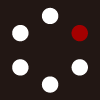
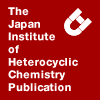
HETEROCYCLES
An International Journal for Reviews and Communications in Heterocyclic ChemistryWeb Edition ISSN: 1881-0942
Published online by The Japan Institute of Heterocyclic Chemistry
e-Journal
Full Text HTML
Received, 29th June, 2015, Accepted, 17th September, 2015, Published online, 24th September, 2015.
■ Nano-Copper Catalyzed Three-Component Tandem Cycloaddition and N-Alkylation Reaction from Aminophenylacetylenes, Sodium Azide and Alkyl Halides
Fangli Gang, Taosheng Dong, Guangli Xu, Ying Fu, and Zhengyin Du*
College of Chemistry and Chemical Engineering, Northwest Normal University, Anning East Road, 967, 730070, China
Abstract
Nano-copper catalyzed one-pot three-component method is realized from aminophenylacetylenes, sodium azide and alkyl halides via tandem 3+2 dipolar cycloaddition reaction and N-alkylation reaction. This synthetic process gave the product of 1-alkyl-4-(N-alkylaminophenyl)-1,2,3-triazoles in good yields.1,2,3-Triazoles are useful structural elements and have been widely used in pharmaceuticals, pesticides, materials and organic synthesis.1 Thus, synthesis of 1,2,3-triazole derivatives is still an attractive project. After Cu-catalyzed azide-alkyne cycloaddition was found, it has been fully developed.2 Different types of catalysts like nano-copper,3 CuI,4 CuFe2O4 nanoparticles,5 Cu2O,6 CuSO47 can all efficiently catalyze this reaction. On the other hand, 1,2,3-triazole as a substrate to construct other derivatives has gradually attracted lots of interest, and this transformation was usually achieved by C—H functionalization8 and new C—N bond formation.9 However, some protocols needs noble metals, special reagents, water- and oxygen-free operation and multistep reactions, which will cause the cost increase and operation inconvenience.
As we all know, multicomponent reaction (MCR), as an environmentally friendly synthetic method, has many advantages for the synthesis of some structure-complex compounds.7d,10 With our continuous pursue of green chemistry on MCR11 and PEG solvent,12 we recently found that 4-methylbenzyl chloride not only participated the formation of the corresponding 1,4-disubstituted 1,2,3-triazole, but also occurred N,N-dialkylation of amino group on benzene ring of 3-aminophenylacetylene in the three-component reaction with sodium azide.3a
The focus of this work is to develop a facile and highly efficient protocol for the synthesis of 1-alkyl-4-(N-alkylaminophenyl)-1H-1,2,3-triazoles by one-pot, three-component tandem reaction from aminophenylacetylene, sodium azide and alkyl halides.
Our initial investigation focused on the reaction of 3-aminophenylacetylene, sodium azide and p-methylbenzyl bromide as a template to optimize the conditions. The results are outlined in Table 1. Several copper compounds, copper powder and copper nanoparticles were firstly examined as catalysts of this reaction at room temperature in air. It is shown that when CuBr, CuCl2 and CuSO4·5H2O were used as catalysts, the primary products were not expected 1-alkyl-4-(N-alkylaminophenyl)-1H-1,2,3-triazoles, but only 4-(3-aminophenyl)-1-(4-methylbenzyl)-1H-1,2,3-triazole with yields of 58%, 65% and 70%, respectively (Table 1, entries 1, 3 and 4). However, the reaction can be smoothly realized under the catalysis of CuI, CuO and Cu powder with low to moderate yields (Table 1, entries 2, 5 and 6). When the same amount of copper nano particles (Cu NPs)3 was used, it is surprised to find that the yield of 4-(3-(N,N-di(4-methylbenzyl)amino)phenyl)-1-(4-methylbenzyl)-1H-1,2,3-triazole as our target product can reach up to 87% (Table 1, entry 7). Then the amount of the catalyst was also examined. When the amount of Cu NPs was reduced from 10 mol% to 5 mol%, it is found that the yield of the product decreased significantly from 87% to 65%, and the yield was still not significantly improved even if the reaction time was prolonged to 24 hours (Table 1, entries 7-9). The effect of a base on this reaction was further explored. However the addition of K2CO3 just increased the yield slightly (Table 1, entry 10). So a base-free process with 10 mol% of Cu NPs as catalyst is determined. When decreasing the amount of 4-methylbenzyl bromide to 1.2 equivalent, the unique cycloaddition product was obtained; decresing the amount of 4-methylbenzyl bromide from 3.6 to 2.4 equivalents, the mixture of cycloaddition product and N,N-dialkylated product was obtained. Furthermore, the yield of N,N-dialkylated product increased and the yield of cycloaddition product decreased with the increase of the amount of 4-methylbenzyl bromide (Table 1, entries 11 and 12). Therefore it is a tandem cycloaddition-N-alkylation reaction.
With the optimum conditions in hand (Table 1, entry 7), the substrate scope was further extended to a broad variety of alkyl halides reacted with 3-aminophenylacetylene and NaN3. The results are listed in Table 2. It is found that various substitutions on benzene ring of benzyl bromides were all well tolerated in this reaction with good product yields. When benzyl bromide was used, the yield of N,N-dibenzyltriazole is 81% (Table 2, 1h). However, when methyl was attached to benzyl bromide, the yields of corresponding N,N-dibenzyltriazoles increased to 87% and 85% in 6 hours for 4-methyl and 3-methylbenzyl bromide, respectively (Table 2, 1a and 1b). Compared with methyl, an electron-donating group, electron-withdrawing groups, such as Br, F, nitro and CN, provided lower yields (Table 2, 1c-f). Different from other substituted benzyl bromides, 4-fluorobenzyl bromide generated N-monosubstituted product in the yield of 77% (Table 2, 1d). When 4-methylbenzyl chloride was subjected to this reaction, the yield of N,N-disubstituted triazole decreased to 52% (Table 2, 1a, X=Cl). It may be due to low reactivity of 4-methylbenzyl chloride. Chain bromoalkanes were also investigated. It is found that 1-bromopropane, 1-bromobutane, 1-bromohexane and 1-bromooctane can complete the reaction smoothly to form corresponding N-alkylation monosubstituted products in good yields (Table 2, 1h-k). We think the reason of no formation of the tertiary amines is the low reactivity of chain alkyl bromide than that of benzyl bromide. Moreover, it is manifest that the reactivity of the brominated chain alkanes decreases with the chain lengthening. Iodobenzene was also used instead of alkyl halides to get the expected corresponding triarylamine, but only was 4-(3-aminophenyl)-1H-1,2,3-triazole obtained.
Subsequently, we replaced 3-aminophenylacetylene with 4-aminophenylacetylene and 2- aminophenylacetylene, and made the following expansion. Similar results were obtained and summarized in Table 3. Different substituents on the benzene ring of benzyl halides can be well compatible with this reaction to provide good yields in the range of 70%-86% (Table 3, 2a-e).
The reaction of 3-methylbenzyl chloride required longer reaction time to get only 56% yield under the same conditions (Table 3, 2e). 1-Brompropane and 1-bromooctane were also involved in this reaction and the corresponding N-monoalkyl substituted products were obtained in the yield of 76% and 65%, respectively (Table 3, 2f and 2g). Unfortunately, when 2-aminophenylacetylene is subjected to this reaction, only 4-cyanobenzyl bromide react with it in the presence of sodium azide to afford corresponding N,N-dibenzyltriazole after 24 hours (Table 3, 3a). Other halogenated hydrocarbons, such as 4-methylbenzyl bromide, 2-bromobenzyl bromide, 4-nitrobenzyl bromide, 4-fluorobenzyl bromide and 1-bromobutane, can occur only Huisgen-cycloaddition reaction and can not obtain expected N-alkylated products, which may be mainly due to large steric hindrance of 2-aminophenylacetylene.
The possible reaction pathway is studied by some control experiments. The Huisgen-cycloaddition product of 3-aminophenylacetylene, 4-methylbenzyl bromide and NaN3 was treated with 2 equivalents of 4-methylbenzyl bromide in methanol at room temperature. The results show that the N-alkylation did not occur without catalytic Cu NPs whereas the yield of N,N-dialkylated product was up to 91% in the presence of 10 mol% Cu NPs (Scheme 1). Obviously, Cu NPs play a catalytic role in Huisgen- cycloaddition as well as in the subsequent N-alkylation. The detailed mechanism is under investigation.
In summary, a tandem one-pot, three-component protocol from aminophenylacetylenes, alkyl halides, and sodium azide to afford N-alkylated 1,4-substituted 1,2,3-triazoles is developed. The process includes one cycloaddition and two or three N-alkylation reactions occurred simultaneously without using any additional reagents except for copper nanoparticles as a catalyst in methanol. The reaction showed better atomic utilization and good regioselectivity.
EXPERIMENTAL
Typical Procedure for Cu NPs.3a CuSO4·5H2O (0.01M) was mixed with 3 mL ethylene glycol (EG) solution of NaOH (0.005 M) and N2H4·H2O (0.015M) under magnetic stirring. After reacted under microwave irradiation at 616 W for 5 min, the mixture turned from blue to brick red. Then cooling to room temperature, distilled water was added to the solution, filtered then the resulting solid was repeatedly washed with distilled water, EtOH and acetone. The resulting solid was placed in a vacuum oven and dried at 60 °C for 3 h and used directly. SEM micrograph showed that the average dimension of Cu NPs was around 18–23 nm.
General procedure for the synthesis of N-alkylated 1,4-disubstituted 1,2,3-triazoles. Sodium azide (0.3 mmol), benzyl halides (0.9 mmol), and aminophenylacetylene (0.25 mmol) were added into a suspension of 0.025 mmol of Cu NPs in MeOH (1 mL). The reaction mixture was stirred at room temperature for completion monitored by TLC. Then the solution was filtered by suction and the solvent was evaporated under reduced pressure. The residue was passed through flash column chromatography (EtOAc/ hexane=1/10) on silica gel to give the pure products.
4-(3-(N,N-Di(3-methylbenzyl)amino)phenyl)-1-(3-methylbenzyl)-1H-1,2,3-triazole (1b): Yield: 85%, yellow solid, mp 135-137 °C. 1H NMR (400 MHz, CDCl3): δ 7.57 (s, 1H), 7.51 (s, 1H), 7.27-7.09 (m, 14H), 6.72 (d, J=7.1, 1H), 5.48(s, 2H), 4.68 (s, 4H), 2.34 (s, 9H). 13C NMR (100 MHz, CDCl3): δ 149.6, 148.6, 138.7, 138.3, 138.1, 134.6, 131.3, 129.5, 129.2, 128.8, 128.5, 128.4, 127.5, 127.3, 124.9, 123.7, 119.5, 114.2, 112.3, 109.4, 53.9, 53.8, 21.3. HRMS (ESI) calcd for C32H32N4 (M + H)+: 473.2627; found 473.2700. IR (KBr, cm-1): 690, 806, 889, 1086, 1184, 1380, 1459, 1496, 1608, 2851, 2918, 3020.
4-(4-(N,N-Di(3-methylbenzyl)amino)phenyl)-1-(3-methylbenzyl)-1H-1,2,3-triazole (2a): Yield: 86%, yellow solid, mp 133-134 °C. 1H NMR (400 MHz, CDCl3): δ 7.49 (s, 1H), 7.31 (s, 1H), 7.25 (s, 1H), 7.17-7.09 (m, 12H), 7.05(s, 1H), 6.68 (s, 1H), 5.47 (s, 2H), 4.61 (s, 4H), 2.33 (d, J = 19.6 Hz, 9H). 13C NMR (100 MHz, CDCl3): δ 149.6, 148.7, 138.5, 136.4, 135.3, 131.7, 131.4, 129.7, 129.6, 129.2, 128.0, 126.7, 119.4, 114.2, 53.9, 53.5, 21.0. HRMS (ESI) calcd for C29H23Br3N4 (M + H)+: 473.2627; found 473.2962. IR (KBr, cm-1): 690, 749, 804, 1048, 1218, 1348, 1452, 1491, 1581, 2851, 2918, 3042.
ACKNOWLEDGEMENTS
We gratefully acknowledge the Natural Science Foundation of China (20702042, 21262028), the Program for Changjiang Scholars and Innovative Research Teams in Universities of the Ministry of Education of China (IRT1177) and the Natural Science Foundation of Gansu Province (1208RJZA140) for financial support.
References
1. (a) R. Hanselmann, G. E. Job, G. Johnson, R. L. Lou, J. G. Martynow, and M. M. Reeve, Org. Process Res. Dev., 2010, 14, 152; CrossRef (b) S. K. Mamidyala and M. G. Finn, Chem. Soc. Rev., 2010, 39, 1252; CrossRef (c) D. I. Rozkiewicz, D. Janczewski, W. Verboom, B. J. Ravoo, and D. N. Reinhoudt, Angew. Chem. Int. Ed., 2006, 45, 5292. CrossRef
2. (a) P. Appukkuttan, V. P. Mehta, and E. V. V. Der Eycken, Chem. Soc. Rev., 2010, 39, 1467; CrossRef (b) J. E. Moses and A. D. Moorhouse, Chem. Soc. Rev., 2007, 36, 1249; CrossRef (c) J. F. Lutz, Angew. Chem. Int. Ed., 2007, 46, 1018; CrossRef (d) C. W. Tornoe, C. Christensen, and M. Meldal, J. Org. Chem., 2002, 67, 3057; CrossRef (e) V. V. Rostovtsev, L. G. Green, V. V. Fokin, and K. B. Sharpless, Angew. Chem. Int. Ed., 2002, 41, 2596; CrossRef (f) H. C. Kolb, M. G. Finn, and K. B. Sharpless, Angew. Chem. Int. Ed., 2001, 40, 2004; CrossRef (g) L. D. Pacho´n, J. H. van Maarseveen, and G. Rothenberg, Adv. Synth. Catal., 2005, 347, 811; CrossRef (h) F. Himo, T. Lovell, R. Hilgraf, V. V. Rostovtsev, L. Noodles, K. B. Sharpless, and V. V. Fokin, J. Am. Chem. Soc., 2005, 127, 210; CrossRef (i) V. O. Rodionov, V. V. Fokin, and M. G. Finn, Angew. Chem. Int. Ed., 2005, 44, 2210. CrossRef
3. (a) L. Huang, W. Liu, J. Wu, Y. Fu, K. Wang, C. Huo, and Z. Du, Tetrahedron Lett., 2014, 55, 2312; CrossRef (b) P. Abdulkin, Y. Moglie, B. R. Knappett, D. A. Jefferson, M. Yus, F. Alonso, and A. E. H. Wheatley, Nanoscale, 2013, 5, 342. CrossRef
4. (a) G. A. Molander and J. Ham, Org. Lett., 2006, 13, 2767; CrossRef (b) F. Ilgen and B. Konig, Green Chem., 2009, 11, 848; CrossRef (c) P. Ji, J. H. Atherton, and M. I. Page, Org. Biomol. Chem., 2012, 10, 7965; CrossRef (d) E. J. Yoo, M. Ahlquist, I. Bae, K. B. Sharpless, V. V. Fokin, and S. Chang, J. Org. Chem., 2008, 73, 5520; CrossRef (e) L. Ackermann, H. K. Potukuchi, D. Landsberg, and R. Vicente, Org. Lett., 2008, 10, 3081; CrossRef (f) Y. Zhao, Z. Yan, and Y. Liang, Tetrahedron Lett., 2006, 47, 1545. CrossRef
5. B. S. P. Anil Kumar, K. H. V. Reddy, B. Madhav, K. Ramesh, and Y. V. D. Nageswar, Tetrahedron Lett., 2012, 53, 4595. CrossRef
6. F. Alonso, Y. Moglie, G. Radivoy, and M. Yus, Synlett, 2012, 23, 2179. CrossRef
7. (a) N. Mukherjee, S. Ahammed, S. Bhadra, and B. C. Ranu, Green Chem., 2013, 15, 389; CrossRef (b) A. K. Feldman, B. Colasson, and V. V. Fokin, Org. Lett., 2004, 22, 3897; CrossRef (c) B. Kaboudin, Y. Abedi, and T. Yokomatsub, Org. Biomol. Chem., 2012, 10, 4543; CrossRef (d) C. Tao, X. Cui, J. Li, A. Liu, L. Liu, and Q. Guo, Tetrahedron Lett., 2007, 48, 3525; CrossRef (e) K. Odlo, E. A. Hoydahl, and T. V. Hansen, Tetrahedron Lett., 2007, 48, 2097; CrossRef (f) A. Kolarovi, M. Schnurch, and M. D. Mihovilovic, J. Org. Chem., 2011, 76, 2613. CrossRef
8. (a) F. Gang, G. Xu, T. Dong, L. Yang, and Z. Du, Chin. J. Org. Chem., 2015, 35, 1428; CrossRef (b) G. B. Yan, A. J. Borah, and M. H. Yang, Adv. Synth. Catal., 2014, 356, 2375; CrossRef (c) Q. Tian, H. Ping, and C. Kuang, Org. Biomol. Chem., 2014, 12, 7474; CrossRef (d) K. D. B. Yamajala, M. Patil, and S. Banerjee, J. Org. Chem., 2015, 80, 3003; CrossRef (e) Z. Wang, Q. Tian, X. Yu, and C. Kuang, Adv. Synth. Catal., 2014, 356, 961; CrossRef (f) W. Liu, Y. Li, B. Xu, and C. Kuang, Org. Lett., 2013, 15, 2342; CrossRef (g) L. Ackermann and R. Vicente, Org. Lett., 2009, 11, 4922; CrossRef (h) S. Chuprekov, N. Chernyak, A. S. Dudnik, and V. Gevorgyan, Org. Lett., 2007, 9, 2333. CrossRef
9. (a) I. V. Seregin and V. Gevorgan, Chem. Soc. Rev., 2007, 36, 1173; CrossRef (b) J. Zhu, Y. Kong, F. Lin, B. Wang, Z. Chen, and L. Liu, Eur. J. Org. Chem., 2015, 7, 1507; CrossRef (c) J. Wen, L. Zhu, Q. Bi, Z. Shen, X. Li, Z. Wang, and Z. Chen, Chem. Eur. J., 2014, 20, 974; CrossRef (d) L. Wang, N. Liu, B. Dai, and H. Hu, Eur. J. Org. Chem., 2014, 29, 6493. CrossRef
10. (a) S. Hassan and T. J. J. Müller, Adv. Synth. Catal., 2015, 357, 617; CrossRef (b) Y. Jiang and C. Kuang, Prog. Chem., 2012, 24, 1983.
11. Z. Du, Y. Li, F. Wang, W. Zhou, and J.-X. Wang, Tetrahedron Lett., 2010, 51, 1745. CrossRef
12. (a) Z. Du, W. Zhou, L. Bai, F. Wang, and J.-X. Wang, Synlett, 2011, 369; CrossRef (b) Z. Du, W. Zhou, and F Wang, Tetrahedron, 2011, 67, 4914. CrossRef