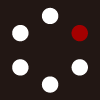
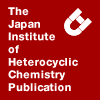
HETEROCYCLES
An International Journal for Reviews and Communications in Heterocyclic ChemistryWeb Edition ISSN: 1881-0942
Published online by The Japan Institute of Heterocyclic Chemistry
e-Journal
Full Text HTML
Received, 16th March, 2016, Accepted, 11th April, 2016, Published online, 28th April, 2016.
■ Synthesis and Antileukemic Activity of New Fluorinated 5-Aza-9-deazapurines
Felicia Phei Lin Lim, Koon Kee Kow, Eng Hwa Yeo, Sek Chuen Chow, and Anton V. Dolzhenko*
School of Pharmacy, Monash University Malaysia, Jalan Lagoon Selatan, Subang Jaya, Selangor 47500, Malaysia
Abstract
Novel fluorinated 4-benzylamino-7-phenylpyrazolo[1,5-a]- [1,3,5]triazin-2-amines were prepared using an efficient and practical approach. The chemoselectivity of condensation of pyrazolylguanidine and trichloroacetonitrile was found to be solvent-dependent and, when conducted in toluene, this reaction afforded 7-phenyl-4-trichloromethylpyrazolo[1,5-a]- [1,3,5]triazin-2-amine as the main product. This key intermediate underwent nucleophilic replacement of the trichloromethyl group with fluorinated benzylamines providing a series of the target compounds. Antiproliferative activity of the prepared compounds against Jurkat T cells was explored using MTS assay. Morphological changes observed in cells treated by the most potent compounds of this series, suggested that these compounds induced apoptosis in cells.The unique character of fluorinated molecules have been well exploited in drug discovery programs.2-4 Utilizing fluorine substitution, new biologically active compounds have been discovered including those with anticancer properties.5 Notable examples include fluorinated purines, which displayed significant antiproliferative activity against several cancer cell lines. Particularly, all three anticancer lead compounds, recently identified in the biological evaluation of an extensive library of purine derivatives, possessed a trifluoromethyl group on the aminosubstituent in position 6 of the heterocyclic system.6 In another focused library of purines with the substituted 6-benzylamino fragment, compounds with fluorinated benzene ring of this moiety were found to be active in cytokinin, antitumor and kinase bioassays.7 On the basis of the purine motif, advancements in drug design and development have inspired extensive research into isosteric heterocyclic systems. Purine isosteres with a 1,3,5-triazine ring have been demonstrated to be versatile scaffolds for the discovery and development of new bioactive compounds.8 Among this group of heterocyclic systems, 1,2,4-triazolo[1,5-a][1,3,5]triazines (5-azapurines) and pyrazolo[1,5-a]- [1,3,5]triazines (5-aza-9-deazapurines) were identified as particularly promising scaffolds for the development of new bioactive compounds.8 Previously, our studies on fluorinated 7-benzylamino-2-phenyl-1,2,4-triazolo[1,5-a][1,3,5]triazin-5-amines (1) lead to the development of practical synthesis and identification of compounds with anticancer properties (Figure 1).9 In our continuous search for new antiproliferative agents utilizing purine analogues, we attempted to alter scaffold 1 by removing a nitrogen atom to obtain 5-aza-9-deazapurine isostere 2 with identical substitution pattern. The choice of this scaffold was also based on our previous promising results on 5-aza-9-deazapurines demonstrating anticancer properties.10,11 Herein, we report synthesis of a small library of new fluorinated 4-benzylamino-7-phenylpyrazolo[1,5-a][1,3,5]triazin-2-amines and antiproliferative activity of these compounds against leukemic Jurkat T cells.
Previously, we synthesized bioactive 1,2,4-triazolo[1,5-a][1,3,5]triazines 19 using a practical method12,13 based on the construction of a trichloromethyl-substituted 1,3,5-triazine ring onto N-(3-phenyl-1,2,4-triazol-5-yl)guanidine followed by successful nucleophilic replacement of the trichloromethyl group by amines. Following the previous general approach for the preparation of 1, we now designed a synthesis of structurally related pyrazolo[1,5-a][1,3,5]triazines 2, albeit utilizing pyrazole-based intermediates to obtained the desired products.
The conversion of 5(3)-amino-3(5)-phenylpyrazole (3), obtained from the reaction of benzoylacetonitrile and hydrazine14 to N-(3-phenylpyrazol-5-yl)guanidine (4) was successfully achieved by the treatment of 3 with cyanamide in the presence of acid (Scheme 1). In the next step, chemoselectivity of the ring closure reaction of guanidine 4 with trichloroacetonitrile was found to be solvent-dependent. This reaction proceeded in ethanol media with elimination of chloroform, but in toluene, a mixture of two products 5 (major 77%) and 6 (minor 8%) was obtained. The formation of compound 5 involved elimination of ammonia, which was probably present in the reaction mixture for sufficient time to displace the trichloromethyl group of 5, therefore leading to the formation of side product 6. We manage to isolate the desired product 5 in pure form via fractional recrystallization exploiting its significantly higher solubility in hot toluene compared to 6. The structure of 5 was supported by NMR spectra, particularly by the characteristic singlet at 7.66 ppm in the 1H NMR spectra attributed to the amino group on C-2 of the heterocyclic system and the signal at 90 ppm in the 13C NMR spectra indicative of the trichloromethyl group.
A small library of fluorinated 4-benzylamino-7-phenylpyrazolo[1,5-a][1,3,5]triazin-2-amines 2 was generated by nucleophilic substitution on C-4 of the heterocyclic system using fluorine / trifluoromethyl substituted benzylamines, which replaced the trichloromethyl group. One of the obvious advantages of employing trichloromethyl group as a leaving group in this reaction was volatility and relatively inert characteristics of the byproduct chloroform.
The structure of the synthesized compounds 2 was confirmed by NMR spectral data, which were similar to their structural analogues 1 with 1,2,4-triazolo[1,5-a][1,3,5]triazine skeleton. However, in the 1H NMR spectra of compounds 2, the NH proton signal of the benzylamino moiety appeared at 8.66-8.81 ppm, while the signal of the same NH proton of analogues 1 was downfield shifted to 9.01-9.12 ppm due to the higher deshielding effect of the more electron-deficient 1,2,4-triazolo[1,5-a][1,3,5]triazine ring system.9
The prepared compounds 2 were subjected to biological screening for antiproliferative activity against Jurkat T cells. The antiproliferative activity of the prepared compounds 2 against Jurkat T cell lines was evaluated in vitro using MTS assay and activity was expressed as IC50 values measured at two time intervals: 24 h and 48 h (Table 1). In general, the trifluoromethyl-substituted 4-benzylamino-7-phenyl- pyrazolo[1,5-a][1,3,5]triazin-2-amines (2d, 2e, and 2f) were found to be almost twice more potent in inhibiting growth of Jurkat T cells as compared to fluoro-substituted compounds 2a, 2b, and 2c. However, adding another trifluoromethyl group to the benzyl moiety (compound 2g) dramatically decreased antiproliferative activity with no observable effect on viability of Jurkat T cells at the highest tested concentration 100 μM. The most active compounds were the 2-trifluoromethyl- and 4-trifluoromethyl- substituted derivatives 2d and 2f, which demonstrated similar IC50 values of 38 μM and 41 μM against Jurkat T cells 48 hours after the treatment. Both 2d and 2f affected the viability of Jurkat T cells in a concentration- and time-dependent manner reducing cell numbers at concentration 100 μM to less than 5% of the control values.
The morphological changes of Jurkat T cells induced by compounds 2d and 2f were further examined using fluorescence microscopy after fixing the cells with 4% paraformaldehyde and staining with Hoechst 33358 dye. Normal nuclear morphology, represented by a dark blue color, with no prominent apoptotic and necrotic cells was observed in the untreated control Jurkat T cells (Figure 2a). Jurkat T cells, 24 hours after treatment with 50 μM of compound 2d and 2f, showed typical hallmarks of apoptosis, such as fragmentation of the nuclei into apoptotic bodies (Figure 2b,c).15 Another observed characteristic of apoptosis was chromatin condensation as represented by the bright fluorescent blue colour owing to the condensed nuclear material. The Jurkat T cells also appeared as multinucleated cell and displayed nuclear margination. Hence, we proposed that compounds 2d and 2f manifest their antiproliferative effect by induction of apoptosis in Jurkat T cells.
We successfully designed and prepared new fluorinated 4-benzylamino-7-phenyl- pyrazolo[1,5-a][1,3,5]triazin-2-amines possessing antileukemic properties. It was found that the most active compounds 2d and 2f induced apoptosis in Jurkat T cells resulting in their significant antiproliferative effect, which make them promising leads for further development of new therapeutic agents targeting T-cell leukemia.
EXPERIMENTAL
General Methods. Melting points were determined on a Gallenkamp melting point apparatus. 1H and 13C NMR spectra were recorded on a Bruker Avance III spectrometer (400 MHz), using DMSO-d6 as a solvent and TMS as an internal reference. TLC analysis was performed on aluminum plates coated with Silica gel F (Analtech) with detection by UV light.
Synthesis of N-(3-phenylpyrazol-5-yl)guanidine (4).
5(3)-Amino-3(5)-phenylpyrazole (3, 3.4 g, 21.4 mmol), cyanamide (2.6 g, 60 mmol) and conc. HCl (3.75 mL, 38.5 mmol) were heated in EtOH (17 mL) under reflux for 7 h. After cooling, aqueous solution of K2CO3 (5%, 250 mL) was added and the product formed was filtered and dried under vacuum. An analytical sample was recrystallized from MeOH.
Yield 56%; mp 137-138 °C.
1H NMR (400 MHz, Me2SO-d6): δ 6.18 (1H, br s, H-8), 6.59 (4H, br s, NH(=NH)NH2), 7.29 (1H, t, 3J = 7.3 Hz, H-4′), 7.39 (2H, t, 3J = 7.6 Hz, H-3′ and H-5′), 8.03 (2H, d, 3J = 8.0 Hz, H-2′ and H-6′).
13C NMR (100 MHz, Me2SO-d6): δ 92.1 (br s, C-4), 125.0 (C-3′ and C-5′), 127.5 (C-4′), 128.7 (C-2′ and C-6′), 131.6 (br s, C-1′), 145.3 (br s, C-3), 152.4 (br s, C-5), 154.8 (br s, NHC(=NH)NH2).
Anal. Calcd for C10H11N5: C, 59.69; H, 5.51; N, 34.80%. Found: C, 59.55; H, 5.64; N, 34.68%.
Reaction of N-(3-phenylpyrazol-5-yl)guanidine (4) with trichloroacetonitrile.
N-(3-Phenylpyrazol-5-yl)guanidine (4, 1.6 g, 7.85 mmol) and trichloroacetonitrile (1.6 mL, 16 mmol) were heated in toluene (15 mL) under reflux for 30 min. After cooling, the precipitate was filtered and underwent fractional recrystallization from toluene to furnish pure 2-amino-7-phenyl-4-trichloromethyl- pyrazolo[1,5-a][1,3,5]triazine (5)
Yield 77%; mp 240-242 °C; Rf 0.74 (AcOEt : hexane – 1:1).
1H NMR (400 MHz, Me2SO-d6): δ 6.68 (1H, s, H-8), 7.45-7.55 (3H, m, H-3′, H-4′ and H-5′), 7.66 (2H, s, NH2), 8.03 (2H, dd, 3J = 8.2 Hz, 4J = 1.5 Hz, H-2′ and H-6′).
13C NMR (100 MHz, Me2SO-d6): δ 87.9 (C-8), 90.0 (CCl3), 126.5 (C-3′ and C-5′), 128.8 (C-2′ and C-6′), 129.7 (C-4′), 131.8 (C-1′), 149.6 (C-4), 153.9 (C-8a), 156.3 (C-7), 157.3 (C-2).
Anal. Calcd for C12H8Cl3N5: C, 43.86; H, 2.45; N, 21.31. Found: C, 43.75; H, 2.52; N, 21.22.
An insoluble in toluene fraction was recrystallized from EtOH to give 2,4-diamino-7-phenyl- pyrazolo[1,5-a][1,3,5]triazine (6)
Yield 8%; mp 338-340 °C; Rf 0.40 (AcOEt).
1H NMR (400 MHz, Me2SO-d6): δ 6.21 (1H, s, H-8), 6.40 (2H, s, NH2), 7.39 (1H, t, 3J = 7.3 Hz, H-4′), 7.46 (2H, t, 3J = 7.3 Hz, H-3′ and H-5′), 7.82 (2H, brs, NH2), 7.96 (2H, d, 3J = 7.3 Hz, H-2′ and H-6′).
13C NMR (100 MHz, Me2SO-d6): δ 86.9 (C-8), 126.1 (C-3′ and C-5′), 128.6 (C-2′ and C-6′), 128.7 (C-4′), 132.9 (C-1′), 150.3 (C-4), 152.3 (C-8a), 154.9 (C-7), 159.2 (C-2).
Anal. Calcd for C11H10N6: C, 58.40; H, 4.46; N, 37.15. Found: C, 58.28; H, 4.50; N, 36.97.
General method for the synthesis of fluorinated 4-benzylamino-7-phenylpyrazolo[1,5-a][1,3,5]triazin- 2-amines (2a-g). 2-Amino-7-phenyl-4-trichloromethylpyrazolo[1,5-a][1,3,5]triazine (6, 200 mg, 0.61 mmol) was added to the solution of the appropriate fluorinated benzylamine (0.78 mmol) in MeOH (7 mL), the mixture was stirred at ambient temperature for 24 h and the reaction progress was monitored by TLC. After evaporation of the solvent, the residue was recrystallized from MeOH (2a-2e, 2g) or toluene (2f) to give pure compounds 2a-g.
4-(2-Fluorobenzylamino)-7-phenylpyrazolo[1,5-a][1,3,5]triazin-2-amine (2a).
Yield 90%; mp 177-179 °C; Rf 0.47 (AcOEt : hexane – 1:1).
1H NMR (400 MHz, Me2SO-d6): δ 4.75 (2H, d, 3J = 6.2 Hz, CH2), 6.26 (1H, s, H-8), 6.53 (2H, s, NH2), 7.15-7.24 (2H, m, H-3′′ and H-5′′), 7.29-7.49 (5H, m, H-3′, H-4′, H-5′, H-4′′ and H-6′′), 7.98 (2H, d, 3J = 7.3 Hz, H-2′ and H-6′), 8.66 (1H, t, 3J = 6.2 Hz, NH).
13C NMR (100 MHz, Me2SO-d6): δ 36.9 (d,3JCF = 4.9 Hz, CH2), 87.1 (C-8), 115.0 (d, 2JCF = 20.9 Hz, C-3′′), 124.9 (d, 4JCF = 3.5 Hz, C-5′′), 125.5 (d, 2JCF = 14.5 Hz, C-1′′), 126.0 (C-3′ and C-5′), 128.5 (C-2′ and C-6′), 128.7 (C-4′), 128.9 (d, 3JCF = 8.1 Hz, C-4′′), 129.0 (d, 3JCF = 4.3 Hz, C-6′′), 132.8 (C-1′), 148.9 (C-4), 152.1 (C-8a), 155.0 (C-7), 159.8 (d, 1JCF = 244.3 Hz, C-2′′),158.8 (C-2).
Anal. Calcd for C18H15FN6: C, 64.66; H, 4.52; N, 25.14. Found: C, 64.60; H, 4.70; N, 24.95.
4-(3-Fluorobenzylamino)-7-phenylpyrazolo[1,5-a][1,3,5]triazin-2-amine (2b).
Yield 75%; mp 176-177 °C; Rf 0.38 (AcOEt : hexane – 1:1).
1H NMR (400 MHz, Me2SO-d6): δ 4.69 (2H, d, 3J = 6.4 Hz, CH2), 6.27 (1H, s, H-8), 6.57 (2H, s, NH2), 7.09 (1H, ddd, 3J = 8.6 Hz, 3JHF = 8.6 Hz, 4J = 2.6 Hz, H-4′′), 7.23-7.28 (2H, m, H-2′′ and H-6′′), 7.36-7.43 (2H, m, H-4′ and H-5′′), 7.47 (2H, t, 3J = 7.3 Hz, H-3′ and H-5′), 7.98 (2H, d, 3J = 7.3 Hz, H-2′ and H-6′), 8.75 (1H, t, 3J = 6.4 Hz, NH).
13C NMR (100 MHz, Me2SO-d6): δ 42.5 (d, 4JCF = 1.7 Hz, CH2), 113.7 (d, 2JCF = 20.9 Hz, C-4′′), 114.2 (d, 2JCF = 21.6 Hz, C-2′′), 123.4 (d, 4JCF = 2.8 Hz, C-6′′), 126.1 (C-3 and C-5), 128.5 (C-2 and C-6), 128.7 (C-4′), 130.2 (d, 3JCF = 8.3 Hz, C-5′′), 132.8 (C-1′), 141.8 (d, 3JCF = 7.1 Hz, C-1′′), 148.8 (C-4), 152.1 (C-8a), 155.0 (C-7), 158.8 (C-2), 162.1 (d, 1JCF = 243.4 Hz, C-3′′).
Anal. Calcd for C18H15FN6: C, 64.66; H, 4.52; N, 25.14. Found: C, 64.56; H, 4.68; N, 25.02.
4-(4-Fluorobenzylamino)-7-phenylpyrazolo[1,5-a][1,3,5]triazin-2-amine (2c).
Yield 48%; mp 178-179 °C; Rf 0.44 (AcOEt : hexane – 1:1).
1H NMR (400 MHz, Me2SO-d6): δ 4.65 (2H, d, 3J = 6.2 Hz, CH2), 6.25 (1H, s, H-8), 6.55 (2H, s, NH2), 7.16 (2H, dd, 3J = 8.7 Hz, 3JHF = 9.1 Hz, H-3′′ and H-5′′), 7.40 (1H, t, 3J = 7.4 Hz, H-4′), 7.44-7.49 (4H, m, H-3′, H-5′, H-2′′ and H-6′′), 7.97 (2H, d, 3J = 7.9 Hz, H-2′ and H-6′), 8.71 (1H, t, 3J = 6.3 Hz, NH).
13C NMR (100 MHz, Me2SO-d6): δ 42.3 (CH2), 87.2 (C-8), 115.0 (d, 2JCF = 21.3 Hz, C-3′′ and C-5′′) 126.1 (C-3′ and C-5′),128.6 (C-2′ and C-6′), 128.8 (C-4′), 129.6 (d, 3JCF = 8.1 Hz, C-2′′ and C-6′′), 132.9 (C-1′), 135.1 (d, 4JCF = 3.0 Hz, C-1′′), 148.8 (C-4), 152.2 (C-8a), 155.0 (C-7), 158.9 (C-2), 161.3 (d, 1JCF = 242.5 Hz, C-4′′).
Anal. Calcd for C18H15FN6: C, 64.66; H, 4.52; N, 25.14. Found: C, 64.60; H, 4.58; N, 25.09.
7-Phenyl-4-(2-trifluoromethylbenzylamino)pyrazolo[1,5-a][1,3,5]triazin-2-amine (2d).
Yield 56%; mp 138-140 °C; Rf 0.44 (AcOEt : hexane – 1:1).
1H NMR (400 MHz, Me2SO-d6): δ 4.92 (2H, d, 3J = 6.3 Hz, CH2), 6.30 (1H, s, H-8), 6.54 (2H, s, NH2), 7.41 (1H, t, 3J = 7.3 Hz, H-4′), 7.48 (2H, t, 3J = 7.3 Hz, H-3′ and H-5′), 7.49 (1H, t, 3J = 7.7 Hz, H-5′′), 7.56 (1H, d, 3J = 7.8 Hz, H-6′′), 7.65 (1H, t, 3J = 7.6 Hz, H-4′′), 7.76 (1H, d, 3J = 7.7 Hz, H-3′′), 8.00 (2H, d, 3J = 7.4 Hz, H-2′ and H-6′), 8.76 (1H, t, 3J = 6.4 Hz, NH).
13C NMR (100 MHz, Me2SO-d6): δ 39.7 (CH2), 87.3 (C-8), 124.5 (q, 1JCF = 274.3 Hz, CF3), 125.8 (q, 3JCF = 5.6 Hz, C-3′′), 125.9 (q, 2JCF = 30.2 Hz, C-2′′), 126.1 (C-3′ and C-5′), 127.4 (C-4′′), 131.7 (C-6′′), 128.6 (C-2′ and C-6′), 128.8 (C-4′), 132.78 (C-5′′), 132.82 (C-1′), 137.0 (q, 3JCF = 1.5 Hz, C-1′′), 149.1 (C-4), 152.1 (C-8a), 155.2 (C-7), 158.8 (C-2).
Anal. Calcd for C19H15F3N6: C, 59.37; H, 3.93; N, 21.87. Found: C, 59.28; H, 4.12; N, 21.63.
7-Phenyl-4-(3-trifluoromethylbenzylamino)pyrazolo[1,5-a][1,3,5]triazin-2-amine (2e).
Yield 76%; mp 162-164 °C; Rf 0.44 (AcOEt : hexane – 1:1).
1H NMR (400 MHz, Me2SO-d6): δ 4.76 (2H, d, 3J = 6.3 Hz, CH2), 6.26 (1H, s, H-8), 6.56 (2H, s, NH2), 7.40 (1H, t, 3J = 7.3 Hz, H-4′), 7.47 (2H, t, 3J = 7.3 Hz, H-3′ and H-5′), 7.59 (1H, t, 3J = 7.6 Hz, H-5′′), 7.64 (1H, d, 3J = 7.8 Hz, H-6′′), 7.75 (1H, d, 3J = 7.6 Hz, H-4′′), 7.78 (1H, s, H-2′′), 7.98 (2H, d, 3J = 7.4 Hz, H-2′ and H-6′), 8.81 (1H, t, 3J = 6.4 Hz, NH).
13C NMR (100 MHz, Me2SO-d6): δ 42.6 (CH2), 87.2 (C-8), 123.8 (q, 3JCF = 3.7 Hz, C-4′′), 124.1 (q, 3JCF = 3.8 Hz, C-2′′), 124.2 (q, 1JCF = 272.2 Hz, CF3), 126.1 (C-3′ and C-5′), 128.6 (C-2′ and C-6′), 128.8 (C-4′), 129.0 (q, 2JCF = 31.5 Hz, C-3′′), 129.4 (C-5′′), 131.7 (C-6′′), 132.8 (C-1′), 140.4 (C-1′′), 148.8 (C-4), 152.2 (C-8a), 155.1 (C-7), 158.9 (C-2).
Anal. Calcd for C19H15F3N6: C, 59.37; H, 3.93; N, 21.87. Found: C, 59.30; H, 4.08; N, 21.73.
7-Phenyl-4-(4-trifluoromethylbenzylamino)pyrazolo[1,5-a][1,3,5]triazin-2-amine (2f).
Yield 72%; mp 188-189 °C; Rf 0.34 (AcOEt : hexane – 1:1).
1H NMR (400 MHz, Me2SO-d6): δ 4.76 (2H, d, 3J = 6.4 Hz, CH2), 6.26 (1H, s, H-8), 6.54 (2H, s, NH2), 7.40 (1H, t, 3J = 7.3 Hz, H-4′), 7.47 (2H, t, 3J = 7.3 Hz, H-3′ and H-5′), 7.62 (2H, d, 3J = 8.2 Hz, H-2′′ and H-6′′), 7.71 (2H, d, 3J = 8.2 Hz, H-3′′ and H-5′′), 7.98 (2H, d, 3J = 7.4 Hz, H-2′ and H-6′), 8.81 (1H, t, 3J = 6.4 Hz, NH).
13C NMR (100 MHz, Me2SO-d6): δ 42.6 (CH2), 87.2 (C-8), 124.3 (q, 1JCF = 271.8 Hz, CF3), 125.1 (q, 3JCF = 3.8 Hz, C-3′′ and C-5′′), 126.0 (C-3′ and C-5′), 127.6 .3 (q, 2JCF = 31.7 Hz, C-4′′), 128.0 (C-2′′ and C-6′′), 128.5 (C-2′ and C-6′), 128.7 (C-4′), 132.8 (C-1′), 143.7 (C-1′′), 148.8 (C-4), 152.1 (C-8a), 155.0 (C-7), 158.8 (C-2).
Anal. Calcd for C19H15F3N6: C, 59.37; H, 3.93; N, 21.87. Found: C, 59.18; H, 4.15; N, 21.67.
4-[3,5-bis-(Trifluoromethyl)benzylamino]-7-phenylpyrazolo[1,5-a][1,3,5]triazin-2-amine (2g).
Yield 68%; mp 238-240 °C; Rf 0.56 (AcOEt : hexane – 1:1).
1H NMR (400 MHz, Me2SO-d6): δ 4.85 (2H, d, 3J = 6.5 Hz, CH2), 6.27 (1H, s, H-8), 6.59 (2H, s, NH2), 7.41 (1H, t, 3J = 7.2 Hz, H-4′), 7.47 (2H, t, 3J = 7.3 Hz, H-3′ and H-5′), 7.98 (2H, d, 3J = 7.3 Hz, H-2′ and H-6′), 8.03 (1H, s, H-4′′), 8.15 (2H, s, H-2′′ and H-6′′), 8.86 (1H, t, 3J = 6.5 Hz, NH).
13C NMR (100 MHz, Me2SO-d6): δ 42.4 (CH2), 87.3 (C-8), 120.9 (sept, 3JCF = 3.8 Hz, C-4′′), 123.3 (q, 1JCF = 272.8 Hz, CF3 2), 126.1 (C-3′ and C-5′), 128.5 (q, 3JCF = 3.7 Hz, C-2′′ and C-6′′), 128.6 (C-2′ and C-6′), 128.8 (C-4′), 130.1 (q, 2JCF = 32.8 Hz, C-3′′ and C-5′′), 132.8 (C-1′), 142.4 (C-1′′), 148.8 (C-4), 152.2 (C-8a), 155.2 (C-7), 158.8 (C-2).
Anal. Calcd for C20H14F6N6: C, 53.10; H, 3.12; N, 18.58. Found: C, 52.92; H, 3.22; N, 18.44.
Cell viability assay
Cell viability was assessed colourimetrically using the Cell Titer 96TM Aqueous cell proliferation assay16 based on the reduction of 5-(3-carboxymethoxyphenyl)2-(4,5-dimethylthiazolyl)-3-(4-sulfophenyl)- tetrazolium, inner salt (MTS) by dehydrogenase enzymes in living cells to coloured water-soluble formazan, concentration of which is directly proportional to the number of living cells in the culture.17 With the appropriate blank (medium alone), aliquots of 100 μL Jurkat T cell suspension (~25,000 cells) were dispensed into the wells of a 96-well flat-bottomed plate followed by treatment with compounds 2a-2g at final concentrations 12.5, 25, 50, and 100 μM. In corresponding control wells of the same plates, Jurkat T cells were grown without treatment. Two 96-well flat-bottomed plates were incubated at 37 °C in an incubator for 24 and 48 h respectively. After incubations, 20 μL of freshly prepared MTS solution (333 μg/mL) in phosphate-buffered saline (PBS) was added to each well and the plate was incubated at 37 °C for 2 h. Absorbance was measured at 490 nm using a Tecan i-control plate reader. Assays were conducted in duplicates in 3 independent experiments for each of 24 and 48 h time intervals. The IC50 values were calculated using Graphpad Prism (version 6) biostatistical software. Quantitative experimental data were presented as mean ± standard deviation.
Fluoroscence microscopy
Aliquots of 1000 μL Jurkat T cell suspension (~250,000 cells) were dispensed into wells of a 24-well flat bottomed plate, followed by treatment with compounds 2d and 2f (final concentration 50 μM). A series of wells with Jurkat T cell on the same plate was remained untreated and was used as a control. The plate was incubated at 37 °C in an incubator for 24 h; cells were harvested and centrifuged down. The supernatant was removed and cell pellet was suspended in 50 μL of paraformaldehyde solution (4% in PBS) at room temperature for 30 min. After removal of supernatant, the cells were re-suspended in 50 μL of PBS containing 50 μg/mL Hoechst 33342 DNA dye and incubated for 30 min at room temperature. After removal of supernatant, the stained cells were re-suspended in 20 μL glycerol / PBS (50:50, v/v) and 2-5 μL of the mixture with stained cells was placed on slides and viewed using an Olympus BX51 fluorescence microscope. Cells were judged to be apoptotic if they displayed the characteristic hallmarks of apoptosis.18
ACKNOWLEDGEMENTS
This work is supported by the Ministry of Higher Education, Malaysia under Fundamental Research Grant Scheme (FRGS) and by a seed grant from the Infection and Immunity Cluster of the Tropical Medicine, Biology and Genomics Platform (Monash University Malaysia).
References
1. Part 30 in the series “Fused heterocyclic systems with an s-triazine ring”, for part 29, see F. P. L. Lim, G. Luna, and A. V. Dolzhenko, Tetrahedron Lett., 2015, 56, 7016. CrossRef
2. K. Kaur, V. Kumar, and G. K. Gupta, J. Fluorine Chem., 2015, 178, 306. CrossRef
3. G. N. Lipunova, E. V. Nosova, V. N. Charushin, and O. N. Chupakhin, J. Fluorine Chem., 2015, 175, 84. CrossRef
4. W. Zhu, J. Wang, S. Wang, Z. Gu, J. L. Acena, K. Izawa, H. Liu, and V. A. Soloshonok, J. Fluorine Chem., 2014, 167, 37. CrossRef
5. O.-u.-R. Abid, M. Khalid, M. T. Hussain, M. Hanif, G. Qadeer, N. H. Rama, A. Kornienko, and K. M. Khan, J. Fluorine Chem., 2012, 135, 240.
6. Z. Demir, E. B. Guven, S. Ozbey, C. Kazak, R. C. Atalay, and M. Tuncbilek, Eur. J. Med. Chem., 2015, 89, 701. CrossRef
7. K. Dolezal, I. Popa, V. Krystof, L. Spichal, M. Fojtikova, J. Holub, R. Lenobel, T. Schmuelling, and M. Strnad, Bioorg. Med. Chem., 2006, 14, 875. CrossRef
8. F. P. L. Lim and A. V. Dolzhenko, Eur. J. Med. Chem., 2014, 85, 371. CrossRef
9. A. V. Dolzhenko, B. J. Tan, G. N. C. Chiu, W. K. Chui, and A. V. Dolzhenko, J. Fluorine Chem., 2015, 175, 68. CrossRef
10. H. Bera, P. K. Ojha, B. J. Tan, L. Sun, A. V. Dolzhenko, W. K. Chui, and G. N. C. Chiu, Eur. J. Med. Chem., 2014, 78, 294. CrossRef
11. L. Sun, J. Li, H. Bera, A.V. Dolzhenko, G. N. C. Chiu, and W. K. Chui, Eur. J. Med. Chem., 2013, 70, 400. CrossRef
12. A. V. Dolzhenko, G. Pastorin, A. V. Dolzhenko, and W. K. Chui, Tetrahedron Lett., 2008, 49, 7180. CrossRef
13. F. P. L. Lim and A. V. Dolzhenko, Tetrahedron Lett., 2014, 55, 6684. CrossRef
14. I. Kim, J. H. Song, C. M. Park, J. W. Jeong, H. R. Kim, J. R. Ha, Z. No, Y.-L. Hyun, Y. S. Cho, N. S. Kang, and D. J. Jeon, Bioorg. Med. Chem. Lett., 2010, 20, 922. CrossRef
15. A. Saraste and K. Pulkki, Cardiovasc. Res., 2000, 45, 528. CrossRef
16. A. H. Cory, T. C. Owen, J. A. Barltrop, and. J. G. Cory, Cancer Commun., 1991, 3, 207.
17. J. A. Barltrop, T. C. Owen, A. H. Cory, and J. G. Cory, Bioorg. Med. Chem. Lett., 1991, 1, 611. CrossRef
18. B. Chazotte, Cold Spring Harb. Protoc., 2011, 83, doi:10.1101/pdb.prot5557 CrossRef