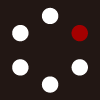
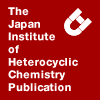
HETEROCYCLES
An International Journal for Reviews and Communications in Heterocyclic ChemistryWeb Edition ISSN: 1881-0942
Published online by The Japan Institute of Heterocyclic Chemistry
e-Journal
Full Text HTML
Received, 26th December, 2014, Accepted, 19th February, 2015, Published online, 3rd March, 2015.
DOI: 10.3987/COM-14-13164
■ An Cost-Effective and Safe Process of L-cis-4,5-Methanoproline Amide, the Key Synthetic Intermediate of Saxagliptin, via an Improved Simmons-Smith Reaction
Ding ding, Xianhua Pan, Wansheng Yu, Xiaojun Li, Suke Chen, and Feng Liu*
School of Perfume and Aroma Technology, Shanghai Institute of Technology, Haiquan Rd. 100 Shanghai, 201418, China
Abstract
L-cis-4,5-Methanoproline amide, a key intermediate of saxagliptin, was synthesized by an improved Simmons-Smith reaction. The zinc carbenoid was formed through Zn/CuBr and CH2I2, under the optimized condition, the title compound was gained with 68% yield and excellent diastereomeric selectivity (40:1 d.r.). The absence of the flammable and expensive ZnEt2 makes this procedure very attractive in large scale production.INTRODUCTION
Saxagliptin (Onglyza) 1 (Figure 1), a highly potent, orally available reversible dipeptidyl peptidase IV (DPP-IV) inhibitor, was jointly developed by Bristol-Myers Squibb Company and AstraZeneca Company, and recently approved by the US FDA for the treatment of type 2 diabetes mellitus.1,2 Compared with sitagliptin, the most widely prescribed DPP-IV inhibitor, saxagliptin shows more safety and less side effects.
Saxagliptin could be assembled by two segments: L-cis-4,5-methanoproline amide 2 and N-Boc-hydroxy- adamantylglycine 3.3,4 The general protocol of compound 2 in the literatures were synthesized from L-pyroglutamic acid, which have the core L-proline derivative structure (Scheme 1).4 L-Pyroglutamic acid was transferred to dihydropyrrole 4 by a 6 steps procedure, then the chiral five-three fused ring was prepared by a typical Simmons-Smith reaction, with Et2Zn and CH2I2.
But the harzard reaction condition, such as the use of flammable and expensive reagent (Et2Zn), and the very low reaction temperature, made the process cumbersome, uneconomical and difficult to handle on large scale. Herein, we wish to make some practical and efficient improvement of the Simmons-Smith reaction, on the base of the literatures’ procedure, to achieve an economical and easily scaled up process for the synthesis of L-cis-4,5-methanoproline amide 2.
RESULTS AND DISCUSSION
Following a reference procedure with modifications,4 dihydropyrrole 4 was prepared (Scheme 2). Thus, methyl esterfication and Boc-protection of L-pyroglutamic acid 5 gave 6. Reduction of 6 with LiEt3BH and treated with acetic anhydride and Na2CO3 formed L-2,3-dehydroproline ester 7. Hydrolysis of 7 with aq. LiOH in THF and then treated with MsCl and ammonia could transform the ester to carboxamide, to give dihydropyrrole carboxamide 4.
With the key intermediate 4 in hand, the improved stereoselective Simmons-Smith cyclopropanation was examined. The original Simmons-Smith reaction was usually carried out with copper-activated zinc (Zn/Cu couple) and diiodomethane (CH2I2), then the active intermediate IZnCH2I was formed, which could be used for the stereospecific conversion of alkenes to cyclopropanes, through a “butterfly-type” transition structure (Scheme 3).5
Due to the irreproducible of the in situ prepared Zn/Cu couple, lots of efforts were focus on the alternative of the zinc reagent, such as Sm/activator,6 R3Al,7 EtZnI,8 Et2Zn9 and Zn/copper halide.10 Among all the above Zn reagents, Zn/copper halide was most attractive, on account of the low cost, convenience and safety of the procedure. So, our improvement of the cyclopropanation was based on Zn/CuX, we then investigated the reaction with respect to the following four key variables: (1) halides, (2) the equivalent of the copper halides, (3) solvent, and (4) the reaction temperature (Table 1).
Firstly, Zinc dust and 0.1 equivalent of CuCl was mixed and warmed to reflux in Et2O under N2, CH2I2 was then added to form the zinc carbenoid, IZnCH2I, then the dihydropyrrole carboxamide 4 was reacted with this in-situ formed carbenoid at 20 °C, after work up, L-cis-4,5-methanoproline amide 2 was gained with 35% isolated yield and 30:1 dr (Table 1, entry 1). Different copper halides were tested (Table 1, entries 2-5) and we choose CuBr (Table 1, entry 2) to continue our optimization. We then scanned the solvents, it was found that ethers were more effective than alkyl halides (compared entry 2, 6, 8, 9 and entry 7), which revealed that the oxygen element of the non-polar solvent may partly participated the reaction. More CuBr loading could improve the reaction yield but the diastereomeric selectivity was lowered (Table 1, entry 10), but less CuBr was proved to be not satisfied (Table 1, entry 11). Then we tried to warm up the cyclopropanation temperature to enhance the yield, but this attempt was not successful, a lot of decompose products were obtained and the diastereomeric selectivity was also influenced (Table 1, entry 12). Lower temperatures could remain the diastereomeric ratio, but the solubility of the reaction mixture was decreased and then the yield was affected (Table 1, entry 13, 14). Finally, with the optimized condition, we got the title product 2 with the yield of 68% and excellent diastereomeric selectivity (40:1 dr), almost equal to the literature procedures.4
In conclusion, we have developed an improved Simmons-Smith reaction for the production of L-cis-4,5-methanoproline amide, a key intermediate of saxagliptin. In our protocol, Et2Zn was successfully replaced by a cheaper and safety Zn/CuBr mixture, which makes this procedure practical and economical attractive.
EXPERIMENTAL
Melting points were determined with a SGW X-4 micro melting point apparatus. 1H NMR spectra were recorded using an Avance 500 MHz spectrometer. All chemical shifts were reported in δ units relative to tetramethylsilane. ESI-MS were recorded on a Waters Quaptro Premier XE Mass Spectrometer. High resolution mass spectra were recorded on a Brucker Solaril X70 mass spectrometer. HPLC were determined on Dionex Ultimate 3000. Optical rotations were obtained on a Perkin-Elmer 241 Autopol polarimeter.
Starting Materials. All chemicals used in this study were commercially available.
(S)-1-tert-Butyl 2-methyl 5-oxopyrrolidine-1,2-dicarboxylate (6). L-Pyroglutamic acid 5 (387.3 g, 3 mol) was slurried in MeOH (1 L), thionyl chloride (652.1 g, 5.7 mol) was added in a manner so that the temperature was maintained between -5–0 °C. After complete addition of the thionyl chloride, the reaction mixture was heated to 25–30 °C and stirred for 5 h. Then the reaction mixture was evaporated under vacuum (T < 45 °C) to approximately 15% of its original volume. The remaining oil was then dissolved in toluene (1 L). Triethylamine (303.6 g, 3 mol) was added dropwise so that the temperature was maintained between -5–0 °C, then filtered. The filtrate was used in the next step directly. To another 5 L three necked bottle, Boc2O (870 g, 3.99 mol), 4-dimethlyaminopyridine (54.9 g, 0.45 mol) and toluene 1 L were combined and cooled to -5–0 °C. The filtrate was added dropwise while keep the tempreture between 0–5 °C. After addition, the reaction mixture was heated to 25–30 °C and stirred for 12 h (monitored by TLC, DCM/MeOH = 7/1). To the reaction vessel was added saturated aqueous NaCl (1 L) and 2 N HCl to adjust the pH to 2-3. The aqueous phase was extracted with EtOAc (200 mL×3), the combined organic layer was washed with brine and dried over magnesium sulfate. Concentrated under reduced pressure and the residue was stirred for 3 h, then filtered to give 6 (580.9 g, HPLC: 97.3%, yield: 80% for 2 steps) as an orange-red solid, the product can be used in the next step without further purification. [α]D20 - 29.7 (c 1.0, CHCl3). {lit.11 [α]D20 - 30.4 (c 1.0, CHCl3)}.
1H NMR (500 MHz, CDCl3) δ 4.63 (dd, J = 9.4, 3.0 Hz, 1H), 3.79 (s, 3H), 2.64-2.50 (m, 1H), 2.39-2.26 (m, 2H), 2.04 (ddt, J = 13.2, 9.6, 3.3 Hz, 1H), 1.50 (s, 9H). ESI-MS m/z: 244.2 (M+1)+.
(S)-1-tert-Butyl 2-methyl 2,3-dihydro-1H-pyrrole-1,2-dicarboxylate (7). To a stirred solution of compound 6 (580.9 g, 2.4 mol) in THF (3 L) at 0 °C was added LiEt3BH (1 M in THF, 2.6 L, 2.6 mol) droply while maintaining the batch temperature in the range of 5–10 °C. After stirring for 2 h, the reaction was cooled to 0 °C and quenched by careful addition of saturated aqueous ammonium chloride solution (1 L). The organic and aqueous layers were separated, the aqueous layer was extracted with DCM (500 mL×3). The combined organic layer was washed with brine (500 mL×3). Dried the organic layer over anhydrous sodium sulfate and distilled off the solvent completely to get a pale yellow oil. In a 3L three necked bottle, the crude product gained in the last step, potassium carbonate (524.4 g, 3.8 mol), acetic anhydride (388.0 g, 3.8 mol), DMF (1.8 L) were combined and heated to 120 °C. After stirring for 3 h, the reaction was cooled to 0 °C and quenched by careful addition of water (1 L). Then, added petroleum ether (1 L) .The reaction mixture was stirred for 20 min. Layers were separated, the aqueous layer was extracted with petroleum ether (500 mL×2). The combined organic layer was washed with brine (500 mL×2) and concertrated to get the title compound 7 as a yellow oily liquid (415.4 g, HPLC: 96.5%, yield: 76 % for 2 steps), which could be used in the next step without further purification.
1H NMR (500 MHz, CDCl3) δ 6.62 (m, 1H), 5.00 (m, 1H), 4.66 (dd, J = 12.0, 5.0 Hz, 1H), 3.80 (s, 3H), 3.17-2.99 (m, 1H), 2.82-2.57 (m, 1H), 1.50 (s, 9H). ESI-MS m/z: 228.2 (M+1)+.
(S)-tert-Butyl 2-carbamoyl-2,3-dihydro-1H-pyrrole-1-carboxylate (4). Compund 7 (415.0 g, 1.9 mol) was slurried in 2.1 L MeOH/water (2:1), lithium hydroxide monohydrate (119.6 g, 2.85 mol) was added in portions while maintaining the batch temperature in the range of -5–0 °C. After complete addition of the lithium hydroxide monohydrate, the reaction mixture was heated to 25–30 °C and stirring for 1 h, MeOH was removed by vacuum distillation and the aqueous layer was back-extracted with methyl tert-butyl ether. Add 1 L methyl tert-butyl ether to the aqueous layer and then add 20% phosphoric acid solution dropwise to adjust pH to 2–3. Layers were separated, the aqueous layer was extracted with 400 mL methyl tert-butyl ether. The combined organic phase was dried and filtered, methyl tert-butyl ether solution of intermediate was obtained, and the solution can be used in the next step directly. Transferred the methyl tert-butyl ether solution to a reaction vessel, cooled to -15 °C. Added N-methylmorpholine (252.5 g, 2.5 mol) and MsCl (228 g, 2 mol) dropwise respectively, while maintaining the batch temperature no more than -8 °C. The reaction mixture was stirred for 0.5 h under this temperaure. Ammonia gas was bubbled into and stirred for 1.5 h. Added saturated aqueous NaCl solution (1 L) to the solution and stirred for 1 h. Then add 20% phosphoric acid solution dropwise to adjust pH to 5–6. The organic layer washed with saturated aqueous NaHCO3 (500 mL×2),dried over anhydrous magnesium sulfate. Distilled off the solvent completely from the organic layer under reduced pressure to get the title compound 4 as pale yellow viscous, recrystallized from i-PrOH to form a off white solid (359.7 g, HPLC: 98.4%, yield: 89.2 % for 2 steps). 1H NMR (500 MHz, CDCl3) δ 7.31 (m, 1H), 6.88 (br, 1H), 6.48-6.45 (m, 1H), 4.95 (m, 1H), 4.34 (dd, J = 12.5, 6.0 Hz, 1H), 2.86-3.00 (m, 1H), 2.44-2.51 (m, 1H), 1.41 (s, 9H). ESI-MS m/z: 213.2 (M+1)+.
L-cis-4,5-Methanoproline amide (2). Zinc powder (6.5 g, 0.1 mol), copper bromide (1.4 g, 0.01 mol) and methyl tert-butyl ether (100 mL) were added respectively under nitrogen atmosphere. Diiodomethane (1.66 g, 0.5 mL) was added with stirring, then the solution was heated to reflux to initiate the reaction. After initiation of the reaction, the system would continue to reflux by itself. Added diiodomethane (26.8 g, 0.1 mol) dropwise, then stirring at 20 °C for 30 min. To the vessel the compound 4 (21.2 g, dissolved in 50 mL methyl tert-butyl ether) were added in a manner so that the temperature was maintained at 20 °C. After complete addition (about 1 h), the reaction were stirred for another 3.5 h. After the reaction was completed (monitored by TLC, hexane/EtOAc = 1/1.5), added saturated aqueous NaHCO3 solution (200 mL). Filtered it after 1 h stirring. The filter cake was washed with EtOAc (100 mL×2), and the filtrate was extracted with EtOAc (200 mL×2). Combination of organic phase, dried over anhydrous sodium sulfate. After removal of solvent by vacuum distillation we got a white solid. The crystallization was completed by addition of 200 mL MeOH /water (1:1 v/v) additional aging for 1 h at 20 °C. The solid was isolated by filtration (15.4 g, 68%), Diastereomeric ratio (dr = 40:1) was determined by HPLC analysis. [α]D20 33.7 (c 0.71, MeOH). {lit.4d [α]D20 35.2 (c 0.71, MeOH)}. Mp 56-58 ºC. 1H NMR (500 MHz, DMSO-d6) δ 6.30 (br s, 2H), 4.08 (s, 1H), 2.25-2.09 (m, 2H), 1.88-1.81 (m, 1H), 1.46 (s, 9H), 1.08 (s, 1H), 0.41-0.29 (m, 2H); 13C NMR (125 MHz, CDCl3) δ 172.1, 155.2, 81.7, 54.1, 38.8, 36.2, 28.4, 13.6, 9.1; ESI-MS m/z: 227.2 (M+1)+; HRMS calcd for C11H18N2NaO3 (M+Na) + requires 249.1215, found 249.1204.
ACKNOWLEDGEMENTS
This work was supported by the National Natural Science Foundation of China (Grants 21302126) and Shanghai Engineering Technology Research Center of Fragrance and Flavor (12DZ2251400).
References
1. D. J. Augeri, J. A. Robl, D. A. Betebenner, D. R. Magnin, A. Khanna, J. G. Robertson, A. Wang, L. M. Simpkins, P. Taunk, Q. Huang, S.-P. Han, B. Abboa-Offei, M. Cap, L. Xin, L. Tao, E. Tozzo, G. E. Welzel, D. M. Egan, J. Marcinkeviciene, S. Y. Chang, S. A. Biller, M. S. Kirby, R. A. Parker, and L. G. Hammann, J. Med. Chem., 2005, 48, 5025. CrossRef
2. Bristol-Myers Squibb and Astra Zeneca News Release, July 31st, 2009.
3. S. A. Savage, G. S. Jones, S. Kolotuchin, S. A. Ramrattan, T. Vu, and R. E. Waltermire, Org. Process Res. Dev., 2009, 13, 1169. CrossRef
4. (a) H. Stephen, R. Ulrich, S. Michel, and C. Stephen, Bioorg. Med. Chem. Lett., 1998, 8, 2123; CrossRef (b) M. Politino, M. M. Cadin, P. M. Skonezny, and J. G. Chen, WO2005106011, 2005; (c) R. Antonio, C. T. Vu, C. Steven, L. Thomas, N. Liu, E. W. Robert, and K. David, J. Org. Chem., 2014, 79, 6233; CrossRef (d) J. Z. Dong, Y. C. Gong, J. Liu, X. F. Chen, X. A. Wen, and H. B. Sun, Bioorg. Med. Chem., 2014, 22, 1383. CrossRef
5. (a) H. E. Simmons and R. D. Smith, J. Am. Chem. Soc., 1958, 80, 5323; CrossRef (b) H. E. Simmons and R. D. Smith, J. Am. Chem. Soc., 1959, 81, 4256; CrossRef (c) G. Wittig and K. Schwarzenbach, Angew. Chem., 1959, 71, 652; CrossRef (d) G. Wittig and F. Wingler, Chem. Ber., 1964, 97, 2139. CrossRef
6. (a) G. A. Molander and L. S. Harring, J. Org. Chem., 1989, 54, 3525; CrossRef (b) G. A. Molander and J. B. Etter, J. Org. Chem., 1987, 52, 3942. CrossRef
7. A. B. Charette and J. F. Marcoux, J. Am. Chem. Soc., 1996, 118, 4539. CrossRef
8. K. Maruoka, Y. Fukutani, and H. Yamamoto, J. Org. Chem., 1985, 50, 4412. CrossRef
9. (a) J. Furukawa, N. Kawabata, and J. Nishimura, Tetrahedron Lett., 1966, 7, 3353; CrossRef (b) J. Furukawa, N. Kawabata, and J. Nishimura, Tetrahedron, 1968, 24, 53. CrossRef
10. R. J. Rawson and I. T. Harrison, J. Org. Chem., 1970, 35, 2057. CrossRef
11. T. Katoh, Y. Nagata, Y. Kobayashi, K. Arai, J. Minami, and S. Terashima, Tetrahedron, 1994, 50, 6221. CrossRef