
b) 1,4-Di(4-bromophenyl)fluorenone (4b)
4b is prepared essentially the same as 4a by employing 3b (1.0 g, 2.0408 mmol), norbornadiene (0.1878 g, 2.0408 mmol) and toluene (20 mL) and purified by column chromatography (PE : DCM = 2 : 1) to afford a pale-yellow solid (0.667 g, 67%); Rf = 0.65 (PE : DCM = 1 : 1), mp 198-200 °C. 1H NMR (300 MHz, CDCl3, ppm) δ 7.80 (d, J = 8.0 Hz, 1H), 7.71 (d, J = 8.0 Hz, 1H), 7.57-7.47 (m, 3H), 7.44-7.37 (m, 3H), 7.35-7.26 (m, 2H), 7.22-7.18 (m, 3H), 6.41(d, J = 5.3 Hz, 1H). 13C NMR (100 MHz, CDCl3, ppm) δ 192.67, 143.19, 142.13, 140.21, 138.31, 136.21, 136.18, 136.13, 134.41, 134.38, 132.10, 131.11, 130.84, 130.61, 130.14, 129.12, 124.18, 123.04, 122.66, 122.58.
c) 1,4-Di(2-bromophenyl)fluorenone (4c)
4c is prepared essentially the same as 4a by employing 3c (1.0 g, 2.0408 mmol), norbornadiene (0.1878 g, 2.0408 mmol) and xylene (20 mL) and purified by column chromatography (PE : DCM = 2 : 1) to afford a pale-yellow solid (0.60 g, 60%); Rf = 0.64 (PE : DCM = 1 : 1); mp 235-237 °C. 1H NMR (300 MHz, CDCl3, ppm) δ 7.80 (d, J = 8.0 Hz, 1H), 7.71 (d, J = 8.0 Hz, 1H), 7.57-7.55 (m, 1H), 7.50-7.35 (m, 5H), 7.35-7.26 (m, 3H), 7.22-7.18 (m, 2H), 6.41(d, J = 5.3 Hz, 1H). 13C NMR (75 MHz, CDCl3) δ 192.62, 143.82, 142.20, 140.31, 140.25, 139.67, 139.23, 139.14, 136.28, 135.76, 134.66, 134.53, 133.39, 133.33, 132.77, 132.71, 131.18, 131.13, 130.91, 130.78, 130.49, 130.23, 129.65, 129.15, 128.14, 128.09, 127.28, 127.23, 124.22, 123.84, 123.74, 123.20, 123.11, 122.73.
4) Syntheses of 4d-4e by Suzuki cross-coupling reaction
a) Synthesis of 1,4-Di[4-(2-thienyl)phenyl]fluorenone (4d)
A 50ml RB flask was charged with 1,4-di(4-bromophenyl)fluornone 4b (0.151 g, 0.31 mmol), 2-thienylboronic acid (0.0947 g, 0.74 mmol), Pd(PPh3)4 (22 mg, 0.031 mmol), toluene (7 mL), EtOH (7 mL) and aq. Na2CO3 (0.1 g, 0.93 mmol) in water (3 mL), and the mixture was refluxed for 8 h before it was poured into water and extracted with EtOAc, and the organic phase was dried over MgSO4. After workup, the residue was purified by column chromatography (PE : DCM = 2 : 1) to afford a yellow solid (0.125 g, 81%); Rf = 0.64 (PE : DCM = 1 : 1); mp 233-235 °C. 1H NMR (300 MHz, CDCl3, ppm) δ 7.78 (d, J = 8.5 Hz, 2H), 7.72 (d, J = 8.5 Hz, 2H), 7.61-7.56 (m, 3 H), 7.52-7.49 (m, 2H), 7.46-7.45 (m, 1H), 7.40-7.34 (m, 3H), 7.32-7.30 (m, 1H), 7.27-7.24 (m, 1H), 7.22-7.19 (m, 2H), 7.16-7.10 (m, 2H), 6.88-6.85 (m, 1H). 13C NMR (100 MHz, CDCl3, ppm) δ 192.96, 143.72, 143.49, 140.76, 138.56, 137.30, 137.24, 136.77, 136.77, 136.55, 136.26, 134.55, 134.31, 134.27, 131.22, 129.81, 129.51, 128.90, 128.28, 128.10, 126.18, 125.44, 125.32, 125.03, 124.05, 123.53, 123.38, 123.19. MS(ESI): m/z = 519.0857 [M+Na]+, C33H20NaOS2 required 519.0848.
b) Synthesis of 1,4-Di[2-(2-thienyl)phenyl]fluorenone (4e)
4e is prepared essentially the same as 4d by employing 4c (0.15 g, 0.31 mmol), 2-thienylboronic acid (0.095 g, 0.74 mmol), Pd (PPh3)4 (22 mg, 0.031 mmol), THF (15 mL) and aq. Na2CO3 (0.1 g, 0.93 mmol) in water (3 mL) and purified by column chromatography (PE : DCM = 2 : 1) to afford a yellow solid (0.116 g, 76%), Rf = 0.63 (PE : DCM = 1 : 1), mp 132-134 °C. 1H NMR (300 MHz, CDCl3, ppm) δ 7.73 (d, J = 7.9 Hz, 1H), 7.63 (d, J = 7.1 Hz, 1H), 7.56-7.35 (m, 7H), 7.19-7.01 (m, 5H), 7.03-7.01 (m, 1H), 6.84-6.77 (m, 4H), 6.47-6.42 (m, 1H). 13C NMR (100 MHz, CDCl3) δ 192.60, 143.58, 142.87, 142.68, 141.93, 140.18, 137.29, 136.58, 136.14, 134.27, 133.66, 133.42, 131.80, 131.31, 130.74, 130.44, 130.14, 130.07, 129.90, 128.84, 128.71, 128.32, 128.00, 127.22, 126.99, 126.93, 126.85, 126.81, 126.73, 125.88, 125.35, 123.78, 122.67. MS (ESI): m/z = 519.0836 [M+Na]+, C33H20NaOS2 required 519.0848.
5) Reduction of 4a-4e to 5a-5e by Na2S∙9H2O
a) 1,4-Diphenyfluorene (5a)
To a 100 mL RB flask was added Na2S∙9H2O (1.056 g, 4.4 mmol), N-methyl-2-pyrrolidone (NMP, 60 mL), the mixture was stirred at RT for 15 min, followed by adding 1,4-diphenylfluorenone (0.666 g, 2 mmol) and the mixture was refluxed for 12 h under N2 atmosphere before it was poured into saturated NH4Cl (100 mL) and the solid was filtered and purified by column chromatography with petroleum ether (PE) to afford a white solid (0.46 g, 72%), Rf = 0.59 (PE), mp 83-85 °C. 1H NMR (300 MHz, CDCl3, ppm) δ 7.59 (d, J = 7.5 Hz, 2H), 7.55-7.37 (m, 9H), 7.35-7.29 (m, 2H), 7.22-7.17 (m, 1H), 7.05 (t, J = 7.4 Hz, 1H), 6.97 (d, J = 7.4 Hz, 1H), 3.99 (s, 2H). 13C NMR (100 MHz, CDCl3) δ 143.76, 141.63, 141.45, 141.18, 141.06, 139.03, 138.07, 136.96, 129.45, 129.23, 128.53, 127.53, 127.29, 126.90, 126.48, 126.21, 124.61, 122.98, 36.93.
b) 1,4-Di(4-bromophenyl)fluorene (5b)
5b is prepared essentially the same as 5a with Na2S∙9H2O (1.056 g, 4.4mmol), NMP (60 mL) and 4b (0.976 g, 2 mmol), which was purified by column chromatography with PE to afford a white solid (0.28 g, 29.5%), Rf = 0.52 (PE), mp 80-82 °C. 1H NMR (300 MHz, CDCl3, ppm) δ 7.65-7.62 (m, 2H), 7.56-7.45 (m, 8H), 7.40-7.34 (m, 1H), 7.29-7.22 (m, 1H), 7.09 (t, J = 7.4 Hz, 1H), 7.01 (d, J = 7.5 Hz, 1H), 4.04 (s, 2H); 13C NMR (100 MHz, CDCl3, ppm) δ 142.71, 140.59, 140.41, 140.14, 140.02, 137.99, 137.03, 135.91, 128.41, 128.19, 127.63, 127.48, 126.48, 126.24, 125.85, 125.44, 125.17, 123.57, 121.94, 35.89.
c) 1,4-Di(2-bromophenyl)fluorene (5c)
5c is prepared essentially the same as 5a with Na2S∙9H2O (1.056 g, 4.4 mmol), NMP (60 mL) and 4c (0.976 g, 2 mmol), which was purified by column chromatography with PE to afford a white solid (0.246 g, 26.0%), Rf = 0.51 (PE), mp 76-78 °C. 1H NMR (300 MHz, CDCl3, ppm) δ 7.65-7.55 (m, 2H), 7.55-7.45 (m, 6H), 7.43-7.41 (m, 1H), 7.36-7.29 (m, 2H), 7.23-7.18 (m, 1H), 7.05 (t, J = 7.4 Hz, 1H), 6.97 (d, J = 7.4 Hz, 1H), 4.00 (s, 2H). 13C NMR (100 MHz, CDCl3) δ 143.78, 141.66, 141.48, 141.21, 141.09, 139.06, 138.10, 136.99, 129.48, 129.26, 128.70, 128.55, 127.55, 127.31, 126.93, 126.51, 126.24, 124.64, 123.01, 36.96.
d) 1,4-Di[4-(2-thienyl)phenyl]fluorene (5d)
5d is prepared essentially the same as 5a with Na2S∙9H2O (0.106 g, 0.44 mmol), NMP (6 mL), and 4d (0.099 g, 0.2 mmol) and purified by column chromatography with PE to afford a white solid (0.070 g, 73%), Rf = 0.18 (PE), mp 231-233 °C. 1H NMR (300 MHz, CDCl3, ppm) δ 7.76 (t, J = 7.7 Hz, 4H), 7.62 (d, J = 7.8 Hz, 2H), 7.55 (d, J = 7.8 Hz, 2H), 7.50 (d, J = 7.7 Hz, 1H), 7.45-7.44 (m, 1H), 7.41-7.39 (m, 1H), 7.37-7.29 (m, 4H), 7.22-7.20 (m, 1H), 7.17-7.07 (m, 4H), 4.04 (s, 2H). 13C NMR (100 MHz, CDCl3) δ 144.18, 144.10, 143.72, 141.71, 141.31, 140.27, 140.07, 139.16, 137.58, 136.49, 133.67, 133.50, 129.80, 129.54, 129.17, 128.16, 126.80, 126.63, 126.34, 126.07, 125.97, 124.98, 124.96, 124.68, 123.26, 123.24, 123.07, 37.01. MS (ESI): m/z = 505.1106 [M+Na]+, C33H22NaS2 required 505.1055.
e) 1,4-Di[2-(2-thienyl)phenyl]fluorene (5e)
5e is prepared essentially the same as 5a with Na2S∙9H2O (0.106 g, 0.44 mmol), NMP (6 mL), and 4e (0.099 g, 0.20 mmol) and purified by column chromatography with PE to afford a white solid (0.061 g, 63%), Rf = 0.25 (PE), mp 68-70 °C. 1H NMR (300 MHz, CDCl3) δ 7.74 (d, J = 7.7 Hz, 1H), 7.68 (d, J = 7.4 Hz, 1H), 7.54-7.47 (m, 1H), 7.43-7.38 (m, 5H), 7.32 (d, J = 7.5 Hz, 1H), 7.17-7.05 (m, 5H), 6.98 (t, J = 7.5 Hz, 1H), 6.67-6.75 (m, 4H), 6.68 (d, J = 7.7 Hz, 1H), 3.58 (s, 1H), 3.43 (s, 1H). 13C NMR (75 MHz, CDCl3) δ 143.73, 142.77, 142.56, 141.48, 139.64, 138.99, 138.92, 137.78, 135.75, 133.68, 133.27, 130.92, 130.77, 129.79, 129.74, 129.26, 128.14, 127.95, 127.85, 127.63, 127.40, 126.89, 126.70, 126.54, 126.31, 126.27, 125.43, 125.32, 124.50, 122.50, 36.36. MS (ESI): m/z = 505.1066 [M+Na]+, C33H22NaS2 required 505.1055.
ACKNOWLEDGEMENTS
We thank the large instruments open foundation of East China Normal University (2013-1) for instrument fund.
References
1. (a) M. Bendikov, F. Wudl, and D. F. Perepichka, Chem. Rev., 2004, 104, 4891; CrossRef (b) A. R. Murphy and J. M. J. Frechet, Chem. Rev., 2007, 107, 1066; CrossRef (c) Y.-J. Cheng, S.-H. Yang, and C.-S. Hsu, Chem. Rev., 2009, 109, 5868; CrossRef (d) J. E. Anthony, Chem. Rev., 2006, 106, 5028; CrossRef (e) H.Yamada, T. Okujima, and N. Ono, Chem. Commun., 2008, 2957; CrossRef (f) K. Takimiya, Y. Kunugi, and T. Otsubo, Chem. Lett., 2007, 36, 578; CrossRef (g) W. Wu, Y. Liu, and D. Zhu, Chem. Soc. Rev., 2010, 39, 1489; CrossRef (h) A. Pron, P. Gawrys, M. Zagorska, D. Djurado, and R. Demadrille, Chem. Sov. Rev., 2010, 39, 2577; CrossRef (i) M. Mas-Torrent and C. Rovira, Chem. Soc. Rev., 2008, 37, 827; CrossRef (j) J. E. Anthony, Angew. Chem. Int. Ed., 2008, 47, 452; CrossRef (k) N. Blouin and M. Leclerc, Acc. Chem. Res., 2008, 41, 1110. CrossRef
2. (a) K. Iida, Jpn. Kokai Tokkyo Koho 2010, JP2010239134 A; (b) K. Iida, H. Kusaka, K. Endo, Y. Li, and K. Okabe, PCT Int. Appl. 2010, WO2010018813 A1; (c) H. N. Cho, Y. C. Kim, J.-M. Hong, J.-B. Kim, D. K. Moon, Y. S. Park, and H. S. Nam, U. S. Pat. Appl.publ. 2002, US20020051895 A1.
3. Z. Jiang, H. Yao, Z. Zhang, C. Yang, Z. Liu, Y. Tao, J. Qin, and D. Ma, Org. Lett., 2009, 11, 2607. CrossRef
4. (a) L. H. Xie, X. Y. Hou, Y. R. Hua, C. Tang, F. Liu, Q. L. Fan, and W. Huang, Org. Lett., 2006, 8, 3701; CrossRef (b) T. Kowada, Y. Matsuyama, and K. Ohe, Synlett, 2008, 1902. CrossRef
5. T. K. Bandyopadhyay and A. J. Bhattacharya, Ind. J. Chem., 1981, B20, 91.
6. (a) L. Rong, Q. Liu, J. Tang, and Z. Chi, Heterocycles, 2010, 81, 977; CrossRef (b) G. Wu, Q. Liu, and J. Tang, Heterocycles, 2010, 81, 1157; CrossRef (c) L. Rong, Q. Liu, Y. Shi, and J. Tang, Chem. Comm., 2011, 47, 2155. CrossRef
7. (a) W. Yao, Q. Liu, Y. Shi, and J. Tang, Heterocycles, 2012, 85, 1077; CrossRef (b) Y. Shi, Q. Liu, G. Wu, L. Rong, and J. Tang, Tetrahedron, 2011, 67, 1201; CrossRef (c) W. Ried and D. Freitag, Chem. Ber., 1966, 90, 2675; CrossRef (d) W. Ried and D. Freitag, Chem. Ber., 1968, 101, 756; CrossRef (e) W. Ried and M. Ritz, Liebigs Ann. Chem., 1969, 724, 122.. CrossRef
8. H. Sauriat-Dorizon, T. Maris, J. D. Wuest, and G. D. Enright, J. Org. Chem., 2003, 68, 240. CrossRef
9. X. Xiong, Q. Liu, J. Zhang, M. Zhu, Y. Wang, and S. Deng, RSC Adv., 2013, 3, 17707. CrossRef
10. T. Takido, Y. Yamane, and K. Itabashi, J. Synth. Org. Chem. Jpn., 1975, 33, 694. CrossRef