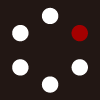
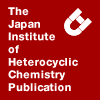
HETEROCYCLES
An International Journal for Reviews and Communications in Heterocyclic ChemistryWeb Edition ISSN: 1881-0942
Published online by The Japan Institute of Heterocyclic Chemistry
e-Journal
Full Text HTML
Received, 23rd April, 2015, Accepted, 20th May, 2015, Published online, 22nd May, 2015.
DOI: 10.3987/COM-15-13234
■ Development of a Facile and Inexpensive Route for the Preparation of α-Halobenzopyridines from α-Unsubstituted Benzopyridines
Osamu Sugimoto,* Hyuma Iwasaki, and Ken-ichi Tanji
Laboratory of Organic Chemistry, School of Food and Nutritional Sciences, University of Shizuoka, 552-1 Yada, Suruga-ku, Shizuoka 422-8526, Japan
Abstract
A facile and inexpensive route for the preparation of α-halobenzopyridines from α-unsubstituted benzopyridines via N-methylbenzopyridin-α-ones was developed. α-Unsubstituted benzopyridines were converted easily into the corresponding N-methylbenzopyridin-α-ones, which were halogenated using PPh3-TCICA or PPh3-DBICA without using solvent to give α-halobenzopyridines.Syntheses of benzopyridine (quinoline and isoquinoline) derivatives are important because their derivatives possess bioactivity.1 α-Halobenzopyridines, such as 2-haloquinolines and 1-haloisoquinolines, are useful intermediates because they can be converted into the corresponding α-substituted benzopyridines via nucleophilic aromatic substitution (SNAr) reactions,2 palladium-catalyzed coupling reactions,3 and halogen exchange reactions.4 The α-halobenzopyridines can be synthesized by reaction of the corresponding benzopyridin-α-ones with phosphorus oxyhalide (POX3). For example, reaction of quinolin-2(1H)-ones/isoquinolin-1(2H)-ones with POCl3 gave 2-chloroquinolines/1-chloroisoquinolines, respectively.5 However, this protocol required two problems to be solved: the poor availability of benzopyridin-α-ones and the intractability of POX3. Although several routes for the preparation of benzopyridin-α-ones from benzene derivatives via ring closure have been reported,6 these syntheses require many steps. Furthermore, many benzopyridin-α-ones are expensive or not commercially available. In addition, POX3 is so reactive with water that careful handling is required during the post-treatment process. Thus, a new route for the preparation of α-halobenzopyridines would be useful.
In this study, a facile and inexpensive route for the preparation of α-halobenzopyridines from N-methylbenzopyridin-α-ones (which are easily prepared from α-unsubstituted benzopyridines) was investigated using an easily prepared and safe halogenating reagent, PPh3-TCICA,7 as a substitute for the conventional chlorinating reagents POCl3 and PCl5.8 Furthermore, PPh3-DBICA, prepared from PPh3 and dibromoisocyanuric acid (DBICA), was used for bromination (Figure 1 and Scheme 1).
Prill et al. reported that reaction of pyridine with dimethyl sulfate gave 1-methylpyridinium salt followed by oxidation at the α-position using K3[Fe(CN)6] and NaOH at 0-10 °C to afford 1-methylpyridin-2(1H)-one in 65-70% yield.9 Using this method, substrates for the preparation of α-halobenzopyridines, N-methylbenzopyridin-α-ones, were synthesized from the corresponding α-unsubstituted benzopyridines in 44-66% yield (Method A, Table 1, entries 1-3). Interestingly, reaction using a simplified method (Method B: without K3[Fe(CN)6]) gave similar yields (28-55% yield, Table 1, entries 4-9). In the reaction using Method B, the air oxidation proceeded during the reaction or the work-up process to give the product, N-methylbenzopyridin-α-ones. Similar reaction is reported that 2-hydroxy-1,2-dihydropyridines undergo the air oxidation to afford 2(1H)-pyridinones.10 Although product yields were poor, this synthetic route has the advantage of inexpensive and easily available substrates.
Previous studies demonstrated that the reaction of quinolin-2(1H)-one with PPh3-TCICA (1) in toluene7 or in no solvent11 gave the chlorinated product, 2-chloroquinoline (Table 2, entries 1-4). In contrast, chlorination of 1-methylquinolin-2(1H)-one using 1 in toluene or xylene did not proceed (entries 5 and 6). These results indicate that halogenation of N-methylbenzopyridin-α-ones using 1 requires more drastic conditions than those used for conventional halogenation using toluene or xylene with reflux.
Halogenation of 1-methylquinolin-2(1H)-one derivatives using 1 (chlorination) or 2 (bromination) without solvent then was performed according a previously described protocol11 (Table 3).
Reaction of quinolin-2(1H)-one (Table 3) with a slight excess amount of 1 without solvent gave 2-chloroquinoline in good yield (entries 2-6), whereas a stoichiometric amount of 1 resulted in recovery of a significant amount of starting material substrate (entry 1). To clarify the generality of this reaction, 3-methyl, 6-methyl, 7-methyl, and 6-methoxy derivatives of quinolin-2(1H)-ones were chlorinated using 1.4-1.5 equiv. of 1 at 160-170 °C. The corresponding substituted 2-chloroquinoline products were obtained in 54-87% yield (entries 7-10). The bromination of the substrates using 2 also gave 2-bromoquinolines (entries 11-16).
Next, 1-haloisoquinolines were prepared by reaction of 2-methylisoquinolin-1(2H)-one with 1 or 2 (Table 4). The 1-chloroisoquinoline and 1-bromoisoquinoline products were obtained in good to moderate yield.
The proposed mechanism for the halogenation of N-methylbenzopyridin-α-ones is shown in Scheme 2. The oxygen atom in A and the phosphorus atom in 1 or 2 reacted to form intermediate B due to a high affinity of oxygen for phosphorus. Subsequent addition of X- and elimination of O=PPh3 formed the α-halobenzopyridinium salt C. The final step, elimination of a methyl group from C proceeded to give the product D.
In summary, a facile and inexpensive route for the preparation of α-halobenzopyridines from α-unsubstituted benzopyridines via N-methylbenzopyridin-α-ones was developed.
EXPERIMENTAL
The melting points were not corrected. 1H-NMR (90 MHz) spectra were obtained using a Hitachi R-90H spectrometer with tetramethylsilane (TMS) as an internal standard.
Preparation of 2-methylisoquinolin-1(2H)-one (general procedure for preparing N-methylbenzopyridin-α-ones)
Method A (Prill et al.9): In a flask (100 mL) equipped with a magnetic stirrer bar, a mixture of isoquinoline (776 mg, 6.01 mmol) and iodomethane (1306 mg, 9.20 mmol) in THF (10 mL) was heated to reflux for 1.5 h. Resulting solids were filtered, washed with EtOAc, and dried. The solids were dissolved in water (6 mL) and the solution cooled to 0-10 °C. Then, K3[Fe(CN)6] (2884 mg, 8.76 mmol) in water (6 mL) and NaOH (1428 mg, 35.7 mmol) in water (3 mL) were added simultaneously dropwise to keep the temperature below 10 °C. After an additional amount of K3[Fe(CN)6] (2887 mg, 8.77 mmol) in water (6 mL) was added, the reaction mixture was allowed to cool to rt and then stirred for 1.5 h. The mixture was neutralized with HCl and extracted with EtOAc. The organic layer was dried over sodium sulfate and separated using silica gel column chromatography eluted with hexane-EtOAc (1:2) to give 2-methylisoquinolin-1(2H)-one (632 mg, 66%).
Method B (simplified method, without K3[Fe(CN)6]): In a flask (100 mL) equipped with a magnetic stirrer bar, a mixture of isoquinoline (788 mg, 6.10 mmol) and iodomethane (4251 mg, 29.9 mmol) in dioxane (15 mL) was heated to reflux for 2 h. Resulting solids were filtered, washed with Et2O, and dried. A mixture of the solids and 1 N NaOH (12 mL) was stirred at rt for 20 h. The mixture was neutralized with HCl and extracted with EtOAc. The organic layer was dried over sodium sulfate and separated using silica gel column chromatography eluted with hexane-EtOAc (1:3) to give 2-methylisoquinolin-1(2H)-one (455 mg, 47%).
1-Methylquinolin-2(1H)-one:13 brown oil. 1H-NMR (CDCl3) δ: 3.71 (3H, s), 6.70 (1H, d, J=9.4 Hz), 7.07-7.70 (4H, m), 7.69 (1H, d, J=9.4 Hz).
1,3-Dimethylquinolin-2(1H)-one: yellow solid. Mp. 67-68 °C (lit.14 74 °C). 1H-NMR (CDCl3) δ: 2.26 (3H, d, J=0.9 Hz), 3.73 (3H, s), 7.05-7.65 (5H, m).
1,6-Dimethylquinolin-2(1H)-one: brown solid. Mp 74-75 °C (lit.15 83-84 °C). 1H-NMR (CDCl3) δ: 2.42 (3H, s), 3.70 (3H, s), 6.67 (1H, d, J=9.5 Hz), 7.10-7.50 (2H, m), 7.33 (1H, s), 7.60 (1H, d, J=9.5 Hz).
1,7-Dimethylquinolin-2(1H)-one: brown solid. Mp 105-106 °C (lit.16 105-107 °C). 1H-NMR (CDCl3) δ: 2.50 (3H, s), 3.70 (3H, s), 6.63 (1H, d, J=9.3 Hz), 7.04 (1H, d, J=8.0 Hz), 7.15 (1H, s), 7.43 (1H, d, J=8.0 Hz), 7.61 (1H, d, J=9.3 Hz).
6-Methoxy-1-methylquinolin-2(1H)-one:17 yellow solid. 1H-NMR (CDCl3) δ: 3.70 (3H, s), 3.86 (3H, s), 6.70 (1H, d, J=9.4 Hz), 6.99 (1H, d, J=2.3 Hz), 7.14 (1H, d, J=9.1 Hz), 7.30 (1H, d, J=9.1 Hz), 7.59 (1H, d, J=9.4 Hz).
2-Methylisoquinolin-1(2H)-one:18 brown oil. 1H-NMR (CDCl3) δ: 3.59 (3H, s), 6.46 (1H, d, J=7.3 Hz), 7.04 (1H, d, J=7.3 Hz), 7.30-7.75 (3H, m), 8.41 (1H, d, J=7.7 Hz).
Preparation of 2-chloroquinoline (general procedure for preparation of α-chlorobenzopyridine): In a flask (50 mL) equipped with a magnetic stirrer bar and balloon, a mixture of triphenylphosphine (1191 mg, 4.54 mmol) and trichloroisocyanuric acid (360 mg, 1.55 mmol) was heated under an argon atmosphere. During the heating process, triphenylphosphine melted, and trichloroisocyanuric acid vigorously reacted at 130-140 °C to form a dark brown oil, followed by further heating for 10 min. 1-Methylquinolin-2(1H)-one (242 mg, 1.50 mmol) was added to the mixture followed by heating at 130-140 °C for 3 h. Then, the reaction mixture was dissolved in CH2Cl2 and basified with triethylamine followed by silica gel column chromatography using hexane-EtOAc (6:1) as an eluate. The product 2-chloroquinoline (204 mg, 82%) was obtained.
2-Chloroquinoline:19 pale yellow liquid. 1H-NMR (CDCl3) δ: 7.37 (1H, d, J=8.5 Hz), 7.50-7.70 (1H, m), 7.70-7.91 (2H, m), 8.09 (2H, d, J=8.5 Hz).
2-Chloro-3-methylquinoline: white solid. Mp 81-82 °C (lit.20 84 °C). 1H-NMR (CDCl3) δ: 2.53 (3H, s), 7.35-7.85 (3H, m), 7.85-8.10 (1H, m), 7.95 (1H, s).
2-Chloro-6-methylquinoline: white solid. Mp 112-113 °C (lit.21 111-114 °C). 1H-NMR (CDCl3) δ: 2.53 (3H, s), 7.33 (1H, d, J=8.7 Hz), 7.42-7.70 (2H, m), 7.75-8.15 (2H, m).
2-Chloro-7-methylquinoline: white solid. Mp 79-80 °C (lit.22 83-84 °C). 1H-NMR (CDCl3) δ: 2.55 (3H, s), 7.20-7.50 (2H, m), 7.70 (1H, d, J=8.4 Hz), 7.79 (1H, s), 8.03 (1H, d, J=8.6 Hz).
2-Chloro-6-methoxyquinoline: white solid. Mp 94-95 °C (lit.23 106-107 °C). 1H-NMR (CDCl3) δ: 3.92 (3H, s), 7.06 (1H, d, J=2.7 Hz), 7.32 (1H, d, J=8.5 Hz), 7.37 (1H, dd, J=9.1 Hz, 2.7 Hz), 7.91 (1H, d, J=9.1 Hz), 7.98 (1H, d, J=8.5 Hz).
1-Chloroisoquinoline:24 pale yellow liquid. 1H-NMR (CDCl3) δ: 7.47-7.98 (4H, m), 8.15-8.48 (2H, m).
Preparation of 2-bromoquinoline (general procedure for preparation of α-bromobenzopyridine): In a flask (50 mL) equipped with a magnetic stirrer bar and a balloon, a mixture of triphenylphosphine (1186 mg, 4.52 mmol) and dibromoisocyanuric acid (652 mg, 2.27 mmol) was heated under an argon atmosphere. Dibromoisocyanuric acid vigorously reacted at 115 °C, and the mixture heated for an additional 10 min. 1-Methylquinolin-2(1H)-one (239 mg, 1.50 mmol) was added to the mixture, which was heated at 160-170 °C for 16 h. Then, the reaction mixture was dissolved in CH2Cl2 and basified with triethylamine, followed by separation using silica gel column chromatography. The use of hexane-EtOAc (6:1) as an eluate resulted in isolation of 2-bromoquinoline (244 mg, 78%).
2-Bromoquinoline:19 yellow oil. 1H-NMR (CDCl3) δ: 7.51 (1H, d, J=8.4 Hz), 7.51-8.20 (4H, m), 7.98 (1H, d, J=8.4 Hz).
2-Bromo-3-methylquinoline: light yellow powder (recryst. from hexane). Mp 90-91 °C. 1H-NMR (CDCl3) δ: 2.55 (3H, d, J=0.5 Hz), 7.35-7.85 (3H, m), 7.85-8.15 (2H, m). Anal. Calcd for C10H8BrN: C, 54.08; H, 3.63; N, 6.31. Found: C, 54.03; 3.85; N, 6.40.
2-Bromo-6-methylquinoline: white solid. Mp 109-111 °C (lit.25 117-118 °C). 1H-NMR (CDCl3) δ: 2.52 (3H, s), 7.30-7.70 (3H, m), 7.75-8.10 (2H, m).
2-Bromo-7-methylquinoline: yellow solid. Mp 79-80 °C. 1H-NMR (CDCl3) δ: 2.55 (3H, s), 7.25-7.55 (2H, m), 7.55-8.15 (3H, m).
2-Bromo-6-methoxyquinoline: white solid. Mp 122-127 °C (lit.26 110 °C). 1H-NMR (CDCl3) δ: 3.92 (3H, s), 7.05 (1H, d, J=2.8 Hz), 7.36 (1H, dd, J=9.1 Hz, 2.8 Hz), 7.45 (1H, d, J=8.6 Hz), 7.87 (1H, d, J=8.6 Hz), 7.93 (1H, d, J=9.1 Hz).
1-Bromoisoquinoline:27 yellow liquid. 1H-NMR (CDCl3) δ: 7.50-7.90 (4H, m), 8.15-8.45 (2H, m).
References
1. For example, procaterol (an intermediate-acting β2-adrenergic receptor agonist), quinine, primaquine, chloroquine (antimalarials), chinoiodine, chinoform (antiamebic agents), and papaverine (an opium alkaloid antispasmodic drug) are known as bioactive quinolineandisoquinoline derivatives.
2. S. Broch, B. Aboab, F. Anizon, and P. Moreau, Eur. J. Med. Chem., 2010, 45, 1657. CrossRef
3. C. W. Cheung, D. S. Surry, and S. L. Buchwald, Org. Lett., 2013, 15, 3734. CrossRef
4. A. C. Bissember and M. G. Banwell, J. Org. Chem., 2009, 74, 4893. CrossRef
5. C. E. Kaslow and W. M. Lauer, Org. Synth., Coll. Vol. 3, 1955, 194; S. A. Gamage, J. A. Spicer, G. W. Rewcastle, J. Milton, S. Sohal, W. Dangerfield, P. Mistry, N. Vicker, P. A. Charlton, and W. A. Denny, J. Med. Chem., 2002, 45, 740. CrossRef
6. C. W. Holzapfel and C. Dwyer, Heterocycles, 1998, 48, 215; CrossRef M. Suzuki, Y. Ohuchi, H. Asanuma, T. Kaneko, S. Yokomori, C. Ito, Y. Isobe, and M. Muramatsu, Chem. Pharm. Bull., 2000, 48, 2003; CrossRef J. C. Jung, Y. J. Jung, and O. S. Park, J. Heterocycl. Chem., 2001, 38, 61; CrossRef A. Detsi, V. Bardakos, J. Markopoulos, and O. Igglessi-Markopoulou, J. Chem. Soc., Perkin Trans. 1, 1996, 2909; CrossRef N. A. Cortese, C. B. Ziegler Jr, B. J. Hrnjez, and R. F. Heck, J. Org. Chem., 1978, 43, 2952; CrossRef J. L. Garcia Ruano, C. Pedregal, and J. H. Rodriguez, Heterocycles, 1991, 32, 2151; CrossRef W. H. Perkin Jr. and R. Robinson, J. Chem. Soc., 1913, 103, 1973; CrossRef T. L. Jacobs, S. Winstein, G. B. Linden, J. H. Robson, E. F. Levy, and D. Seymour, Org. Synth., 1948, 28, 70; CrossRef K. Tomita, Yakugaku Zasshi, 1951, 71, 1100; M. Rehwald, K. Gewald, H. J. Lankau, and K. Unverferth, Heterocycles, 1997, 45, 483; CrossRef W. M. Lauer and C. E. Kaslow, Org. Synth., 1944, 24, 68; CrossRef S. Nakano, Yakugaku Zasshi, 1962, 82, 498; C. R. Hauser and G. A. Reynolds, J. Am. Chem. Soc., 1948, 70, 2402; CrossRef S. Nakano, Yakugaku Zasshi, 1962, 82, 492; U. Mavers, F. Berruex, and M. Schlosser, Tetrahedron, 1996, 52, 3223; CrossRef F. Effenberger and W. Hartmann, Chem. Ber., 1969, 102, 3260; CrossRef J. H. M. Lange, P. C. Verveer, S. J. M. Osnabrug, and G. M. Visser, Tetrahedron Lett., 2001, 42, 1367; CrossRef A. K. Bose, M. S. Manhas, V. V. Rao, C. T. Chen, I. R. Trehan, S. D. Sharma, and S. G. Amin, J. Heterocycl. Chem., 1971, 8, 1091; CrossRef S. Gabriel, Ber., 1916, 49, 1608; CrossRef N. Gautier and R. H. Dodd, Synth. Commun., 1998, 28, 3769; CrossRef J. W. Wilson III, E. L. Anderson, and G. E. Ullyot, J. Org. Chem., 1951, 16, 800; CrossRef A. Sugimoto, H. Shinba-Tanaka, and M. Ishikawa, Synthesis, 1995, 431; CrossRef G. Simchen, Angew. Chem., Int. Ed. Engl., 1966, 5, 663; CrossRef G. Simchen and W. Kramer, Chem. Ber., 1969, 102, 3656; CrossRef G. S. Poindexter, J. Org. Chem., 1982, 47, 3787; CrossRef E. M. Brun, S. Gil, R. Mestres, and M. Parra, Synthesis, 2000, 273; CrossRef T. Sakamoto, M. An-naka, Y. Kondo, and H. Yamanaka, Chem. Pharm. Bull., 1986, 34, 2754; CrossRef N. Yagi, H. Oomori, and M. Okazaki, Yuki Gosei Kagaku Kyokaishi, 1969, 27, 51; CrossRef T. Sakamoto, Y. Kondo, and H. Yamanaka, Chem. Pharm. Bull., 1985, 33, 626; CrossRef G. M. Sanders, M. van Dijk, and H. J. den Hertog, Recl. Trav. Chim. Pays-Bas, 1976, 95, 31; CrossRef T. Nagase, Bull. Chem. Soc. Jpn., 1964, 37, 1175. CrossRef
7. O. Sugimoto and K. Tanji, Heterocycles, 2005, 65, 181. CrossRef
8. W. H. Perkin Jr. and R. Robinson, J. Chem. Soc., 1912, 101, 1775; CrossRef A. Nuvole and G. A. Pinna, J. Heterocycl. Chem., 1978, 15, 1513. CrossRef
9. E. A. Prill and S. M. McElvain, Org. Synth., 1935, XV, 41. CrossRef
10. K. Hayakawa, T. Yasukouchi, and K. Kanematsu, Tetrahedron Lett., 1987, 28, 5895. CrossRef
11. O. Sugimoto, Y. Harada, and K. Tanji, Heterocycles, 2012, 86, 1583. CrossRef
12. When a substrate (1.50 mmol) and 1 (1.50 mmol) is reacted, the molar ratio is 3.0 because 1 has a threefold chlorinating availability. Similarly, when a substrate (1.50 mmol) and 2 (1.50 mmol) was reacted, the molar ratio is 2.0 because 2 has a twofold brominating availability (see the structure of 1 and 2 shown in Figure 1).
13. J. C. Schoeneboom, S. Groetsch, M. Christl, and B. Engels, Chem. Eur. J., 2003, 9, 4641. CrossRef
14. H. Ahlbrecht, Chem. Ber., 1975, 108, 2300. CrossRef
15. Y. Wu, C. Chuang, and P. Lin, Tetrahedron, 2000, 56, 6209. CrossRef
16. D. J. Cook, R. S. Yunghans, T. R. Moore, and B. E. Hoogenboom, J. Org. Chem., 1957, 22, 211. CrossRef
17. O. Fischer and M. Chur, J. Prakt. Chem., 1916, 93, 363. CrossRef
18. Y. Nakao, H. Idei, K. S. Kanyiva, and T. Hiyama, J. Am. Chem. Soc., 2009, 131, 15996. CrossRef
19. O. Sugimoto, M. Mori, K. Moriya, and K. Tanji, Helv. Chim. Acta, 2001, 84, 1112. CrossRef
20. O. Meth-Cohn, S. Rhouati, B. Tarnowski, and A. Robinson, J. Chem. Soc., Perkin Trans. 1, 1981, 1537. CrossRef
21. B. S. Lee, J. H. Lee, and D. Y. Chi, J. Org. Chem., 2002, 67, 7884. CrossRef
22. S. Inglis, R. Jones, D. Fritz, C. Stojkoski, G. Booker, and S. Pyke, Org. Biomol. Chem., 2005, 3, 2543. CrossRef
23. G. B. Bachman, J. Org. Chem., 1944, 9, 302. CrossRef
24. G. A. Molander and I. Shin, Org. Lett., 2011, 13, 3956. CrossRef
25. E. J. V. Conolly, J. Chem. Soc., 1925, 127, 2083. CrossRef
26. S. E. Wengryniuk, A. Wiickgenannt, C. Reiher, N. A. Strotman, K. Chen, M. D. Eastgate, and P. S. Baran, Org. Lett., 2013, 15, 792. CrossRef
27. H. E. Jansen and J. P. Wibaut, Recl. Trav. Chim. Pays-Bas., 1937, 56, 699. CrossRef