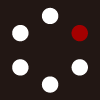
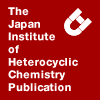
HETEROCYCLES
An International Journal for Reviews and Communications in Heterocyclic ChemistryWeb Edition ISSN: 1881-0942
Published online by The Japan Institute of Heterocyclic Chemistry
e-Journal
Full Text HTML
Received, 8th June, 2015, Accepted, 11th September, 2015, Published online, 24th September, 2015.
■ Efficient Microwave-Assisted Syntheses of A Series of Novel Mono(imino)pyrrolyl Compounds
Biyun Su,* Xiaoteng Li, Xudong Wang, and Qianding Li
College of Chemistry and Chemical Engineering, Xi’an Shiyou University, 18, 2nd Dianzi Road, Xi’an, Shanxi 710065, China
Abstract
A series of novel mono(imino)pyrroles that were hard to prepare under traditional solvent reaction condition were readily synthesized under solvent free condition using microwave chemistry via p-TSA (p-toluenesulfonic acid) catalyzed Schiff base condensation. The structures of the compounds were characterized by means of MS, 1H NMR, IR, elementary analysis and X-ray diffraction analysis. The reactivity of different aromatic amines with 2-acetylpyrrole was discussed.In recent years, late transition metal complexes with bis(imino)pyridyl ligands have emerged as a family of active catalysts for olefin polymerization or oligomerization.1 After that, iminopyrrolides and related five-membered chelate ligands attracted attention of chemists.2 Among these ligands, the symmetric bis(imino)pyrrole was the most reported ligand, which was designed and synthesized to prepare new transition metal complexes.3 Some of the complexes were able to catalyze α-olefin polymerization,4 some were highly active for the living copolymerization of ethylene and norbornene.5 In contrast to the symmetric bis(imino)pyrrole, little attention was paid to asymmetric mono(imino)pyrrolyl derivatives. To the best of our knowledge, only a limited number of mono(imino)pyrrole and their metal complexes were reported in literature. For most of them, the side-arm of pyrrole imino was H atom.4 In order to restore the original backbone of the classic bis(imino)pyridine ligand, CH3 was adopted as a side-arm to replace H atom to construct a series of new mono(imino)pyrrolyl compounds. An efficient method was also developed to synthesize this type of compounds. As shown in Scheme 1, the mono(imino)pyrroles with CH3 side-arm were readily prepared by condensation of precursor 2-acetylpyrrole with a series of aromatic amines under solvent free condition using microwave chemistry.6 The present approach offered several advantages such as shorter reaction time, better yields, lower costs and less pollution as compared with the conventional liquid phase synthesis. The reported synthesis methods for 2-acetylpyrrole (2), which was the precursor of mono(imino)pyrroles ligand, mainly included Vilsmeier reaction, Oddo reaction and Friedel-Crafts reaction.7 For the Friedel- Crafts reaction, several new strategies were adopted to improve efficiency of the acylation of pyrrole.8 Herein we adopted acetic anhydride and N,N-dimethylacetamide as acetylation reagents to convert pyrrole (1) into 2-acetylpyrrole (2) respectively. The experimental results discovered that trace water and oxygen in reaction would greatly affect the isolated yield when acetic anhydride was adopted as acetylation reagent, acetic anhydride was apt to hydrate and pyrrole monomer was apt to polymerize, which would lead to the decrease of yield of 2-acetylpyrrole to 12.6%. On the contrary, selecting of N,N-dimethylacetamide as acetylation reagent seemed to be better in terms of mild reaction condition, relatively single product, high yields for 2, which could reach 68% (Scheme 1).
Schiff base condensation was generally performed by stirring the mixture of aldehyde (or ketone) and amine in organic medium with acid catalysis. However, the experiment showed that this traditional liquid phase synthesis was not suitable for the Schiff base condensation of 2-acetylpyrrole with aromatic amine. The attempt was failed to prepare mono(imino)pyrroles by catalytic condensation in organic solvent, whether the reaction was conducted at room temperature for a long stirring time (up to 72 h) or refluxed for a reasonable period in ethanol in the presence of HOAc (or p-TSA). Unexpectedly, when p-TSA catalyzed microwave chemistry was applied, compounds 3a-3j was afforded, as depicted in Scheme 1. Compared with the aforementioned liquid phase synthesis, microwave assisted condensation showed several advantages such as simplified work-up procedure, improved reaction rate and cleaner products. Most importantly, it could facilitate a reaction happening readily which could not happen under traditional liquid phase reaction conditions.
In order to obtain the best yield for the mono(imino)pyrroles 3a-3j, a series of experiment was performed under different reaction time and temperature. The experimental results showed that the optimum reaction time is in the range of 5-10 min, and the most suitable temperature is in the range of 120-180 °C. After purification, all compounds were isolated as air-stable solid in high purity and in different yields (20.54 - 73.9%). The structures of 3a-3j were proved by IR, 1H NMR, MS and elementary analysis, as well as X-ray crystallographic diffraction.9 The IR spectra show that the CN stretching frequencies appear in the range of 1603.0-1615.4 cm-1. The elemental analysis results suggest that the components of 3a-3j are in accord with the formula of C6H7N2Ar (Ar infers to substituted benzene group), which agrees with the expected Schiff-base condensation products. 1H NMR spectra also give the reasonable chemical shifts for the desired product. Take 1H NMR of 3a for example, the spectrum of N-H at pyrrole ring consists with a single gentle peak at 9.84 ppm, the spectra of aromatic H at the benzene ring consist of two triplet at 7.34, 6.82 ppm and a doublet at 6.67 ppm respectively and integrals in a 2 : 2 : 1 ratio. The spectra of pyrrole ring aromatic H consist of a triplet and two doublet at 7.09, 6.83, 6.82, 6.23 respectively and integrals in a ration of 1 : 1 : 1. Methyl group connected with imino -C=N-Ar consists of a single peak at 2.12 ppm due to the three H atoms are in an identical chemical environment. The 1H NMR of 3b-3j can also well explain the structure of molecules. The molecular structure of 2-{1-[(4-ethylphenyl)imino]ethyl}pyrrole (3d) is further confirmed by single-crystal X-ray crystallography. The compound crystallized in triclinic system with space group P-1, a = 6.7857(2) Å, b = 9.234(2) Å, c = 10.341(2) Å, α = 73.820(4)°, β = 72.710(4)°, γ = 88.369(4)°, Z = 2 and V = 593.1(2) Å3. The molecular structure of 3d is shown in Figure 1. Experimental and refinement details can be found in the supplementary data.
During the experiment, we examined the reactivity of a series of substituted anilines and 2-acetylpyrrole. It was found that these substituted anilines showed very different reactivity under similar reaction conditions (5-10 min and 120-180 °C). Anilines containing alkyl group comply with the reactivity order of 4-ethylaniline > 2,5-dimethylaniline > 3,4-dimethylaniline > aniline > 2-methylaniline > 2,4,6-trimethylaniline > 2,6-dimethylaniline > 2,6-diethylaniline. Anilines containing chlorine group comply with the reactivity order of p-chloroaniline > m-chloroaniline > o-chloroaniline (unreacted). The trend of isolated yield is consistent with the reactivity. It could be reasonably inferred that the rate constant and equilibrium constant of the reaction are affected by the position and size of the substituted group in aniline. The bigger ortho-substituted group will cause slower rate and lower yield, while the meta-substituted group might be favorable for higher reaction rate and yield in a sense.
The mechanism for preparing mono(imino)pyrroles is a typical nucleophilic addition reaction, in which several transition states might be involved. According to Transition State Theory (TST), in each step, the colliding molecules must have sufficient energy to overcome a potential energy barrier (the activation energy). The rate of a reaction is determined by the number of activated complexes formed and the frequency with which they go over to the final state. In traditional liquid phase synthesis the energy of activated complexes are provided by external heat source, which is comparatively slow and inefficient for transferring energy into the reaction system. In contrast, microwave irradiation produces efficient internal heating by direct coupling of microwave energy with the molecules presented in the reaction mixture.10 In addition to that, there are other thermal effects that can accelerate the chemical transformations in a microwave field but cannot be achieved or duplicated by conventional heating. Microwave facilitates the polarization of molecules under irradiation causing rapid reaction to occur. This is consistent with the reaction mechanism, which involves several polar transition states.11 The different interaction ways of the above two heaters well explain the fact that why the microwave can readily accelerate the reactions of the Schiff base condensation between 2-acetylpyrrole and aromatic amines, while it is not easy to happen in conventional reaction of liquid phase.
EXPERIMENTAL
C, H and N analyses were performed by a HP-MOD 1106 microanalyzer. IR spectra were carried out with a Perkin-Elemer FTIR 2000 spectrometer. 1H NMR spectra were recorded on a Bruker AVANCE III 500 spectrometer using TMS as internal standard. Mass spectra were determined with a Kratos AEI MS-50 instrument using electron impact (EI) method. The microwave-assisted condensations were performed in a Microwave Synthesis / Extraction System (MAS-II), from Shanghai Sineo Microwave Chemistry Technology Co., Ltd. Melting points determined with a melting apparatus were uncorrected. Pyrrole, N,N-dimethylacetamide, aniline, 2,4,6-trimethylaniline, 3,4-dimethylaniline, 4-ethylaniline, 2,5-dimethylaniline, 2,6-dimethylaniline, 2,6-diethylaniline, 2-methylaniline, 4-chloroaniline, 3-chloroaniline were purchased from Albemarle Co. (USA).
Synthetic Procedure of 2-Acetylpyrrole (2)
10.4 g (0.12 mol) of N, N-Dimethylacetamide in 20 mL anhydrous benzene was cooled by ice water bath to 0 °C, to which 17 g (0.11 mol) of phosphorus oxychloride in 30 mL anhydrous benzene was slowly added with stirring within 30 min. After removing the ice bath, the mixture was stirred at room temperature for 50 min. The reaction system was cooled again to 0 °C, 6.8 g (0.10 mol) of pyrrole in 15 mL anhydrous benzene was added slowly into the reaction mixture within 20 min. After stirring at room temperature for 10-12 h, 100 mL ice water was added, solid sodium bicarbonate and 40% sodium hydroxide solution were adopted in sequence to adjust pH of the reaction solution to 7 and 12 respectively. The obtained mixture was stirred at room temperature for 3 h. Benzene organic layer was separated out, and the residual aqueous phase was extracted twice into CHCl3 (30 mL × 2). The obtained CHCl3 layer was combined with the benzene organic layer, then washed with 30 mL of water and dried by anhydrous magnesium sulfate. Removal of solvent on a rotary evaporator yielded 9 g of crude brown solid, which was recrystallized by cyclohexane to afford white acicular crystal in 68% (7.4 g) yield. mp 90-91 °C. IR (KBr): νN-H 3258 cm-1, νC=O 1640 cm-1. 1H NMR (500MHz, CDCl3): δ 7.05 (d, 1H, pyrrole ring aromatic H), 6.88 (m, 1H, pyrrole ring aromatic H), 6.13 (d, 1H, pyrrole ring aromatic H), 2.39 (s, 3H, -N=C(CH3)-), MS (EI): m/z 109 (M). Anal. Calcd for C6H7NO: C, 66.04; H, 6.47; N, 12.84. Found: C, 65.98; H, 6.74; N, 12.60.
General Synthetic Procedure of 3a-3j
Method a (traditional liquid synthesis, failed)
4.20 Mmol of aromatic amine in about 10 mL EtOH was added dropwise into a EtOH (about 25 mL) solution of 2-acetylpyrrole (3.40 mmol), small amount of acetic acid (or p-TSA) was added as catalyst. The reaction mixture was stirred at room temperature for 72 h, using TLC to monitor process of the reaction. The experimental results showed that there was almost no reaction happening under this condition. The reaction mixture was then refluxed at 78 °C with stirring for more than 3.5 h, TLC did not detect obvious reaction signs.
Method b (microwave-assisted condensations, succeeded)
The microwave-assisted condensations of 2-acetylpyrrole (2) and aromatic amines were performed in a Microwave Synthesis/Extraction System (MAS-II). 2 (2.0 mmol) and excess of aromatic amine (2.5 mmol) were put into an open vessel, the mixture was irradiated at optimal temperature for a reasonable time, the obtained mixture was purified by column chromatography on silica gel, if necessary, supplemented by recrystallization method to afford highly pure products. The products 3a-3j were fully characterized by IR, 1H NMR, mass spectrum and elemental analysis. The microwave-assisted reaction temperature, reaction time, yields, melting points and elutes used to purify products 3a-3j are summarized in Table 1. Spectral data of these compounds are listed as below:
2-{1-[(Phenyl)imino]ethyl}pyrrole (3a)
IR (KBr): νC═N 1607.2 cm-1. 1H NMR (500MHz, CD2Cl2): δ 9.84 (s, 1H, pyrrole N-H), 7.34 (t, 2H, benzene ring aromatic H), 7.09(t, 1H, pyrrole ring aromatic H), 6.83 (d, 1H, pyrrole ring aromatic H), 6.82 (t, 2H, benzene ring aromatic H), 6.67 (d, 1H, benzene ring aromatic H), 6.23 (d, 1H, pyrrole ring aromatic H), 2.12 (s, 3H, -N=C(CH3)-). MS (EI): m/z 184 (M). Anal. Calcd for C12H12N2: C, 78.23; H, 6.57; N, 15.21. Found: C, 78.50; H, 7.01; N, 14.96.
2-{1-[(2,4,6-Trimethylphenyl)imino]ethyl}pyrrole (3b)
IR (KBr): νC═N 1603.0 cm-1. 1H NMR (500MHz, CD2Cl2): δ 9.85 (s, 1H, pyrrole N-H), 6.87 (t, 2H, benzene ring aromatic H), 6.74(t, 1H, pyrrole ring aromatic H), 6.64 (d, 1H, pyrrole ring aromatic H), 6.21 (t, 1H, pyrrole ring aromatic H), 2.27 (s, 3H, -N═C(CH3)-), 1.97 (d, 6H, phenyl-CH3), 1.92 (d, 3H, phenyl-CH3). MS (EI): m/z 225 (M+). Anal. Calcd for C15H18N2: C, 79.61; H, 8.02; N, 12.38. Found: C, 79.71; H, 7.96; N, 12.39.
2-{1-[(3,4-Dimethylphenyl)imino]ethyl}pyrrole (3c)
IR (KBr): νC═N 1609.6 cm-1. 1H NMR (500 MHz, CD2Cl2): δ 9.59 (s, 1H, pyrrole N-H), 7.07 (d, 1H, benzene ring aromatic H), 6.89 (t, 1H, pyrrole ring aromatic H), 6.63 (d, 1H, benzene ring aromatic H), 6.58 (s, 1H, pyrrole ring aromatic H), 6.52 (d, 1H, benzene ring aromatic H), 6.23 (t, 1H, pyrrole ring aromatic H), 2.24 (d, 6H, phenyl-CH3), 2.10 (s, 3H, -N=C(CH3)-). MS (EI): m/z 212 (M). Anal. Calcd for C14H16N2: C, 79.21; H, 7.60; N, 13.20. Found: C, 79.05; H, 7.49; N, 12.91.
2-{1-[(4-Ethylphenyl)imino]ethyl}pyrrole (3d)
IR (KBr): νC═N 1611.3 cm-1. 1H NMR (500MHz, CD2Cl2): δ 9.80 (s, 1H, pyrrole N-H), δ 7.33 (t, 2H, benzene ring aromatic H), 6.90 (d, 1H, pyrrole ring aromatic H), 6.82 (t, 2H, benzene ring aromatic H), 6.71 (t, 1H, pyrrole ring aromatic H), 6.35 (d, 1H, pyrrole ring aromatic H), 4.02 (m, 2H, phenyl-(CH2)-CH3), 2.15 (t, 3H, phenyl-CH2-(CH3)), 2.05 (s, 3H, -N=C(CH3)-). MS (EI): m/z 212 (M+). Anal. Calcd for C14H16N2: C, 79.21; H, 7.60; N, 13.20. Found: C, 79.35; H, 7.54; N, 12.94.
2-{1-[(2,5-Dimethylphenyl)imino]ethyl}pyrrole (3e)
IR (KBr): νC═N 1612.5 cm-1. 1H NMR (500MHz, CD2Cl2): δ 9.89 (s, 1H, pyrrole N-H), 7.08 (t, 1H, benzene ring aromatic H), 6.82 (t, 1H, benzene ring aromatic H), 6.75(t, 1H, pyrrole ring aromatic H), 6.65 (d, 1H, pyrrole ring aromatic H), 6.49 (d, 1H, benzene ring aromatic H), 6.22 (t, 1H, pyrrole ring aromatic H), 2.30 (s, 3H, -N=C(CH3)-), 2.04 (s, 6H, phenyl-CH3). MS (EI): m/z 212 (M). Anal. Calcd for C14H16N2: C, 79.21; H, 7.60; N, 13.20. Found: C, 79.52; H, 7.33; N, 12.94.
2-{1-[(2,6-Dimethylphenyl)imino]ethyl}pyrrole (3f)
IR (KBr): νC═N 1610.2 cm-1. 1H NMR (500MHz, CD2Cl2): δ 9.77 (s, 1H, pyrrole N-H), 7.04 (t, 2H, benzene ring aromatic H), 6.89 (t, 1H, benzene ring aromatic H), 6.81(t, 1H, pyrrole ring aromatic H), 6.65 (d, 1H, pyrrole ring aromatic H), 6.23 (t, 1H, pyrrole ring aromatic H), 2.01 (s, 6H, phenyl-CH3), 1.93 (s, 3H, -N=C(CH3)-). MS (EI): m/z 212 (M). Anal. Calcd for C14H16N2: C, 79.21; H, 7.60; N, 13.20. Found: C, 79.55; H, 7.44; N, 13.59.
2-{1-[(2,6-Diethylphenyl)imino]ethyl}pyrrole (3g)
IR (KBr): νC═N 1612.3 cm-1. 1H NMR (500MHz, CD2Cl2): δ 9.75 (s, 1H, pyrrole N-H), 7.08 (d, 2H, benzene ring aromatic H), 6.98 (t, 1H, benzene ring aromatic H), 6.77(d, 1H, pyrrole ring aromatic H), 6.64 (d, 1H, pyrrole ring aromatic H), 6.23 (t, 1H, pyrrole ring aromatic H), 2.36 (m, 4H, phenyl-(CH2)CH3), 1.95 (s, 3H, -N=C(CH3)-), 1.10 (t, 6H, phenyl-CH2(CH3)). MS (EI): m/z 240 (M). Anal. Calcd for C16H20N2: C, 79.96; H, 8.39; N, 11.66. Found: C, 79.72; H, 7.98; N, 11.64.
2-{1-[(2-Methylphenyl)imino]ethyl}pyrrole (3h)
IR (KBr): νC═N 1609.0 cm-1. 1H NMR (500MHz, CD2Cl2): δ 9.76 (s, 1H, pyrrole N-H), 7.20 (t, 1H, benzene ring aromatic H), 7.15 (t, 1H, benzene ring aromatic H), 6.99 (t, 1H, benzene ring aromatic H), 6.82 (t, 1H, pyrrole ring aromatic H), 6.66 (d, 1H, benzene ring aromatic H), 6.65 (d, 1H, pyrrole ring aromatic H), 6.23 (t, 1H, pyrrole ring aromatic H), 2.08 (s, 3H, -N=C(CH3)-), 2.04 (s, 3H, phenyl-CH3). MS (EI): m/z 197 (M+). Anal. Calcd for C13H14N2: C, 78.75; H, 7.12; N, 14.13. Found: C, 78.32; H, 6.95; N, 14.58.
2-{1-[(4-Chlorophenyl)l)imino]ethyl}pyrrole (3i)
IR (KBr): νC═N 1614.3 cm-1. 1H NMR (500MHz, CD2Cl2): δ 9.59 (s, 1H, pyrrole N-H), 7.29 (d, 2H, benzene ring aromatic H), 6.90 (d, 1H, pyrrole ring aromatic H), 6.74 (t, 2H, benzene ring aromatic H), 6.68 (t, 1H, pyrrole ring aromatic H), 6.25 (d, 1H, pyrrole ring aromatic H), 2.10 (s, 3H, -N=C(CH3)-). MS (EI): m/z 217 (M+). Anal. Calcd for C12H11ClN2: C, 65.91; H, 5.07; N, 12.81. Found: C, 66.29; H, 4.82; N, 12.87.
2-{1-[(3-Chlorophenyl)l)imino]ethyl}pyrrole (3j)
IR (KBr): νC═N 1615.4 cm-1. 1H NMR (500MHz, CD2Cl2): δ 9.58 (s, 1H, pyrrole N-H), 7.30 (d, 2H, benzene ring aromatic H), 6.92 (s, 1H, pyrrole ring aromatic H), 6.76 (d, 1H, benzene ring aromatic H), 6.74 (d, 1H, benzene ring aromatic H), 6.68 (d, 1H, pyrrole ring aromatic H), 6.26 (t, 1H, pyrrole ring aromatic H), 2.11 (s, 3H, -N=C(CH3)-). MS (EI): m/z 217 (M+). Anal. Calcd for C12H11ClN2: C, 65.91; H, 5.07; N, 12.81. Found: C, 65.52; H, 4.88; N, 12.60.
SUPPLEMENTARY DATA
Experimental and refinement details of the X-ray crystallographic structure of compound 3d can be obtained free of charge from the Cambridge Crystallographic Data Centre (http://www.ccdc. cam.ac.uk), reference codes CCDC 990761.
ACKNOWLEDGEMENTS
We are grateful to the National Natural Science Foundation of China, the Scientific Research Plan Project of Shaanxi Education Department (No. 12JK0620) and the Science and Technology Research Program of Shaanxi Province (No. 2013 KJXX-33) for financial support.
References
1. B. L. Small, M. Brookhart, and A. M. A. Bennett, J. Am. Chem. Soc., 1998, 120, 4049; CrossRef G. J. P. Britovsek, M. Bruce, V. C. Gibson, B. S. Kimberley, P. J. Maddox, S. Mastroianni, S. J. McTavish, C. Redshaw, G. A. Solan, S. Strömberg, A. J. P. White, and D. J. Williams, J. Am. Chem. Soc., 1999, 121, 8728; CrossRef B. Y. Su and G. X. Feng, Polymer International, 2010, 59, 1058; B. Y. Su, J. S. Zhao, Q. Z. Zhang, and W. L. Qin, Polymer International, 2009, 58, 1051. CrossRef
2. K. Tenza, M. J. Hanton, and A. M. Z. Slawin, Organometallics, 2009, 28, 4852; CrossRef B. Y. Su, H. B. Ta, and Q. Z. Zhang, Chinese J. Catal., 2011, 32, 1439.
3. V. C. Gibson and S. K. Spitzmesser, Chem. Rev., 2003, 103, 283; CrossRef Y. Matsuo, K. Mashima, and K. Tani, Chem. Lett., 2000, 1114. CrossRef
4. K. Mashima and H. Tsurugia, J. Organomet. Chem., 2005, 690, 4414. CrossRef
5. Y. Yoshida, J. Saito, M. Mitani, Y. Takagi, S. Matsui, S. Ishii, T. Nakano, N. Kashiwa, and T. Fujita, Chem. Commun., 2002, 1298. CrossRef
6. A. Davoodnia, M. M. Heravi, Z. Safavi-Rad, and N. Tavakoli-Hoseini, Synth. Commun., 2010, 40, 2588; CrossRef H. Valizadeh and A. Shockravi, Synth. Commun., 2009, 39, 4341; CrossRef S. Sobhani and Z. Tashrifi, Synth. Commun., 2009, 39, 120. CrossRef
7. F. H. Men, S. F. Zhang, W. F. Gao, and G. W. Chen, Fine Chemicals, 1996, 1316; D. O. A. Garrido, G. Buldain, and B. Frydman, J. Org. Chem., 1984, 49, 2619. CrossRef
8. G. Y. Bai, Y. H. Yang, Z. Ma, and J. Z. Xu, Res. Chem. Intermed., 2012, 38, 795; CrossRef K. Suzuki, A. R. Katritzky, S. K. Singh, and H. Y. He, J. Org. Chem., 2003, 68, 5720; CrossRef J. E. Taylor, M. D. Jones, J. M. Williams, and S. D. Bull, Org. Lett., 2010, 12, 5740; CrossRef F. D. Vasco, A. F. Álvaro, E. G. Brigas, J. P. Derouane, and B. S. S. Lourenço, J. Mol. Catal. A: Chem., 2009, 305, 100; CrossRef J. S. Yadav, B. V. S. Reddy, G. Kondaji, R. S. Rao, and S. P. Kumar, Tetrahedron Lett., 2002, 43, 8133. CrossRef
9. B. Y. Su, J. X. Wang, X. Liu, and Q. D. Li, Acta Cryst. C, 2013, 69, 1073; CrossRef B. Y. Su, J. X. Wang, X. Liu, and Q. D. Li, Acta Cryst. C, 2013, 69, 522; CrossRef B. Y. Su, W. L. Qin, L. Jiao, and J. X. Wang, Acta Cryst. E, 2012, 68, o3326; CrossRef B. Y. Su, L. Li, J. X. Wang, and X. Y. Li, Acta Cryst. E, 2012, 68, o2888. CrossRef
10. C. O. Kappe and A Stadler, In “Microwaves in Organic and Medicinal Chemistry” Wiley-VCH, Weinheim, 2005, 73. CrossRef
11. O. G. Bhusnure, S. S. Mokale, Y. S. Nalwar, and Y. B. Vibhute, J. Pharm. Biomed. Sci., 2011, 6, 1.