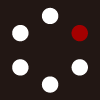
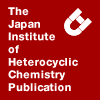
HETEROCYCLES
An International Journal for Reviews and Communications in Heterocyclic ChemistryWeb Edition ISSN: 1881-0942
Published online by The Japan Institute of Heterocyclic Chemistry
e-Journal
Full Text HTML
Received, 19th December, 2014, Accepted, 20th January, 2015, Published online, 27th January, 2015.
DOI: 10.3987/COM-14-13157
■ One-Carbon Homologation of Pyrrole Carboxaldehyde via Wittig Reaction and Mild Hydrolysis of Vinyl Ether – toward the Synthesis of a Sterically Locked Phytochrome Chromophore
Ryo Sakata, Takahiro Soeta, and Yutaka Ukaji*
Division of Material Sciences, Graduate School of Natural Science and Technology, Kanazawa University, Kakuma, Kanazawa, Ishikawa 920-1192, Japan
Abstract
Mild hydrolysis of vinyl ether derived from a pyrrole carboxaldehyde corresponding to the B,C-ring component of phytochromobilin chromophore was achieved by treatment with oxalyl chloride in chloroform in the presence of water and ethanol to afford a one-carbon homologated aldehyde. This aldehyde was applied to the synthesis of the sterically locked 15E-anti CD-ring component of the chromophore. Furthermore, the aldehyde could be converted to the intermediate for the sterically locked 5Z-anti AB-ring component.Phytochromes are chromoproteins that have a linear tetrapyrrole chromophore, which is covalently bound to the protein and responds to red and far-red light through a reversible interchange between the red light-absorbing (Pr) and the far-red-light-absorbing (Pfr) forms. Land plants use phytochromobilin (PΦB), cyanobacteria use phycocyanobilin (PCB), and other bacteria use biliverdin (BV) as chromophores.
Phytochromes play critical roles in various light-regulated processes through photoconversion; the first step in the photoconversion from Pr to Pfr is thought to be a Z-to-E isomerization around the C15–C16 double bond between the C- and D-rings of the chromophores (Figure 1).1 To determine the stereochemistries of the Pr- and Pfr-forms of the chromophore, syntheses of the sterically locked tetrapyrrole chromophores were examined.2,3 Previously, the sterically locked PCB derivative with an 8-membered 15E-anti CD-ring component was synthesized and used in biological investigations, which induced branch making without light.3e,f However, the influence of the ring size of the sterically locked 15E-anti CD ring component on biologically activity remained unclear. For the synthesis of the sterically locked chromophore, oxidative functionalization of the common pyrrole and pyrromethenone was investigated.4 Recently, DDQ oxidation of the B,C-ring component of phytochromobilin chromophore 1 in the presence of MeOH was found to afford the corresponding pyrrole carboxaldehyde 2.4a Based on this oxidative transformation, the sterically locked 7-membered 15E-anti CD-ring component could be synthesized via a convergent strategy.4b,5 In contrast, the previous method for the synthesis of the 8-membered CD-ring component of 15E-anti PCB derivatives required many reaction steps in the linear strategy that was adopted.3e,5 To synthesize the 8-membered 15E-anti CD-ring component more efficiently, the new oxidation strategy could be applied. The present report describes the one-carbon homologation of the pyrrole carboxaldehyde 2 by a Wittig reaction, followed by mild hydrolysis of the
vinyl ether obtained, and its application to the synthesis of sterically locked 8-membered 15E-anti CD ring component 5 (Scheme 1). In addition, simple conversion of the homologated aldehyde 3 to the corresponding alcohol 6, which is the synthetic intermediate for the synthesis of sterically locked 5Z-anti AB-ring component 7,4b was also demonstrated.
First, one-carbon homologation was performed via a Wittig reaction using Ph3P=CH(OMe) starting from t-butyl 3-(3-(allyloxy)-3-oxopropyl)-4-formyl-1H-pyrrole-2-carboxylate (2), which was readily obtained by DDQ oxidation of 1,4a and the desired vinyl ether 8 was obtained in 75% yield as a mixture of E- and Z-isomers (E/Z = 1/2).
Next, hydrolysis of the vinyl ether 8 was examined. Hydrolysis of methyl vinyl ethers derived from pyrrole or indole carboxaldehydes under aqueous acidic conditions gave the corresponding aldehydes in good yields.6 However, the hydrolysis of 8 under acidic conditions, such as the presence of hydrochloric acid or p-toluenesulfonic acid, afforded complicated mixtures of the compounds.7 Although conversion to the aldehyde via the corresponding dimethyl acetal also was attempted,6c the dimethyl acetal was obtained in less than 20% yield after treatment with a catalytic amount p-toluenesulfonic acid in MeOH/THF. Nucleophilic cleavage by trimethylsilyl chloride/sodium iodide6e also failed to give a complicated mixture. During a survey of the conditions for hydrolysis, the vinyl ether 8 was shown to convert gradually to the desired aldehyde 3 over 140 h by monitoring of 1H NMR spectra of the isolated vinyl ether 8 in chloroform-d. This observation can be explained as follows. Chloroform-d slightly decomposed in the presence of oxygen under light to produce deuterium chloride,8 which caused hydrolysis with irrupting moisture. Based on this observation, the hydrolysis of the vinyl ether 8 in chloroform was examined. In the presence of 1 M hydrochloric acid or p-toluenesulfonic acid, hydrolysis proceeded; however the reactions were not clean enough. After several examinations, treatment with oxalyl chloride in the presence of water and ethanol in chloroform9 resulted in clean and quick hydrolysis to give the aldehyde 3 in 83% yield.10 These conditions were generally applicable to the aromatic and aliphatic vinyl ethers, as shown in Table 1.
Next, aldehyde 3 was used for the synthesis of the 15E-anti CD ring component (Scheme 3). The coupling with the D-ring moiety was performed via a Wittig reaction. The D-ring precursor 11 was converted into the phosphonium intermediate via palladium activation, and was further converted to the corresponding ylide 12 by treatment with butyllithium; coupling with the aldehyde 3 afforded 13 as a mixture of E- and Z-isomers.4b,11,12 The resulting olefinic moiety was reduced by hydrogenation to afford the product 4 in 20% yield from 3. Treatment of 4 with trifluoroacetic acid induced decarboxylation, and successive reaction with trimethyl orthoformate produced α-diformylated compound 14. Finally, the dialdehyde 14 was cyclized in the presence of DBU to afford the 15E-anti locked CD-ring component 5 with an 8-membered ring in 36% yield from 4 and uncyclized 14 in 21% yield. The obtained 14 was again treated with DBU to afford another 5 in 16% yield from 4. Although chemical yields were not good enough, the synthesis of the sterically locked 8-membered 15E-anti CD-ring component could be achieved via the convergent strategy in rather short steps than via linear route.3e
The one-carbon homologated aldehyde 3 also had the potential to be a useful intermediate for the synthesis of a sterically locked 5Z-anti AB-ring component. Reduction of 3 with sodium borohydride readily furnished the corresponding alcohol 6 (Scheme 4), which was already transformed into the 5Z-anti AB ring component 7 via the corresponding aldehyde 15.3a,4b Previously, the B-ring alcohol 6 needed to be synthesized through additional Barton pyrrole formation.3a Using the present method, intermediate 6 can be prepared simply from the common B,C-ring precursor 1 in 4 steps.
As described above, the one-carbon homologation of pyrrole carboxaldehyde, which could be readily produced by regioselective DDQ oxidation of the B,C-ring common pyrrole, was achieved by a Wittig reaction followed by mild hydrolysis of the resulting vinyl ether using oxalyl chloride in the presence of water and ethanol. This hydrolysis method could be generalized for aromatic and aliphatic vinyl ethers. Furthermore, the homologated aldehyde obtained was applied to the synthesis of sterically locked 15E-anti CD ring and 5Z-anti AB ring components. The present strategy provides an efficient route for the synthesis of sterically locked tetrapyrrole chromophores starting from a common pyrrole compound.
EXPERIMENTAL
1H NMR spectroscopy was performed in CDCl3 using a JEOL ECS 400 NMR (400 MHz) spectrometer. Chemical shifts (δ) were determined relative to TMS (δ = 0 ppm) as an internal standard. 13C NMR spectroscopy was performed in CDCl3 on a JEOL ECS 400 NMR (100 MHz) spectrometer and chemical shifts (δ) were determined relative to CDCl3 (δ = 77.0 ppm) as an internal standard. IR spectra were acquired on a JASCO FT/IR-230 spectrometer. Mass spectra were obtained using JMS-700, JMS-T100TD, and Bruker microtof II mass spectrometers. Melting point was determined on a micro-melting apparatus (Yanagimoto–Seisakusho) and was uncorrected. Merck silica gel 60 PF254 (Art. 7749) and Cica silica gel 60N spherical neutral (37563-84) were used for thin-layer chromatography (TLC) and flash column chromatography, respectively.
tert-Butyl 3-(3-(allyloxy)-3-oxopropyl)-4-formyl-1H-pyrrole-2-carboxylate (2): To the solution of 1 (0.88 g, 3.0 mmol) and MeOH (1.2 mL, 30 mmol) in toluene (12 mL), DDQ (1.4 g, 6.2 mmol) was added portionwise and the mixture was stirred at rt for 48 h. The reaction mixture was filtered through a bed of Celite. The filtrate was diluted with AcOEt and water and the aqueous layer was extracted with AcOEt. The combined extracts were washed with sat. aqueous solution of NaHSO3, sat. aqueous solution of NaHCO3, and brine, dried over Na2SO4 and condensed under reduced pressure. The residue was purified by column chromatography (SiO2, hexane/AcOEt = 2/1, v/v) to give the corresponding aldehyde 2 (0.72 g, 78% yield) as a solid: mp 95–96 ºC (AcOEt/hexane); 1H NMR (CDCl3, 400 MHz): δ = 1.55 (s, 9H), 2.61 (d, 2H, J = 7.9 Hz), 3.36 (d, 2H, J = 7.9 Hz), 4.56 (d, 2H, J = 5.9 Hz), 5.20 (dd, 1H, J = 10.5, 1.5 Hz), 5.28 (dd, 1H, J = 17.2, 1.5 Hz), 5.90 (ddt, 1H, J = 17.2, 10.5, 5.9 Hz), 7.43 (d, 1H, J = 3.7 Hz), 9.91 (s, 1H), 10.0 (br, 1H); 13C NMR (CDCl3, 100 MHz): δ = 20.4, 28.3, 35.0, 65.0, 82.5, 118.1, 123.1, 125.6, 129.1, 129.8, 132.2, 160.9, 172.4, 186.0; IR (KBr) 3267, 3009, 2981, 2931, 2881, 1742, 1700, 1664, 1559, 1418, 1393, 1296, 1141, 1052 cm–1; Anal. Calcd (%) for C16H21NO5: C, 62.53; H, 6.89; N, 4.56. Found: C, 62.29; H, 6.86; N, 4.48.
tert-Butyl 3-(3-(allyloxy)-3-oxopropyl)-4-(2-methoxyvinyl)-1H-pyrrole-2-carboxylate (8): To a suspension of (methoxymethyl)triphenylphosphonium chloride (3.42 g, 10.0 mmol) in THF (30 mL), nBuLi (3.28 mL of 2.65 M solution in hexane, 8.7 mmol) was added dropwise at 0 ºC and the mixture was stirred for 1 h at 0 ºC. Aldehyde 2 (431 mg, 1.4 mmol) in THF (5 mL) was added to the resulting solution and the mixture was stirred at 0 ºC for 10 min. The reaction was quenched by the addition of sat. aqueous solution of NH4Cl and the mixture was subsequently extracted with AcOEt. The combined extracts were washed with water and brine, dried over Na2SO4 and condensed under reduced pressure. The residue was purified by column chromatography (SiO2, hexane/AcOEt = 6/1 to 3/1, v/v) to give E- and Z-mixture of the vinyl ether (354 mg, 75% yield, E/Z = 1/2) as an oil: 1H NMR (CDCl3, 400 MHz): (E) δ = 1.56 (s, 9H), 2.58 (t, 2H, J = 8.3 Hz), 3.06 (t, 2H, J = 8.3 Hz), 3.65 (s, 3H), 4.58 (d, 2H, J = 5.5 Hz), 5.22 (d, 1H, J = 10.6 Hz), 5.25 (d, 1H, J = 17.3 Hz), 5.63 (d, 1H, J = 12.8 Hz), 5.92 (ddt, 1H, J = 17.3, 10.6, 5.5 Hz), 6.72 (d, 1H, J = 12.8 Hz), 6.76 (d, 1H, J = 2.8 Hz), 8.81 (br, 1H); (Z) δ = 1.56 (s, 9H), 2.55 (t, 2H, J = 8.3 Hz), 3.08 (t, 2H, J = 8.3 Hz), 3.75 (s, 3H), 4.58 (d, 2H, J = 5.5 Hz), 5.219 (d, 1H, J = 6.9 Hz), 5.224 (dd, 1H, J = 10.6 Hz), 5.30 (dd, 1H, J = 17.3 Hz), 5.92 (ddt, 1H, J = 17.3, 10.6, 5.5 Hz), 6.07 (d, 1H, J = 6.9 Hz), 7.32 (d, 1H, J = 3.2 Hz), 8.87 (br, 1H); 13C NMR (CDCl3, 100 MHz): (E) δ = 20.6, 28.4, 35.5, 56.3, 64.9, 81.0, 95.9, 116.9, 118.0, 120.5, 120.8, 126.6, 132.3, 147.5, 161.0, 172.8; (Z) δ = 20.3, 28.4, 35.5, 60.1, 64.9, 80.9, 96.6, 118.0, 119.1, 119.8, 121.9, 126.2, 132.3, 145.5, 161.1, 172.9; IR (neat) 3312, 2977, 2933, 1736, 1685, 1663, 1560, 1455, 1401, 1368, 1288, 1259, 1130, 1050, 987, 941, 781 cm–1; HRMS (DART): m/z calcd for C18H26NO5: 336.1811: [M+H]+; found: 336.1803.
General Procedure for Hydrolysis of Vinyl Ether 9 (Table 1): To a solution of vinyl ether 9 (0.5 mmol) in CHCl3 (4.5 mL),9 oxalyl chloride (63 mg, 0.5 mmol) in CHCl3 (0.5 mL)9 was added at rt. To this reaction mixture, ethanol (29 µL, 0.5 mmol) and H2O (9 µL, 0.5 mmol) were subsequently added and the whole was stirred at rt. After reaction was completed (monitored by TLC), the reaction was quenched by the addition of sat. aqueous solution of NaHCO3 and the mixture was subsequently extracted with CHCl3. The combined extracts were washed with brine, dried over Na2SO4, and condensed under reduced pressure. The residue was purified by column chromatography.
tert-Butyl 3-(3-(allyloxy)-3-oxopropyl)-4-(2-oxoethyl)-1H-pyrrole-2-carboxylate (3):13 To a solution of vinyl ether 8 (63 mg, 0.2 mmol) in CHCl3 (5.5 mL),9 oxalyl chloride (25 mg, 0.2 mmol) in CHCl3 (0.5 mL)9 was added at room temperature. To this reaction mixture, ethanol (11 µL, 0.2 mmol) and H2O (4 µL, 0.2 mmol) were subsequently added and the whole was stirred at rt for 0.5 h. The reaction was quenched by the addition of sat. aqueous solution of NaHCO3 and the mixture was subsequently extracted with CHCl3. The combined extracts were washed with brine, dried over Na2SO4, and condensed under reduced pressure. The residue was purified by column chromatography (SiO2, hexane/Et2O = 4/1 to 2/1, v/v) to give aldehyde 3 (53 mg, 83% yield) as an oil. 1H NMR (CDCl3, 400 MHz): δ = 1.58 (s, 9H), 2.59 (t, 2H, J = 7.8 Hz), 2.97 (t, 2H, J = 7.8 Hz), 3.59 (d, 2H, J = 1.8 Hz), 4.56 (d, 2H, J = 5.6 Hz), 5.22 (dd, 1H, J = 11.9, 1.5 Hz), 5.28 (dd, 1H, J = 15.9, 1.5 Hz), 5.89 (ddt, 1H, J = 15.9, 11.9, 5.6 Hz), 6.78 (d, 1H, J = 3.2 Hz), 9.41 (br, 1H), 9.68, (t, 1H, J = 1.8 Hz); 13C NMR (CDCl3, 100 MHz): δ = 20.3, 28.4, 35.2, 40.1, 65.0, 81.3, 114.6, 118.1, 120.9, 121.2, 128.2, 132.2, 160.7, 172.7, 199.4; IR (neat) 3312, 2977, 2935, 1727, 1685, 1508, 1456, 1409, 1368, 1289, 1172, 1132 cm–1; HRMS (DART): m/z calcd for C17H24NO5: 322.1655: [M+H]+; found: 322.1651.
tert-Butyl 4-(3-(4-ethyl-5-oxo-2,5-dihydro-1H-pyrrol-3-yl)propyl)-3-(3-methoxy-3-oxopropyl)-1H- pyrrole-2-carboxylate (4): To a mixture of 11 (49 mg, 0.27 mmol) and nBu3P (0.64 ml, 2.67 mmol) in THF (3 mL), [Pd(PPh3)4] (15.5 mg, 0.013 mmol) and NaBr (55 mg, 0.52 mmol) in MeOH (3 mL) was added. After refluxing overnight, the solution was cooled to –40 ºC followed by addition of nBuLi (0.17 ml of 1.6 M solution in hexane, 0.26 mmol). The reaction was gradually warmed to –10 ºC and aldehyde 3 (86 mg, 0.26 mmol) in THF (1 mL) was added to the reaction mixture. After stirring at rt overnight, the solvent was evaporated and the residue was diluted with AcOEt and water. The aqueous layer was extracted with AcOEt and the combined extracts were washed by sat. aqueous solution of NH4Cl and brine, then dried over Na2SO4. The solvent was condensed under reduced pressure and the residue was purified by chromatography (SiO2, hexane/AcOEt = 1/2, v/v) to give 13 (58 mg) containing inseparable byproducts as an oil. To a solution of the obtained 13 in MeOH (3 mL), 5% Pd/C (19 mg) was added and the mixture was stirred at rt under 1 atm of hydrogen for 6 h. The mixture was filtered through a bed of Celite and condensed under reduced pressure. The residue was purified by TLC (SiO2, hexane/acetone = 2/1, v/v) to give 4 (23 mg, 20% yield from 3). 1H NMR (CDCl3, 400 MHz): δ = 1.07 (t, 3H, J = 7.2 Hz), 1.57 (s, 9H), 1.67–1.75 (m, 2H), 2.27 (q, 2H, J = 7.2 Hz), 2.43 (t, 2H, J = 7.8 Hz), 2.48 (t, 2H, J = 7.8 Hz), 2.54 (t, 2H, J = 7.2 Hz), 2.99 (t, 2H, J = 7.2 Hz), 3.67 (s, 3H), 3.84 (s, 2H), 6.67 (d, 1H, J = 2.0 Hz), 6.99 (br, 1H) 9.20 (br, 1H); 13C NMR (CDCl3, 100 MHz): δ = 13.5, 16.7, 20.5, 24.8, 27.6, 28.4, 29.8, 35.3, 48.2, 51.5, 81.0, 119.2, 120.7, 124.0, 127.4, 134.1, 152.6, 161.0, 173.6; IR (neat) 3334, 2975, 2934, 2867, 1733, 1682, 1507, 1456, 1406, 1368, 1298, 1249, 1141, 1060, 1032 cm–1; HRMS (ESI-TOF)): m/z calcd for C22H32N2O5Na: 427.2209: [M+Na]+; found: 427.2203.
Methyl 3-(7-ethyl-2-formyl-8-oxo-1,4,5,6,8,9-hexahydrocycloocta[1,2-b:7,6-b']dipyrrol-3-yl)- propanoate (5): Compound 4 (37 mg, 0.092 mmol) was dissolved in CF3CO2H (0.46 mL, 6.0 mmol) and the reaction mixture was stirred at rt for 2 h. To the mixture, trimethyl orthoformate (0.46 mL, 4.2 mmol) was added and the reaction mixture was stirred for 2 h. After the solvent was evaporated, the residue was dissolved in THF (4.6 mL) followed by addition of DBU (56 mg, 0.37 mmol), and the reaction mixture was heated at 60 ºC overnight. After the reaction was cooled to rt, the solvent was evaporated and the residue was diluted with AcOEt and water. The aqueous layer was extracted with AcOEt. The combined extracts were washed with sat. aqueous solution of NH4Cl and brine, dried over Na2SO4 and condensed under reduced pressure. The residue was purified by TLC (SiO2, hexane/AcOEt = 1/1, v/v) to give the sterically locked CD-ring component 5 (11 mg, 36% yield from 4) and the diformyl compound 14 (7 mg, 21%). The obtained 14 was treated with DBU (12 mg, 0.076 mmol) in THF (1 mL) at 60 ºC overnight. After the solvent was removed, the residue was partitioned with AcOEt and water and extracted with AcOEt. The combined extracts were washed with sat. aqueous solution of NH4Cl and brine, dried over Na2SO4 and condensed under reduced pressure. The residue was purified by TLC (SiO2, hexane/AcOEt = 1/2, v/v) to give another 5 (5 mg, 16% yield from 4) as an oil. 1H NMR (CDCl3/CD3OD = 3/1, 400 MHz): δ = 1.11 (t, 3H, J = 7.6 Hz), 1.97 (quint, 2H, J = 6.5 Hz), 2.35–2.41 (m, 4H), 2.50 (t, 2H, J = 6.5 Hz), 2.61 (t, 2H, J = 7.8 Hz), 3.06 (t, 2H, J = 7.8 Hz), 3.68 (s, 3H), 6.19 (s, 1H), 9.10 (br, 1H), 9.58 (s, 1H), 10.77 (br, 1H); 13C NMR (CDCl3/CD3OD = 3/1, 100 MHz): δ = 13.3, 16.4, 19.0, 21.4, 22.8, 33.0, 36.1, 51.5, 100.7, 124, 3, 129.4, 133.1, 135.1, 136.7, 140.7, 142.7, 171.9, 173.0, 177.5; IR (KBr) 3237, 2932, 2858, 1738, 1683, 1621, 1557, 1455, 1373, 1260, 1219, 1157, 1050, 836, 756 cm–1; HRMS (ESI-TOF)): m/z calcd for C19H22N2O4Na: 365.1477: [M+Na]+; found: 365.1472.
tert-Butyl 3-(3-(allyloxy)-3-oxopropyl)-4-(2-hydroxyethyl)-1H-pyrrole-2-carboxylate (6):3a To the aldehyde 3 (32 mg, 0.1 mmol) in MeOH (4 mL), NaBH4 (3.8 mg, 0.1 mmol) was added at 0 ºC. After stirring for 10 min at rt, sat. aqueous solution of NaHCO3 was added, the mixture was extracted with CHCl3. The combined extracts were dried over Na2SO4 and condensed under reduced pressure. The residue was purified by column chromatography (SiO2, hexane/AcOEt = 1/1, v/v) to give the alcohol 6 (29 mg, 89% yield) as an oil. 1H NMR (CDCl3, 400 MHz): δ = 1.54 (s, 9H), 2.56 (t, 2H, J = 7.8 Hz), 2.69 (t, 2H, J = 6.6 Hz), 2.99 (t, 2H, J = 7.8 Hz), 3.73 (t, 2H, J = 6.6 Hz), 4.55 (d, 2H, J = 5.6 Hz), 5.19 (dd, 1H, J = 11.9, 1.5 Hz), 5.26 (dd, 1H, J = 15.9, 1.5 Hz), 5.88 (m, 1H), 6.71 (d, 1H, J = 3.2 Hz), 9.13 (br, 1H); 13C NMR (CDCl3, 100 MHz): δ = 20.4, 28.1, 28.4, 35.5, 63.0, 65.0, 81.0, 118.1, 120.1, 120.8, 120.9, 127.9, 132.2, 160.8, 172.9; IR (neat) 3346, 2978, 2935, 1738, 1714, 1407, 1223, 1172, 1133, 1051, 933 cm–1; HRMS (DART): m/z calcd for C17H26NO5: 324.1811: [M+H]+; found: 324.1819.
ACKNOWLEDGEMENTS
The present work was financially supported in part by the Mitani Foundation for Research and Development and a Grant-in-Aid for Challenging Exploratory Research from the Japan Society for the Promotion of Science.
References
1. M. A. Mroginski, D. H. Murgida, D. von Stetten, C. Kneip, F. Mark, and P. Hildebrandt, J. Am. Chem. Soc., 2004, 126, 16734; CrossRef T. Mizutani and S. Yagi, J. Porphyrins Phthalocyanines, 2004, 8, 226; CrossRef A. T. Ulijasz, G. Cornilescu, C. C. Cornilescu, J. Zhang, M. Rivera, J. L. Markley, and R. D. Vierstra, Nature, 2010, 463, 250; CrossRef Y. Yang, M. Linke, T. Haimberger, J. Hahn, R. Matute, L. González, P. Schmieder, and K. Heyne, J. Am. Chem. Soc., 2012, 134, 1408; CrossRef N. C. Rockwell, S. S. Martin, and J. C. Lagarias, Biochemistry, 2012, 51, 3576; CrossRef X. Zhuang, J. Wang, and Z. Lan, J. Phys. Chem. B, 2013, 117, 15976; CrossRef E. S. Burgie, T. Wang, A. N. Bussell, J. M. Walker, H. Li, and R. D. Vierstra, J. Biol. Chem., 2014, 289, 24573. CrossRef
2. K. Inomata, Bull. Chem. Soc. Jpn., 2008, 81, 25; CrossRef K. Inomata, Heterocycles, 2012, 85, 2879. CrossRef
3. M. A. S. Hammam, H. Nakamura, Y. Hirata, H. Khawn, Y. Murata, H. Kinoshita, and K. Inomata, Bull. Chem. Soc. Jpn., 2006, 79, 1561; CrossRef H. Khawn, L.-Y. Chen, H. Kinoshita, and K. Inomata, Chem. Lett., 2008, 37, 198; CrossRef L.-Y. Chen, H. Kinoshita, and K. Inomata, Chem. Lett., 2009, 38, 602; CrossRef K. Inomata, H. Khawn, L.-Y. Chen, H. Kinoshita, B. Zienicke, I. Molina, and T. Lamparter, Biochemistry, 2009, 48, 2817; CrossRef K. Nishiyama, A. Kamiya, M. A. S. Hammam, H. Kinoshita, S. Fujinami, Y. Ukaji, and K. Inomata, Bull. Chem. Soc. Jpn., 2010, 83, 1309; CrossRef R. Yang, K. Nishiyama, A. Kamiya, Y. Ukaji, K. Inomata, and T. Lamparter, Plant Cell, 2012, 24, 1936; CrossRef Y. Hirose, N. C. Rockwell, K. Nishiyama, R. Narikawa, Y. Ukaji, K. Inomata, J. C. Lagarias, and M. Ikeuchi, Proc. Natl. Acad. Sci. USA, 2013, 110, 4974. CrossRef
4. R. Iwamoto, Y. Ukaji, and K. Inomata, Chem. Lett., 2010, 39, 176; CrossRef L.-Y. Chen, R. Iwamoto, Y. Ukaji, and K. Inomata, Chem. Lett., 2011, 40, 632; CrossRef R. Sakata, R. Iwamoto, S. Fujinami, Y. Ukaji, and K. Inomata, Heterocycles, 2011, 82, 1157; K. Takahashi, R. Iwamoto, R. Sakata, T. Soeta, K. Inomata, and Y. Ukaji, Heterocycles, 2012, 86, 1031; CrossRef Y. Tanaka, R. Iwamoto, R. Sakata, T. Soeta, K. Endo, S. Fujinami, K. Inomata, and Y. Ukaji, Heterocycles, 2015, 90, 883. CrossRef
5. J. B. Hendrickson, J. Am. Chem. Soc., 1977, 99, 5439; CrossRef F. A. Carey and R. J. Sundberg, 'Advanced Organic Chemistry', 5th ed. Part B, Springer, New York, 2007, p.1165. The synthetic scheme of the previous synthesis of the 8-membered 15E-anti CD-ring component3e was recognized to be linear because the C-ring was constructed in later stage on the ω-nitroalkyl side chain of the firstly prepared D-ring moiety.
6. V. J. Demopoulos, J. Heterocycl. Chem., 1988, 25, 635; CrossRef D. J. Kempf and S. L. Condon, J. Org. Chem., 1990, 55, 1390; CrossRef J. D. Williams, J. J. Chen, J. C. Drach, and L. B. Townsend, J. Med. Chem., 2004, 47, 5753; CrossRef G. Gentile, R. D. Fabio, F. Pavone, F. M. Sabbatini, Y. St-Denis, M. G. Zampori, G. Vitulli, and A. Worby, Bioorg. Med. Chem. Lett., 2007, 17, 5218; CrossRef Z. Kosarych and T. Cohen, Tetrahedron Lett., 1980, 21, 3959; CrossRef D. S. Ermolat’ev, J. B. Bariwal, H. P. L. Steenackers, S. C. J. De Keersmaecker, and E. V. Van der Eycken, Angew. Chem. Int. Ed., 2010, 49, 9465; CrossRef S. Gemma, S. Kunjir, S. S. Coccone, M. Brindisi, V. Moretti, S. Brogi, E. Novellino, N. Basilico, S. Parapini, D. Taramelli, G. Campiani, and S. Butini, J. Med. Chem., 2011, 54, 5949. CrossRef
7. Based on the analyses of the byproducts by 1H NMR, one of the undesired pathways might be the electrophilic aromatic substitution of the pyrrole ring.
8. It was reported that chloroform undergoes decomposition to give hydrogen chloride and carbon oxides such as phosgene in the presence of oxygen under light; A. M. Clover, J. Am Chem. Soc., 1923, 45, 3133; CrossRef Y. Kuwahara, A. Zhang, H. Soma, and A. Tsuda, Org. Lett., 2012, 14, 3376. CrossRef
9. Dehydrated chloroform in the presence of amylene as a stabilizer (Wako 031-21935) without ethanol was used as a solvent. For general purpose of hydrolysis, chloroform in the presence of ethanol as a stabilizer could be used.
10. Ethanol was crucial for the quick and clean hydrolysis probably due to smooth generation of hydrogen chloride by the reaction with oxalyl chloride. In the absence of ethanol, the consumption of vinyl ether was rather slow.
11. Y. Tsukahara, H. Kinoshita, K. Inomata, and H. Kotake, Bull. Chem. Soc. Jpn., 1984, 57, 3013. CrossRef
12. At this stage, the allyl ester side chain of compound 3 was converted to methyl ester in the product 13 due to the basic conditions using methanol.
13. Diluted conditions were required for the hydrolysis of the vinyl ether 8. When the hydrolysis was performed according to the general procedure, deprotection of tert-butyl ester moiety also proceeded.