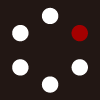
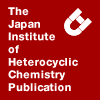
HETEROCYCLES
An International Journal for Reviews and Communications in Heterocyclic ChemistryWeb Edition ISSN: 1881-0942
Published online by The Japan Institute of Heterocyclic Chemistry
e-Journal
Full Text HTML
Received, 23rd August, 2015, Accepted, 9th October, 2015, Published online, 23rd October, 2015.
DOI: 10.3987/COM-15-13309
■ Synthesis and Transformations of 2-Oxo-2,3-dihydro-(1H,3H)-quino[4,3-e]-1,2,4-thiadiazine 4,4-Dioxide to N-Methyl-, 2-Chloro- and 2-Aminoquino[4,3-e]-1,2,4-thiadiazine 4,4-Dioxides
Elwira Chrobak,* Stanisław Boryczka, Michał Wlekliński, Joachim Kusz, Maciej Zubko, and Andrzej Maślankiewicz
Department of Organic Chemistry, The Medical University of Silesia, Jagiellońska 4, 41-200 Sosnowiec, Poland
Abstract
Fusion of 4-amino-3-quinolinesulfonamide (1a) with urea and N-methyl- and N,N’-dimethylurea resulted in 2-oxo-2,3-dihydro-(1H,3H)- quino[4,3-e]-1,2,4-thiadiazine 4,4-oxide (3a). Reaction of thiadiazinone 3a with a MeI/MeOK/DMF system gave a mixture of the 3-methyl derivative 3c (major product) and 6-methyl derivative 3d (minor product), and the mixture of 3c and 3d could be methylated with the same system as above to afford the 3,6-dimethyl derivative 3e. Chlorination of 3a with P(O)Cl3/phosphoric acid system performed in the presence of pyridine hydrochloride resulted in zwitterionic pyridinio-quinothiadiazinate 7 (63%) accompanied by 2-chloroquinothiadiazine 6 (15%), and the same reaction performed with the use of triethylamine hydrochloride gave the expected chloroquinothiadiazine 6 (73%). Chloro derivative 6 was then aminated to 2-aminoquinothiadiazines 8a,b,c.INTRODUCTION
The well-established pharmacological activity of areno and heteroareno fused 1,2,4-thiadiazine S,S-dioxides has induced continuous interest in studies on synthesis and properties of these compounds.1-9
The biological activities of the abovementioned compounds as potassium channel openers,3,8,10 AMPA potentiators,11 cytotoxic agents,2 and anti-HIV agents attracted researchers’ attention as possible candidates for application in the therapy of hypertension,3,8,10 diabetes,10 mental disorders and neurodegenerative diseases,11 cancers2 and viral infections.12
Several papers have shown13-20 that fusion of o-aminoarene- and aminoheteroarenesulfonamide with urea provides easy access to the respective 1,2,4-thiadiazin-3-one S,S-dioxides (Scheme 1, formula B). However, the molecules of the most active 3-amino-1,2,4-thiadiazine S,S-dioxide derivatives contain an endocyclic guanidine fragment incorporated in the 1,2,4-thiadiazine ring. An attempt at direct transformation of 1,2,4-thiadiazin-3-one S,S-dioxides B to 3-aminothiadiazines F using an RNH2/(R’)3N/P2O5 system, however, gave low yields of the expected compounds F.7 Therefore, transformation of the 3-oxo-function of the thiadiazinone ring of B into amino compounds F is usually performed via compounds D or E bearing a leaving group in the 3thiadiazinic position. Thiation of thiadiazinone B with P2S5 leading to thiadiazinethione C followed by methylation of C to methylthio derivative D3,8,14-17 or chlorination of derivatives B to chlorothiadiazine E6,7 were performed for this purpose. The latter route, successfully performed for thieno-1,2,4-thiadiazine derivatives,6 inspired our study on 2-oxo-2,3-dihydro-(1H, 3H)-quino[4,3-e]-1,2,4-thiadiazine 4,4-dioxide (3a).
RESULTS AND DISCUSSION
Fusion of o-aminobenzenesulfonamides or o-aminopyridinesulfonamides with urea (1-4 molar equiv) led to the respective 1,2,4-thiadiazin-3-one S,S-dioxides of type B13-20 (Scheme 1). This process was usually carried out at a temperature of about 180 °C (160-200 °C) up to the solidification of the “melt” and proceeded with intensive gaseous substance evolution. The mixture (cold) was triturated with water to give the ammonium salt of the respective 1,2,4-thiadiazin-3-one S,S-dioxide. The salt (or the solid reaction product) was decomposed with aqueous alkali followed by acidification to pH ~2 to precipitate thiadiazin-3-one B. Similar treatment of 4-amino-3-quinolinesulfonamide (1a) with urea gave the title 2-oxo-2,3-dihydro-(1H,3H)-quino[4,3-e]-1,2,4-thiadiazine 4,4-dioxide (3a) (Scheme 2). As 1-methyl and 3-methyl derivatives 3b or 3c may be of interest as pharmacologically active compounds, 4-amino-3-quinolinesulfonamide (1a) was subjected to fusion with N-methyl- and N,N’-dimethylurea (2b, 2c). However, the reaction gave in all cases studied the parent quinothiadiazin-2-one 3a, i.e. the product without methyl groups.
The formation of the same product 3a from fusion of 4-amino-3-quinolinesulfonamide (1a) with any of urea, N-methylurea or N,N’-dimethylurea (2a-c) strongly suggests that the ureas mentioned above supply the same structural fragment in the course of formation of the thiadiazine ring of 3a. This prompted us to consider reaction pathways leading from 1a and 2a-c to 3a.
It is well-known that urea derivatives with at least one N-H bond undergo thermal decomposition to isocyanic acid or isocyanate and amine or ammonia: RNH(CO)NR1R2→RNCO + HNR1R2.21 The same conclusions were drawn from a more detailed study on methylureas by Chen and Isa.22 Thus, decomposition of urea and N-methylurea should lead to isocyanic acid, but that of N,N’-dimethylurea should give methyl isocyanate.
Both amino groups of aminosulfonamide 1a may react with isocyanic acid derivatives. However, as shown with electronic formulae (Scheme 2), the 4-amino group is deactivated as electron donor – but the highly nucleophilic endocyclic nitrogen atom of 4-aminoquinoline 1a may extract a pseudoacidic proton from sulfonamide group to form sulfonamidate betaine H.23 Thus, as shown in Scheme 2, both isocyanic acid derivatives should carbamoylate aminosulfonamide 1a at sulfonamide NH2 group to form sulfonyl isoureas J. This assumption falls in agreement with thiocarbamoylation of o-aminobenzenesulfonamides by alkyl isothiocyanates at sulfonamide NH2 group.24 Following Chen and Isa’s pathways of thermal decomposition of ureas,22 J would rearrange to tautomers K. Decomposition of K would in both cases (R=H and R=Me) lead to 3-quinolinesulfonyl isocyanate L, which finally undergoes cyclocondensation to 3a. The above consideration led us to the conclusion that urea, N-methylurea or N,N’-dimethylurea (2a-c) supply the same divalent –C(=O)– structural fragment in the course of formation of the thiadiazine ring of 3a.
Hydrolysis of 2-methoxyquinothiazine dioxide 4 (boiling azeotropic HClaq) led to quinothiadiazinone 3a, but the same treatment of 2-methoxy-6-methylquinothiadiazine 5a led to a non-homogenous mixture containing, as concluded from TLC and the 1H NMR spectra, the expected 6-methyl derivative 3d. On the other hand, hydrolysis of 2-chloro-6-methylquinothiadiazine 5b with a DMSO/H2O/HCl system allowed us to obtain individual 6-methylquinothiadiazinone 3d.
N-Methylation
In the search for biologically active compounds, areno- and heteroareno-fused 1,2,4-thiadiazine S,S-dioxides were modified by alkylation at N or O heteroatoms.5,25-27 Several papers deal with the alkylation of areno and heteroareno-fused 1,2,4-thiadiazin-3-one S,S-dioxides5,25-27 (see Scheme 4). For the quino[4,3-e]-1,2,4-thiadiazine 4,4-dioxides studied by our group, methylation of sodium (or potassium) salts of 2-methyl- and 2-methoxy-(1H)-quino[4,3-e]-1,2,4-thiadiazine 4,4-dioxide (9) and (4), and 2-dimethylamino-(6H)-quino[4,3-e]-1,2,4-thiadiazine 4,4-dioxide (8b) proceeded at the endocyclic nitrogen of the pyridine ring and led to the 6-methyl derivatives.26,27
Taking the above into consideration, one would expect that alkylation of quinothiadiazinone 3a may follow at four heteroatoms, as indicated in Scheme 5.
However, treatment of the potassium salt of thiadiazinone 3a (DMF solution, rt) with 1 molar equiv. of methyl iodide proceeded mainly at nitrogen N3 to give 3-methyl-quinothiadiazinone 3c (62%), accompanied by 6-methyl isomer 3d (17%). Methylation of the mixture of 3c and 3d with the same system led to the 3,6-dimethyl derivative 3e (68%).
Alkylation of sodium salts of quinothiadiazinone 3a may formally proceed through nitrogen anionic forms I and IV (Scheme 6). In fact, formation of 3c demonstrates the occurrence of anion IV. On the other hand, the formation of 3d is in agreement with the previous observation that anionic forms derived from 1-NH quinothiadiazines are alkylated at the pyridine ring nitrogen26,27 (see Scheme 4).
The structure of 3c-e and the positions of the newly-introduced N-CH3 groups were concluded from NOE, HSQC and HMBC experiments as presented in Scheme 7.
Irradiation of the N-CH3 proton signal of 3c (δ = 3.69 ppm) did not affect any C-H or N-H proton signal. This means that the NCH3 - CH or -NH proton distances are too far to fulfill the steric requirement for the occurrence of NOE (~4 Å)28 and therefore it suggests that the NCH3 group should be located at position 3. In fact, as deduced from X-ray diffraction analysis of the 3c monocrystal (Figure 1), the N-H proton/NCH3 protons distances are 5.004 Å, 4.362 Å and 4.626 Å. On the other hand, due to the short H-10 proton-N1-H proton distance (2.09 Å), irradiation of the H-10 proton (δ = 8.76 ppm) led to enhancement of the N1-H proton signal (bs, δ = 12.2 ppm) by 9.6%. The structures of the 6-methyl derivative 3d and the 3,6-dimethyl derivative 3e were demonstrated by HSQC and HMBC experiments (Scheme 7). Additionally, the structure of 3d was confirmed by the hydrolysis of 2-metoxyquinothiadiazine 5a and 2-chloroquinothiadiazine 5b (see Scheme 3).
Chlorination
As mentioned above, the transformation of the oxo function at thiadiazine ring of compounds B (Scheme 1) into a more active leaving group is necessary for further functionalization of areno and heteroareno-fused 1,2,4-thiadiazine S,S-dioxides B, as a first step toward the preparation of biologically active compounds.
For this purpose, the lactam fragment of oxo derivative 3a was subjected to deoxo-chlorination. Several chlorination reagents or systems were tested. Ineffective reactions occurred with P(O)Cl3, PhOP(O)Cl2 and with the P(O)Cl3/Et3N x HCl system (120-150 oC, 4-8 h). Reaction of 3a with the PhOP(O)Cl2/Et3N x HCl system (150 oC, 4 h) led to ~90% unreacted substrate 3a accompanied by a small amount of the desired 2-chlorothiadiazine 6. Following the Hansen group procedure,7 thiadiazinone 3a was then subjected to chlorination with a P(O)Cl3/pyridine hydrochloride/phosphoric acid system and the expected chlorothiadiazine 6 was isolated in 15% yield, although the main product appeared to be zwitterionic pyridinio-quinothiadiazinate 7 (63%). Formation of 7 falls in agreement with literature data concerning the formation of pyridinio salts or pyridinio zwitterionic species (with 1,3-diazine motif) in the reactions of pyrimidinones or 2- and 6-purinones with PhOP(O)Cl2 or P(O)Cl3 in the presence of pyridine.29 The structure of 7 was assigned a negatively charged N3 nitrogen atom due to stabilization of the negative charge by strong electron withdrawing sulfonyl group.30
Since an increase in steric hindrance at the nucleophilic nitrogen of a tertiary amine should lead to a reduction in the formation of aminium species, we tried to replace the pyridine hydrochloride with triethylamine hydrochloride. In fact, the reaction of 3a with a P(O)Cl3/triethylamine hydrochloride/85% phosphoric acid system afforded 2-chloroquino[4,3-e]-1,2,4-thiadiazine 4,4-dioxide (6) in a good yield of 75%.
Finally, reactions of chlorothiadiazine 6 with amines led to aminothiadiazines 8a,b,c.
CONCLUSIONS
Although areno and heteroareno-fused 1,2,4-thiadiazine S,S-dioxides may be easily obtained by the fusion of o-aminoarene- and aminoheteroarenesulfonamide with urea, the most important fused 1,2,4-thiadiazine S,S-dioxides may only be reached in complex multi-step syntheses.
Our study extends previous findings concerning heteroarene-fused 1,2,4-thiadiazine S,S-dioxides, showing that alkylation of sodium salts of quinothiadiazinone 3a proceeds mainly at the sulfonamide nitrogen of the thiadiazine ring and to a lesser extent at the pyridine ring nitrogen, i.e. outside the thiadiazine ring.
The most valuable results were obtained through functionalization of quinothiadiazinone 3a to 2-chloroquinothiadiazine 6 in a deoxo-chlorination reaction performed with the use of a P(O)Cl3/phosphoric acid system in the presence of triethylamine hydrochloride. Chloro derivative 6 was a convenient substrate for the synthesis of 2-aminoquinothiadiazines 8a,b,c.
EXPERIMENTAL
Melting points were measured in open capillary tubes and are uncorrected. All NMR spectra were recorded on a Bruker AVANCE 400 spectrometer operating at 400.22 MHz and 100.64 MHz for 1H and 13C nuclei, respectively, in deuterochloroform or in hexadeuterodimethyl sulfoxide solutions with tetramethylsilane (δ 0.0 ppm) as the internal standard. Two-dimensional 1H-13C HSQC and HMBC experiments were performed using standard Bruker software HSQCGP and HMBCGP, respectively, and the following parameters: the spectral widths in F2 and F1 were ca. 5 kHz for 1H and 16.7 kHz for 13C, the relaxation delay was 1.5 s, the refocusing in the HSQC experiment was 1.7 ms and the delay for long-range evolutions was 50 ms in 1H/13C HMBC. 2D spectra were acquired as 2048 x 1024 hypercomplex files, with 1-4 transients. EI MS spectra were determined on a Finnigan MAT 95 spectrometer at 70 eV. IR spectra were recorded with a Magma – IR 500 (Nicolet) spectrometer in potassium bromide pellets. TLC analyses were performed employing Merck’s aluminum oxide 60 F254 neutral (type E) plates using chloroform as the eluent.
4-Amino-3-quinolinesulfonamide (1a),31 2-methoxy- and 2-chloro-6-methyl-(6H)-quino[4,3-e]- 1,2,4-thiadiazine 4,4-dioxides (5a and 5b)26 were prepared as described previously.
N-Methyl- and N,N’-dimethylureas were commercial products.
Fusion of 4-amino-3-quinolinesulfonamide (1a) with ureas
4-Amino-3-quinolinesulfonamide (1a) (0.9 g, 4 mmol) and urea (0.6 g, 10 mmol) were mixed together, powdered and then heated at 200 °C (oil bath temperature). The reaction proceeded with strong evolution of gases and the formation of a clear solution, which solidified after 30 min. After cooling, the mixture was powdered, dissolved in water and filtered to remove small amounts of insoluble material. The filtrate was acidified with concentrated HCl to pH 2. The precipitate of 3a was collected by filtration, washed with water and dried. For purification, crude 3a was boiled with EtOH (18 mL) to yield 2-oxo-1,3-dihydro(1H, 3H)-quino[4,3-e]-1,2,4-thiadiazine 4,4-dioxide (3a) (0.88 g, 88%).
Reactions of 1a (0.89 g, 4 mmol) with N-methylurea (4-8 mmol) were performed in the same manner and gave 3a (0.7-0.8 g, 70-80%).
Reactions of 1a (0.89 g, 4 mmol) with N,N’-dimethylurea (4-8 mmol) were performed in the same manner for 1 h. After cooling, the mixture was powdered, dissolved in water and filtered to recover a small amount (0.075 g; 8%) of insoluble substrate 1a. The filtrate was acidified with concentrated HCl to pH 2. The precipitate was collected by filtration, washed with water and dried. It consisted mainly of thiadiazinone 3a and substrate 1a, as deduced from TLC and 1H NMR data. 1H NMR spectrum showed the ratio 1a:3a=2:5, based on the intensities of singlets of α-quinolinyl proton (δ = 8.92 ppm for 1a) and H-5 proton (δ = 9.25 ppm for 3a). The crude product (~0.850 g) was recrystallized from 20 mL of DMF to give 0.530 g (53%) of 3a. The filtrate was concentrated vacuo at 80 °C to 1/4 volume and cooled to rt, and the solid deposited was separated by filtration to give 0.080 g (9%) of substrate 1a.
2-Oxo-2,3-dihydro-(1H, 3H)-quino-[4,3-e]-1,2,4-thiadiazine 4,4-dioxide (3a)
mp 324-326 oC (decomp.). EIMS (70 eV): m/z (%) = 249 (100, M+). 1H NMR (DMSO-d6), δ: 7.80-7.83 (m, 1H, Harom), 8.01-8.09 (m, 1H, Harom), 8.09-8.10 (m, 1H, Harom), 8.86-8.88 (m, 1H, Harom), 9.25 (s, 1H, H-5), 11.52 (bs, 1H, NH), 13.8 (bs, 1H, NH). 13C NMR (DMSO-d6), δ: 114.1, 116.9, 124.3, 124.4, 128.6, 134.1, 141.9, 142.0, 146.4, 151.5. Anal. Calcd for C10H7N3O3S: C 48.19, H 2.83, N 16.86. Found: C 48.03, H 3.15, N 16.65.
Hydrolysis of 2-methoxy-(1H)-quino[4,3-e]-1,2,4-thiadiazine 4,4-dioxide (4)
A mixture of 2-methoxy-(1H)-quino[4,3-e]-1,2,4-thiadiazine 4,4-dioxide (4) (0.2 g, 0.76 mmol) and 4 mL of hydrochloric acid (1:1) was heated at 100 °C (oil bath temperature) for 30 min with occasional shaking. The mixture was clarified after 5 min. It was then diluted with 4 mL of water and cooled to rt. The precipitate of 3a was collected by filtration, washed with a few drops of cold water, and dried to give 0.12 g (64%) of 3a with an mp 317-320 °C and an 1H NMR spectrum identical to that of the authentic material prepared as above.
The filtrate was concentrated in vacuo at 60 °C. The residue was neutralized with 5% aqueous NaHCO3. The solid was filtered off, washed with water and dried to give 0.06 g (30%) of unreacted 4 as based on mp, TLC and 1H NMR data.
Hydrolysis of 2-methoxy-6-methyl-(6H)-quino[4,3-e]-1,2,4-thiadiazine 4,4-dioxide (5a)
Hydrolysis of 2-methoxy derivative 5a was performed with hydrochloric acid as described above for compound 4. Both solids were non-homogenous, as concluded from the 1H NMR (DMSO-d6) spectra and TLC data, but the presence of the 6-methyl derivative 3d was observed in a total amount of ~20% (as judged from the 1H NMR spectra).
Hydrolysis of 2-chloro-6-methyl-(6H)-quino[4,3-e]-1,2,4-thiadiazine 4,4-dioxide (5b)
2-Chloro derivative (100 mg, 0.36 mmol), 3 mL of DMSO and one drop of a solution of HCl in DMSO (0.1 mL of 5% HClaq and 0.9 mL of DMSO) were stirred at rt for 32 h. The solution was diluted with 20 mL of cold water. The solid was filtered off and dried in air to give a mixture (80 mg) of substrate 5b and 6-methyl derivative 3d in a ratio of ca. 5:1. The mixture was triturated with 1 mL of 5% aqueous KOH. The insoluble substrate 5b (64 mg) was filtered off and washed with water. The filtrate was acidified with 5% HCl to pH 2 to give 14 mg (~15%) of 3d with the same properties [1H NMR (DMSO-d6) spectra and TLC data] as the sample prepared from the methylation of 3a, as described below.
N-Methylation of 2-oxo-2,3-dihydro-(1H,3H)-quino-[4,3-e]-1,2,4-thiadiazine 4,4-dioxide (3a)
Potassium methoxide (0.290 g, ca. 4.1 mmol) was added with stirring to a suspension of 2-oxo-2,3-dihydro-(1H,3H)-quino-[4,3-e]-1,2,4-thiadiazine 4,4-dioxide (3a) (1 g, 4 mmol) in dry DMF (8 mL). The mixture was stirred for 5-10 min until the mixture became clear. Then, a solution of methyl iodide (1.6 mL, ca. 4 mmol) in DMF (2.6 mL) was added dropwise for 15 min and the mixture was stirred at rt for 20 h. The solid was filtered off, washed with cold water and dried on air to give a mixture (0.87 g) of N-methylquinothiadiazinones 3c and 3d. It was boiled for 5 min with DMF (50 mL), cooled to rt and filtered off to give 3d (0.18 g, 17%). The filtrate was concentrated in vacuo at 80 °C to 1/3 volume, cooled to rt and filtered off to give 3c (0.65 g, 62%).
A mixture of 3c and 3d (ratio 1 : 0.27, 0.53 g, 2.0 mmol) was methylated as above with potassium methoxide (0.145 g, ca. 2.05 mmol) and a solution of methyl iodide (0.8 mL, ca. 2 mmol) in DMF (1.3 mL) to give 3,6-dimethyl-2-oxo-2,3-dihydro-(3H,6H)-quino-[4,3-e]-1,2,4-thiadiazine 4,4-dioxide (3e) (0.38 g, 68%).
3-Methyl-2-oxo-2,3-dihydro-(1H,3H)-quino-[4,3-e]-1,2,4-thiadiazine 4,4-dioxide (3c)
mp 293-296 oC (CHCl3/EtOH). EIMS (70 eV): m/z (%) = 263 (100, M+). 1H NMR (DMSO-d6), δ [δ C for carbons from single bond and / long range proton-carbon correlations]: 3.30 [(s, 3H, CH3N3); 26.5 (CH3N3) / 150.0 (C2)], 7.78 [(m, 1H, H9); 128.6 (C9) / 116.5 (C10a); 130.2 (C7)], 7.98 [(m, 1H, H8); 133.4 (C8) / 123.8 (C10); 149.6 (C6a)], 8.10 [(d, 1H, H7); 130.2 (C7) / 116.5 (C10a); 128.6 (C9)], 8.74 [(d, 1H, H10); 123.8 (C10) / 133.4 (C8); 140.0 (C10b); 149.6 (C6a)], 9.12 [(s, 1H, H5); 142.5 (C5) / 113.2 (C4a); 140.0 (C10b); 149.6 (C6a)], 12.2 (br s, 1H, NH). Anal. Calcd for C11H9N3O3S: C 50.18, H 3.45, N 15.96. Found: C 49.97, H 3.48, N 15.89.
NOE experiments: irradiation of the H-10 proton (δ = 8.76 ppm) led to enhancement of the NH proton signal (bs, δ = 12.2 ppm) by 9.6%; irradiation of the N-CH3 protons signal (δ = 3.69 ppm) did not affect any C-H or N-H proton signal, irradiation of the H7 proton signal (δ = 8.12 ppm) and H10 proton signal (δ = 8,76 ppm) (δ = 3.69 ppm) did not affect CH3 protons signal.
6-Methyl-2-oxo-2,3-dihydro-(3H,6H)-quino-[4,3-e]-1,2,4-thiadiazine 4,4-dioxide (3d)
mp 297-300 oC (DMF). EIMS (70 eV): m/z (%) = 263 (100, M+). 1H NMR (DMSO-d6), δ [δ C for carbons from single bond and / long range proton-carbon correlations]: 4.38 [(s, 3H, CH3N); 43.8 (CH3N) / 145.4 (C5); 139.3 (C6a)], 7.94 [(m, 1H, H9); 129.2 (C9) / 117.6 (C10a); 119.9 (C7)], 8.19 [(m, 1H, H8); 135.6 (C8) / 125.3 (C10); 139.3 (C6a)], 8.29 [(m, 1H, H7); 119.9 (C7) / 117.6 (C10a); 129.2 (C9)], 9.01 [(m, 1H, H10); 125.3 (C10) / 135.6 (C8); 139.3 (C6a); 148.2 (C10b)], 9.55 [(s, 1H, H5); 145.4 (C5) / 114.0 (C4a); 139.3 (C6a); 148.2 (C10b)], 11.69 [(br s, 1H, N3-H) / 114.0 (C4a)], no correlation was observed with C2 carbon signal δ C = 150.9 ppm. Anal. Calcd for C11H9N3O3S: C 50.18, H 3.45, N 15.96. Found: C 49.99, H 3.48, N 15.85.
3,6-Dimethyl-2-oxo-2,3-dihydro-(3H,6H)-quino-[4,3-e]-1,2,4-thiadiazine 4,4-dioxide (3e)
mp 279-281 oC (EtOH). EIMS (70 eV): m/z (%) = 277 (54, M+), 183 (100). 1H NMR (DMSO-d6), δ [δ C for carbons from single bond and / long range proton-carbon correlations]: 3.18 [(s, 3H, CH3N3); 25.7 (CH3N3) / 155.4 (C2)], 4.17 [(s, 3H, CH3N6); 42.4 (CH3N6) / 138.9 (C6a); 142.8 (C5)], 7.76 [(m, 1H, H9); 127.7 (C9) / 118.4 (C7); 123.9 (C10a); 134.4 (C8); 138.9 (C6a)], 8.02 [(m, 1H, H8); 134.4 (C8) / 127.7 (C9); 138.9 (C6a); 123.9 (C10a)], 8.04 [(m, 1H, H7); 118.4 (C7) / 127.7 (C9); 138.9 (C6a)], 8.67 [(m, 1H, H10); 125.9 (C10) / 134.4 (C8); 138.9 (C6a); 154.8 (C10b)], 9.27 [(s, 1H, H5); 142.8 (C5) / 112 (C4a); 123.9 (C10a); 138.9 (C6a); 154.8 (C10b). Anal. Calcd for C12H11N3O3S: C 51.98, H 4.00, N 15.15. Found: C 51.64, H 3.84, N 15.14.
Chlorination of 2-oxo-2,3-dihydro-(1H,3H)-quino-[4,3-e]-1,2,4-thiadiazine 4,4-dioxide (3a) with P(O)Cl3/ pyridine or triethylamine hydrochlorides /phosphoric acid systems
A mixture of 3a (0.5 g, ca. 2 mmol), phosphorus oxychloride (2.4 mL), pyridine hydrochloride (0.8 g, 7 mmol) and 85% ortho-phosphoric acid (0.14 mL) was stirred at 100 °C for 8 h. The mixture was concentrated in vacuo at 50 °C, and the residual oil was carefully treated with ice water (10 mL) with stirring at 0 °C. The crude product was isolated by filtration, washed with water, and triturated with saturated aqueous NaHCO3 (10 mL). The solid was filtered off, washed with water and dried in air to give pyridinio-quinothiadiazinate 7 (0.44 g, 63%). The filtrate was acidified with 5% HCl with stirring at 0 °C and the white precipitate that formed was filtered, washed with water, and dried to give 0.08 g (15%) of 2-chloro-(1H)-quino-[4,3-e]-1,2,4-thiadiazine 4,4-dioxide (6).
The same reaction performed with the use of triethylamine hydrochloride (0.96 g, 7 mmol), instead of pyridine hydrochloride, gave 0.40 g (74%) of chloroquinothiadiazine 6.
2-Chloro-(1H)-quino-[4,3-e]-1,2,4-thiadiazine 4,4-dioxide (6)
mp >350 oC (dec.). EIMS (70 eV): m/z (%) = 269 [36.8, (M+2)], 267 (100, M+). 1H NMR (DMSO-d6), δ: 7.82-7.86 (m, 1H, Harom), 8.05-8.06 (m, 2H, Harom), 8.63-8.65 (m, 1H, Harom), 9.56 (s, 1H, H-5), no N1- H proton signal was seen (hidden in a broad H2O peak). 13C NMR (DMSO-d6), δ: 114.1, 116.8, 123.0, 124.6, 129.0, 134.9, 140.4, 141.2, 147.3, 151.3. Anal. Calcd for C10H6ClN3O2S: C 44.87, H 2.26, N 15.70. Found: C 44.57, H 2.11, N 15.45.
2-(1-Pyridinio)-(1H)-quino-[4,3-e]-1,2,4-thiadiazinate 4,4-dioxide (7)
mp >306 oC (EtOH). EIMS (70 eV): m/z (%) = 310 (100). 1H NMR (DMSO-d6), δ: 7.71-7.79 (m, 1H, Harom), 7.86-7.95 (m, 1H, Harom), 8.01-8.09 (m, 1H, Harom), 8.22-8.32 (m, 2H, Harom), 8.81-8.87 (m, 1H, Harom), 8.87-8.95 (m, 1H, Harom), 9.10 (s, 1H, H5), 10.12-10.22 (m, 2H, Harom). ). 13C NMR (DMSO-d6), δ: 114.5, 124.0, 125.1, 127.5, 127.9, 128.0, 129.4, 131.7, 141.3, 145.2, 148.8, 149.0, 150.1, 150.7, 155.5. Anal. Calcd for C15H10N4O2S: C 58.06, H 3.22, N 18.06. Found: C 57.79, H 3.19, N 17.70.
Amination of 2-chloro-(1H)-quino-[4,3-e]-1,2,4-thiadiazine 4,4-dioxide (6)
a) dimethylamine
Chloroquinothiadiazine 6 (270 mg, ~1 mmol) and 4 mL of a 40% aqueous Me2NH solution were placed in a steel autoclave. It was heated in an oil bath at 100 ºC for 2 h. The mixture was cooled down to rt, transferred to a distillation flask and the excess Me2NH was then distilled off under vacuum. The solid was filtered off, washed with cold water and boiled with EtOH. Hot solution was decanted off to leave dimethylamino derivative 8a (181 mg, 85%) with mp 313-316 ºC (EtOH); according to ref. 27 the mp 313-316 ºC and 1H NMR spectrum are identical with the sample prepared previously.27
b) with n-butylamine
Chloroquinothiadiazine 6 (270 mg, ~1 mmol) and n-butylamine (4 mL) were refluxed for 1 h. The excess of n-butylamine was then evaporated to dryness under reduced pressure in a water bath. The residue was cooled down to rt and triturated with water (4 mL). The solid was filtered off, washed with cold water and dried in air. The crude product was purified by column chromatography (SiO2, CHCl3/EtOH, 3:1, v/v) and recrystallized from EtOH to give 8b (234 mg, 77%).
c) with aniline
Chloroquinothiadiazine 6 (270 mg, ~1 mmol) and aniline (1.2 mL, 12 mmol) were stirred at 100 oC for 1 h. The mixture was cooled down to rt and triturated with a mixture of CHCl3/EtOH (3:1, v/v) (4 mL). The solid was filtered off and dried in air, and finally recrystallized from EtOH to give 8c (200 mg, 61%).
2-Butylamino-(6H)-quino-[4,3-e]-1,2,4-thiadiazine 4,4-dioxide (8b)
mp 248-250 oC (EtOH). EIMS (70 eV): m/z (%) = 304 (37.4, M+). 1H NMR (DMSO-d6), δ: 0.91-0.95 (m, 3H, CH3), 1.35-1.41 (m, 2H, CH2), 1.52-1.59 (m, 2H, CH2), no NH-CH2 protons signal was seen, hidden in a broad H2O peak, at δ = 3.33 ppm, 7.70-7.78 (m, 2H, Harom), 7.86-7.97 (m, 1H, Harom), 8.47-8.49 (m, 1H, Harom), 8.95 (s, 1H, H5), 8.06 (s, 1H, NH), 11.47 (s, 1H, NH). 13C NMR (DMSO-d6), δ: 14.0, 19.7, 31.1, 40.7, 113.0, 117.8, 123.0, 123.2, 127.8, 129.3, 132.6, 143.1, 143.9, 152.7. Anal. Calcd for C14H16N4O2S: C 55.25, H 5.30, N 18.41. Found: C 54.97, H 5.15, N 18.12.
2-Phenylamino-(6H)-quino-[4,3-e]-1,2,4-thiadiazine 4,4-dioxide (8c)
mp 302-306 oC (EtOH). EIMS (70 eV): m/z (%) = 324 (100, M+). 1H NMR (DMSO-d6), δ: 2.95-3.90 (bs, 1H, NH), 7.03-7.16 (m, 1H, Harom), 7.28-7.45 (m, 2H, Harom), 7.60-7.84 (m, 3H, Harom), 7.86-8.10 (m, 2H, Harom), 8.46-8.75 (m, 1H, Harom), 9.06 (s, 1H, H5), 9.79 (s, 1H, NH). 13C NMR (DMSO-d6), δ: 112.8, 117.9, 121.1, 123.8, 124.1, 124.7, 127.7, 129.1, 129.7, 133.6, 139.3, 140.3, 142.8, 157.0. Anal. Calcd for C16H12N4O2S: C 59.25, H 3.73, N 17.27. Found: C 58.97, H 3.59, N 16.99.
X-Ray structure analysis
The diffraction data were collected with a four – circle Xcalibur diffractometer with Sapphire3 CCD detector using graphite monochromated Mo Kα radiation. The intensity data were collected and processed using Oxford Diffraction CrysAlis Software.32 The crystal structures were solved by direct methods with the program SHELXS-9733 and refined by full-matrix least-squares method on F2 with SHELXL-97.33
Crystals of 2-oxo-2,3-dihydro-3-methyl-(1H,3H)-quino[4,3-e]-1,2,4-thiadiazine 1,1-dioxide (3c) were obtained by slow evaporation of DMF solution at room temperature. Crystal data for 3c: monoclinic, space group P21/c, a = 5.6446(1) Å, b = 20.1260(2) Å, c = 10.1508(1) Å, α = 90º, β = 113.781(1)º, γ = 90º, V = 1055.25 Å3, Z = 4, dx = 1.657 Mg m-3, T = 100(1) K, Data were collected for a crystal of dimensions 0.07 x 0.44 x 0.53 mm3. Final R indices for 1841 reflections with I > 2σ(I) and 184 refined parameters are R1 = 0.0266, wR2 = 0.0693 (R1 = 0.0268, wR2 = 0.0693 for all 1867 data).
Crystallographic data for compound 3c has been deposited with Cambridge Crystallographic Data Centre (CCDC deposition number 1054243) Copies of the data can be obtained upon request from CCDC, 12 Union road, Cambridge CB2 1EZ, UK).
ACKNOWLEDGEMENTS
This work was supported by the Medical University of Silesia in Katowice, Poland. Grant No KNW-1-002/N/5/0.
References
#. Part CXLV in the series of Azinyl Sulfides.
┴. These authors contributed equally to the work.
^. Retired from Medical University of Silesia.
1. Z. Brzozowski, J. Sławiński, M. Gdaniec, A. Innocenti, and C. T. Supuran, Eur. J. Med. Chem., 2011, 46, 4403. CrossRef
2. A. Kamal, Y. V. V. Srikanth, M. N. A. Khan, Md. Ashraf, M. K. Reddy, F. Sultana, T. Kaur, G. Chashoo, N. Suri, I. Sehar, Z. A. Wani, A. Saxena, P. R. Sharma, S. Bhushan, D. M. Mondhe, and A. K. Saxena, Bioorg. Med. Chem., 2011, 19, 7136. CrossRef
3. B. Pirotte, P. de Tullio, Q.-A. Nguyen, F. Somers, P. Fraikin, X. Florence, P. Wahl, J. B. Hansen, and P. Lebrun, J. Med. Chem., 2010, 53, 147. CrossRef
4. P. Lebrun, B. Becker, N. Morel, P. Ghisdal, M.-H. Antoine, P. de Tullio, and B. Pirotte, Biochem. Pharmacol., 2008, 75, 468. CrossRef
5. B. Pirotte, P. de Tullio, S. Boverie, C. Michaux, and P. Lebrun, J. Med. Chem., 2011, 54, 3188. CrossRef
6. F. E. Nielsen, S. Ebdrup, A. Frost Jensen, L. Ynddal, T. B. Bodvarsdottir, C. E. Stidsen, A. Worsaae, H. C. M. Boonen, P. O. G. Arkhammar, T. Fremming, P. Wahl, H. T. Kornǿ, and J. B. Hansen, J. Med. Chem., 2006, 49, 4127. CrossRef
7. F. E. Nielsen, T. B. Bodvarsdottir, A. Worsaae, P. Mackay, C. E. Stidsen, H. C. M. Boonen, L. Pridal, P. O. G. Arkhammar, P. Wahl, L. Ynddal, F. Junager, N. Dragsted, T. M. Tagmose, J. P. Mogesen, A. Koch, S. P. Treppendahl, and J. B. Hansen, J. Med. Chem., 2002, 45, 4171. CrossRef
8. S. Boverie, M. H. Antoine, F. Somers, B. Becker, S. Sebille, R. Ouedraogo, S. Counerotte, B. Pirotte, P. Lebrun, and P. de Tullio, J .Med .Chem., 2005, 48, 3492. CrossRef
9. A. Cherepakha, V. O. Kovtunenko, A. Tolmachev, and O. Lukin, J. Heterocycl. Chem., 2013, 50, 1071. CrossRef
10. B. Ng, Y. Kang, C. L. Elias, Y. He, H. Xie, J. B. Hansen, P. Wahl, and H. Y. Gaisano, Diabetes, 2007, 56, 2124. CrossRef
11. P. Francotte, P. de Tullio, T. Podona, O. Diouf, P. Fraikin, P. Lestage, L. Danober, J. Y. Thomas, D. H. Caignard, and B. Pirotte, Bioorg. Med. Chem., 2008, 16, 9948. CrossRef
12. a) X. Liu, R. Yan, N. G. Chen, and W. F. Xu, Chin. Chem. Lett., 2007, 18, 137; CrossRef b) X. Liu, R. Yan, Y. Wang, P. Zhan, E. DeClerq, C. Pannecouque, M. Witvrouw, M. T. Molina, and S. Vega, Arch. Pharm. Life Sci., 2008, 341, 216. CrossRef
13. L. Raffa, A. Monzani, and A. Albasini, Il Farmaco – Ed. Sc., 1964, XIX, 35.
14. B. Pirotte, P. de Tullio, B. Masereel, J. Delarge, J. Lepagnol, and P. Renard, US Patent, 5 459 138 (Oct. 17, 1995).
15. B. Pirotte, P. de Tullio, P. Lebrun, M. H. Antoine, J. Fontaine, B. Masereel, M. Schynts, L. Dupont, A. Herchuelz, and J. Delarge, J. Med. Chem., 1993, 36, 3211. CrossRef
16. P. de Tullio, B. Pirotte, P. Lebrun, J. Fontaine, L. Dupont, M. H. Antoine, R. Ouedraogo, S. Khelili, C. Maggetto, B. Masereel, O. Diouf , T. Podona, and J. Delarge, J. Med. Chem., 1996, 39, 937. CrossRef
17. C. G. Neill, P. N. Preston, and R. H. Wightman, Tetrahedron, 1998, 54, 13645. CrossRef
18. F. C. Novello, S. C. Bell, E. L. A. Abrams, C. Ziegler, and J. M Sprague, J. Org. Chem., 1960, 25, 970. CrossRef
19. E. Magnien, W. Tom, and W. Oroshnik, J. Med. Chem., 1964, 7, 821. CrossRef
20. S. Kotovskaya, G. Mokrushina, I. Postovskii, E. Pidémskii, A. Goleneva, and T. Vysokova, Khim. Farm. Zh., 1979, 13, 389.
21. F. Hegarty, vol. 2, subchapter 9.10.4.2, ed. by D. Barton and W. D. Ollis, ‘Comprehensive Organic Chemistry’, Pergamon Press, Oxford-New York-Toronto-Sydney-Paris-Frankfurt, 1979.
22. J. P. Chen and K. Isa, J. Mass Spectrom. Soc. Jpn., 1998, 46, 299. CrossRef
23. A species similar to H was observed as torasemide tautomer by A. Danilovski, D. Filić, M. Orešić, and M. Dumić, Croat. Chem. Acta, 2001, 74, 103.
24. P. de Tullio, S. Boverie, B. Becker, M.-H. Antoine, Q.-A. Nguyen, P. Francotte, S. Counerotte, S. Sebille, B. Pirotte, and P. Lebrun, J. Med. Chem., 2005, 48, 4990 – to activate of sulfonamide group, the reaction was performed in the presence of K2CO3/acetone system. CrossRef
25. X. Liu, R. Yan, N. Chen, W. Xu, M. T. Molina, and S. Vega, Molecules, 2006, 11, 827. CrossRef
26. E. Chrobak, M. Wlekliński, A. Maślankiewicz, J. Kusz, M. Zubko, and E. Michalik, Heterocycles, 2011, 83, 1819. CrossRef
27. E. Chrobak, M. Wlekliński, A. Maślankiewicz, J. Kusz, M. Zubko, and A. Zięba, Heterocycles, 2010, 81, 1799. CrossRef
28. D. Neuhaus and M. Williamson, ‘The Nuclear Overhauser Effect In Structural and Conformational Analysis’, VCH Publisher, New York, 1989, pp. 451-502.
29. a) R. W. Adamiak, E. Biała, Z. Gdaniec, S. Mielewczyk, and B. Skalski, Chem. Scr., 1986, 26, 3; b) B. Skalski, G. Wenska, Z. Gdaniec, and R. W. Adamiak, Tetrahedron, 1993, 49, 5859. CrossRef
30. J. Shorter, “Electronic Effects of the Sulfinyl and Sulfonyl Groups”, chapter 10, ed. by S. Patai, Z. Rappoport, and C. Stirling, ‘The Chemistry of Sulphones and Sulphoxides’, John Willey & Sons, Chichester-New York-Brisbane-Toronto-Singapore, 1988, a) p 531, b) pp. 523-524. CrossRef
31. A. Maślankiewicz and L. Skrzypek, Heterocycles, 1994, 38, 1317. CrossRef
32. Oxford Diffraction (2006) CrysAlis CCD and CrysAlis RED. Versions 1.171.32.29. Oxford Diffraction Ltd, Wrocław, Poland.
33. G. M. Sheldrick, Acta Cryst., 2008, A 64, 112. CrossRef