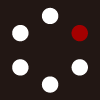
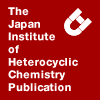
HETEROCYCLES
An International Journal for Reviews and Communications in Heterocyclic ChemistryWeb Edition ISSN: 1881-0942
Published online by The Japan Institute of Heterocyclic Chemistry
e-Journal
Full Text HTML
Received, 16th September, 2015, Accepted, 21st October, 2015, Published online, 28th October, 2015.
DOI: 10.3987/COM-15-13325
■ Synthesis and Antibacterial Activity of Tripropeptin C Derivatives Containing the Pyrimidine Ring System
Sehei Hirosawa, Yoshiaki Takahashi,* Ayumi Murotani, Hideki Hashizume, Toshiaki Miyake, and Yuzuru Akamatsu
Hiyoshi branch, Institute of Microbial Chemistry (BIKAKEN), 3-34-17, Ida Nakahara-ku, Kawasaki, Kanagawa 211-0035, Japan
Abstract
A series of modifications to the terminal guanidine group of the arginine residue in tripropeptin C was performed, and the corresponding pyrimidine derivatives were synthesized as a part of a structure-activity relationship study. Some of the resulting compounds maintained excellent antibacterial activity. Our findings indicated that the guanidine group in tripropeptin C is not essential for the antibacterial activity of this compound, and the possibility of chemical modification of the guanidine group was demonstrated.INTRODUCTION
Antimicrobial resistance is an increasing problem worldwide and presents a major threat to public health.1,2 There is therefore an urgent need for the discovery and development of new antibacterial agents that are effective against multidrug-resistant bacteria, structurally different from existing medicines and exert a novel mode of action.3
During screening of microbial products for novel antibiotics showing activity against drug-resistant bacteria, we discovered a structurally related mixture of tripropeptins4–6 in the cultured cells and broth of the soil bacterium Lysobacter sp. strain BMK333-48F3. The major component of this group of novel cyclic lipodepsipeptide antibiotics, tripropeptin C (TPPC), showed potent antimicrobial activity towards a variety of different Gram-positive pathogens, including methicillin-resistant Staphylococcus aureus (MRSA), vancomycin-resistant Enterococcus faecalis/faecium and penicillin-resistant Streptococcus pneumoniae.4,5,7 TPPC also showed excellent in vivo therapeutic efficacy in a mouse-MRSA septicemia model.7 TPPC inhibited peptidoglycan biosynthesis in a different way to currently available drugs, such as vancomycin and bacitracin, and showed no cross-resistance to these drugs.7 Recently, we discovered an unique effect of TPPC, which revitalizes and synergistically potentiates the activity of beta-lactams against MRSA strains.8,9 Based on its excellent biological properties, TPPC is considered to be a promising novel class of antibiotic against MRSA.
As shown in Figure 1, TPPC contains five hydroxyl groups, two carboxyl groups and a guanidine group. To investigate the detailed structure-activity relationships of TPPC, we are carrying out thorough investigations into these functional groups. In our previous report, we made a series of modifications to the carboxyl groups and synthesized the corresponding esters and amides.10 From these studies, it became clear that the two free carboxyl groups play an important role in the antibacterial activity of TPPC. In the current study, we focused on the role of the terminal guanidine group of the arginine residue in the antibacterial activity of TPPC. Herein, we describe modification of the guanidine group by the introduction of a pyrimidine ring and analysis of the antibacterial activities of the TPPC derivatives.
RESULTS AND DISCUSSION
As outlined in Scheme 1, TPPC was treated with a variety of 1, 3-diketones in the presence of potassium carbonate according to the Pinner method11,12 of pyrimidine synthesis. The reaction of TPPC with pentane-2, 4-dione produced 4, 6-dimethylpyrimidine derivative 1 with a 75% yield, along with a small amount of recovered TPPC (entry 1, Table 1), without causing cleavage of the lactone ring. The dehydration product or epimerized product was not observed. When the 3-position of pentane-2, 4-dione was substituted by a methyl group, the cyclization reaction also proceeded with similar efficiency to produce compound 2. However, the yield of cyclization product decreased when this position was substituted by a more bulky ethyl group (entry 3). Furthermore, substitution with an electron withdrawing group, such as chlorine, resulted in a reduction of reactivity and a significant decrease in the yield of cyclization product (entry 6). In the reaction with 1, 3-diketones possessing a trifluoromethyl group on the tip, a potent electron withdrawing group, pyrimidine derivatives 4 and 5 were obtained, although the yield fluctuated depending on the number of trifluoromethyl groups.
Table 2 indicates the 13C NMR data for the pyrimidine portion in compounds 1–6. It was observed that each signal of the pyrimidine ring of compounds 4 and 5 divided into two peaks. These signals were simplified by heating at 70 or 80 °C. Similarly, two singlet peaks for the trifluoromethyl group were observed in the 19F NMR spectra of compounds 4 and 5. Because the two singlet peaks converged to form
a single peak after heating, the existence of a rotamer caused by the trifluoromethylated pyrimidine ring was proposed.
Table 3 presents the antibacterial activities of the pyrimidine derivatives 1–6 against the clinical isolates of methicillin-sensitive S. aureus (MSSA, n=10) and MRSA (n=10). Compound 1 was less active than TPPC but showed good antibacterial activity against MSSA and MRSA strains. Antibacterial activity increased along with elongation of the alkyl chain at the 5′-position, and compound 3, possessing an ethyl substituent, displayed excellent antibacterial activity comparable with TPPC. It was interesting that the antibacterial activity was maintained even when the guanidine group was converted into a pyrimidine ring. By contrast, the antibacterial activities of compounds 4–6, possessing electron withdrawing groups such as trifluoromethyl or chlorine in the pyrimidine ring, were greatly decreased compared with TPPC. TPPC has been reported to inhibit peptidoglycan biosynthesis by binding to the important lipid carrier prenyl pyrophosphate.7 The bis-methyl ester of TPPC modifying the carboxyl groups does not bind to the target molecule, resulting in the loss of antibacterial activity.10 Analysis of complex formation exhibited that TPPC and compounds 1 and 4 can form a complex with prenyl pyrophosphate via calcium ions (refer to supporting information). However, obvious differences in binding affinities were observed between compounds (TPPC>1>4). Since a correlation has been found between anti-MRSA activity and binding affinity, further analysis of this phenomenon will help to elucidate the detailed mode of action of TPPC.
Although the antibacterial activity of compounds 1–6 varied greatly depending on the substituent at the pyrimidine ring, the possibility of chemical modification against the terminal guanidine group was demonstrated, unlike the case of the carboxyl groups. In addition, it became clear that the guanidine group of TPPC is not essential for the expression of its antibacterial activity. In summary, as a part of a structure-activity relationship study, we have synthesized pyrimidine derivatives of TPPC with a modified terminal guanidine group that retain antibacterial activity. These findings exemplify one potential direction for the derivatization of TPPC. The effects of different types of modifications of the guanidine group are currently being tested in follow-up studies in our laboratory.
EXPERIMENTAL
NMR spectra (1H at 500, 13C at 125.8, and 19F at 376.5 MHz) were recorded on a Bruker AVANCE 500 spectrometer (Bruker Biospin, Rheinstetten, Germany) at 300K, unless stated otherwise. The chemical shifts (δ) of 1H, 13C, and 19F spectra were measured downfield from internal Me4Si (for 1H and 13C) or internal Freon 11 (for 19F), and were confirmed by shift-correlated 2D spectra. The mass spectra were recorded using a Thermo Scientific LTQ Orbitrap mass spectrometer (Thermo Fisher Scientific, San Jose, CA, USA). Thin layer chromatography was performed on a Kieselgel 60 F254 (Merck, Darmstadt, Germany), and column chromatography was carried out on a Wakogel C-200 (Wako Pure Chemical Industries, Ltd., Osaka, Japan). Elemental analysis was performed using a Perkin Elmer PE 2400 II analyzer (PerkinElmer Inc., Waltham, MA, USA).
Starting materials. TPPC was prepared as reported previously, with slight modifications.4 All other chemicals used in this study were commercially available.
Evaluation of the minimum inhibitory concentrations (MICs). MICs were determined using a previously reported procedure.9
21-Deguanidino-21-(4, 6-dimethylpyrimidin-2-ylamino)tripropeptin C (1).
To a solution of TPPC (200 mg, 0.173 mmol) in pyridine (6 mL) were added pentane-2, 4-dione (86.7 mg, 0.866 mmol) and potassium carbonate (71.8 mg, 0.519 mmol) and the mixture was stirred for 60 h at 50 °C. Concentration gave a residue, which was dissolved in 50% aqueous THF (4 mL) and acidified with AcOH (0.4 mL). Concentration gave a residue, which was extracted with 1-BuOH, and the organic solution was successively washed with 1% aqueous AcOH and water, and concentrated. The resulting solid was purified by gradient column chromatography (CHCl3–MeOH–H2O = 60:10:1 to 60:30:6) to give 1 as a colorless solid (167.7 mg, 75%) together with TPPC recovered (15.9 mg, 8%). Compound 1 had 1H NMR (500 MHz, DMSO-d6) δ 0.84 [6H, d, J = 6.5 Hz, (CH3)2C-49], 1.50 (1H, m, H-49), 2.18 (6H, s, CH3-4′ and -6′), 2.28 (1H, slightly br d, J = 13 Hz, H-38a), 2.67 (1H, slightly br t, J = 12 Hz, H-38b), 3.75 (1H, br s, H-35), 5.04 (1H, s, HO), 5.09 (1H, br s, H-39), 5.46 (1H, br s, HO), 5.58 (1H, br s, HO), 6.32 (1H, s, H-5′), 6.83 (1H, slightly br t, J = 5.0 Hz, HN-21), 7.26 (1H, br s, HN), 8.03 (1H, d, J = 9.0 Hz, HN) and 8.50 (1H, br s, HN); HRMS (ESI): m/z calcd for C56H88N11O19 [M+H]+: 1218.6252, found: m/z=1218.6219. Anal. Calcd for C56H87N11O19・4H2O: C, 52.12; H, 7.42; N, 11.94. Found: C, 51.99; H, 7.27; N, 11.68.
21-Deguanidino-21-(4, 5, 6-trimethylpyrimidin-2-ylamino)tripropeptin C (2).
This compound was prepared from TPPC and 3-methylpentane-2, 4-dione in the similar manner described for 1 in 63% yield as a colorless solid; 1H NMR (500 MHz, DMSO-d6) δ 0.84 [6H, d, J = 6.5 Hz, (CH3)2C-49], 1.50 (1H, m, H-49), 2.00 (3H, s, CH3-5′), 2.22 (6H, s, CH3-4′ and -6′), 2.29 (1H, slightly br d, J = 13 Hz, H-38a), 2.67 (1H, slightly br t, J = 12 Hz, H-38b), 3.76 (1H, br s, H-35), 5.05 (1H, s, HO), 5.09 (1H, br s, H-39), 5.45 (1H, br s, HO), 5.58 (1H, br s, HO), 6.59 (1H, br s, HN-21), 7.27 (1H, br s, HN), 7.74 (1H, d, J = 9.0 Hz, HN), 8.02 (1H, d, J = 9.0 Hz, HN) and 8.48 (1H, br s, HN); HRMS (ESI): m/z calcd for C57H90N11O19 [M+H]+: 1232.6409, found: m/z=1232.6396. Anal. Calcd for C57H89N11O19・5H2O: C, 51.77; H, 7.55; N, 11.65. Found: C, 51.77; H, 7.79; N, 11.63.
21-Deguanidino-21-(5-ethyl-4, 6-dimethylpyrimidin-2-ylamino)tripropeptin C (3).
This compound was prepared from TPPC and 3-ethylpentane-2, 4-dione in the similar manner described for 1 in 34% yield as a colorless solid, along with the recovered TPPC (12%). Compound 3 had 1H NMR (500 MHz, DMSO-d6) δ 0.84 [6H, d, J = 6.5 Hz, (CH3)2C-49], 1.02 (3H, t, J = 7.5 Hz, CH3CH2-5′), 1.50 (1H, m, H-49), 2.25 (6H, s, CH3-4′ and -6′), 2.28 (1H, slightly br dd, J = 2 and 14 Hz, H-38a), 2.45 (2H, q, J = 7.5 Hz, CH3CH2-5′), 2.67 (1H, slightly br t, J = 13 Hz, H-38b), 3.75 (1H, br s, H-35), 5.05 (1H, s, HO), 5.09 (1H, br s, H-39), 5.45 (1H, br s, HO), 5.57 (1H, br s, HO), 6.62 (1H, slightly br s, HN-21), 7.27 (1H, br s, HN), 7.74 (1H, d, J = 9.0 Hz, HN), 7.79 (1H, br s, HN), 8.03 (1H, d, J = 9.0 Hz, HN) and 8.49 (1H, br s, HN); HRMS (ESI): m/z calcd for C58H92N11O19 [M+H]+: 1246.6565, found: m/z=1246.6531. Anal. Calcd for C58H91N11O19・4H2O: C, 52.84; H, 7.57; N, 11.69. Found: C, 52.86; H, 7.67; N, 11.84.
21-Deguanidino-21-[4-methy-6-(trifluoromethyl)pyrimidin-2-ylamino]tripropeptin C (4).
This compound was prepared from TPPC and 1, 1, 1-trifluoro-3-methylpentane-2, 4-dione in the similar manner described for 1 in 88% yield as a colorless solid; 1H NMR (500 MHz, DMSO-d6) δ 0.84 [6H, d, J = 6.5 Hz, (CH3)2C-49], 1.50 (1H, m, H-49), 2.28 (1H, slightly br d, J = 13 Hz, H-38a), 2.36 (3H, slightly br s, CH3-4′), 2.67 (1H, slightly br t, J = 13 Hz, H-38b), 3.75 (1H, br s, H-35), 5.04 (1H, s, HO), 5.10 (1H, br s, H-39), 5.48 (1H, br d, J = 6.0 Hz, HO), 5.60 (1H, br s, HO), 6.87 (1H, s, H-5′), 7.26 (1H, br s, HN), 7.65 (1H, br s, HN-21), 7.75 (1H, d, J = 9.0 Hz, HN), 8.03 (1H, d, J = 8.5 Hz, HN) and 8.51 (1H, br s, HN); 19F NMR (DMSO-d6)(at 300K): δ –69.34 (s) and –69.49 (s); (at 343K) : δ –69.44 (s). HRMS (ESI): m/z calcd for C56H85F3N11O19 [M+H]+: 1272.5970, found: m/z=1272.5957. Anal. Calcd for C56H84F3N11O19・4H2O: C, 50.03; H, 6.90; N, 11.46. Found: C, 50.02; H, 6.75; N, 11.22.
21-[4, 6-Bis(trifluoromethyl)pyrimidin-2-ylamino]-21-deguanidinotripropeptin C (5).
This compound was prepared from TPPC and 1, 1, 1, 5, 5, 5-hexafluoropentane-2, 4-dione in the similar manner described for 1 in 55% yield as a colorless solid, along with the recovered TPPC (45%). Compound 5 had 1H NMR (500 MHz, DMSO-d6) δ 0.84 [6H, d, J = 6.5 Hz, (CH3)2C-49], 1.49 (1H, m, H-49), 2.28 (1H, slightly br d, J = 13 Hz, H-38a), 2.67 (1H, slightly br t, J = 12 Hz, H-38b), 3.75 (1H, br s, H-35), 5.04 (1H, s, HO), 5.09 (1H, br s, H-39), 5.47 (1H, br s, HO), 5.60 (1H, br s, HO), 7.26 (1H, br s, HN), 7.36 (1H, s, H-5′), 7.76 (1H, d, J = 9.0 Hz, HN) and 8.03 (1H, d, J = 9.0 Hz, HN); 19F NMR (DMSO-d6) (at 300K): δ –69.35 (s) and –69.52 (s); (at 353K) : δ –69.53 (s). HRMS (ESI): m/z calcd for C56H81F6N11NaO19 [M+Na]+: 1348.5507, found: m/z=1348.5472. Anal. Calcd for C56H81F6N11O19・5H2O: C, 47.49; H, 6.48; N, 10.88. Found: C, 47.77; H, 6.39; N, 10.67.
21-(5-Chloro-4, 6-dimethylpyrimidin-2-ylamino)-21-deguanidinotripropeptin C (6).
This compound was prepared from TPPC and 3-chloropentane-2, 4-dione in the similar manner described for 1 in 10% yield as a colorless solid, along with the recovered TPPC (50%). Compound 6 had 1H NMR (500 MHz, DMSO-d6) δ 0.85 [6H, d, J = 6.5 Hz, (CH3)2C-49], 1.49 (1H, m, H-49), 2.31 (6H, s, CH3-4′ and -6′), 2.80 (1H, br s, H-38b), 3.83 (1H, br s, H-35), 5.03 (1H, s, HO), 7.09 (1H, slightly br t, J = 5.0 Hz, HN-21), 7.81 (1H, br s, HN) and 8.06 (1H, d, J = 7.5 Hz, HN); HRMS (ESI): m/z calcd for C56H87ClN11O19 [M+H]+: 1252.5863, found: m/z=1252.5853.
ACKNOWLEDGEMENTS
The authors are grateful to Kunio Inoue of the Institute of Microbial Chemistry (BIKAKEN), Tokyo, for determining the spectra of the antibacterial compounds. The authors would also like to thank Yoshiko Koyama and Dr Kiyoko Iijima of the Institute of Microbial Chemistry (BIKAKEN), Hiyoshi, for assistance with the NMR and mass spectrometry experiments.
References
1. L. B. Rice, Curr. Opin. Microbiol., 2009, 12, 476. CrossRef
2. S. Chang, D. M. Sievert, J. C. Hageman, M. L. Boulton, F. C. Tenover, F. P. Downes, S. Shah, J. T. Rudrik, G. R. Pupp, W. J. Brown, D. Cardo, and S. K. Fridkin, N. Engl. J. Med., 2003, 348, 1342. CrossRef
3. R. Sawa, Y. Takahashi, H. Hashizume, K. Sasaki, Y. Ishizaki, M. Umekita, M. Hatano, H. Abe, T. Watanabe, N. Kinoshita, Y. Homma, C. Hayashi, K. Inoue, S. Ohba, T. Masuda, M. Arakawa, Y. Kobayashi, M. Hamada, M. Igarashi, H. Adachi, Y. Nishimura, and Y. Akamatsu, Chem. Eur. J., 2012, 18, 15772. CrossRef
4. H. Hashizume, M. Igarashi, S. Hattori, M. Hori, M. Hamada, and T. Takeuchi, J. Antibiot., 2001, 54, 1054. CrossRef
5. H. Hashizume, S. Hirosawa, R. Sawa, Y. Muraoka, D. Ikeda, H. Naganawa, and M. Igarashi, J. Antibiot., 2004, 57, 52. CrossRef
6. H. Hashizume, S. Hattori, M. Igarashi, and Y. Atamatsu, J. Antibiot., 2004, 57, 394. CrossRef
7. H. Hashizume, R. Sawa, S. Harada, M. Igarashi, H. Adachi, Y. Nishimura, and A. Nomoto, Antimicrob. Agents Chemother., 2011, 55, 3821. CrossRef
8. H. Hashizume, Y. Takahashi, S. Harada, and A. Nomoto, 54th Interscience Conference on Antimicrobial Agents Chemotherapy, Washington D. C., Abstr. F1-2078, 2014.
9. H. Hashizume, Y. Takahashi, S. Harada, and A. Nomoto, J. Antibiot., 2015, 68, 373. CrossRef
10. S. Hirosawa, Y. Takahashi, H. Hashizume, T. Miyake, and Y. Akamatsu, J. Antibiot., 2014, 67, 265. CrossRef
11. A. Pinner, Ber., 1893, 26, 2122. CrossRef
12. M. V. R. Reddy, M. K. Harper, and D. J. Faulkner, Tetrahedron, 1998, 54, 10649. CrossRef