Unraveling the Structure of the Variola Topoisomerase IB-DNA Complex: A Possible New Twist on Smallpox Therapy
Smallpox, a serious and highly contagious disease caused by the variola virus (a member of the Poxviridae virus family) (1, 2), is one of the most severe infectious human diseases, with a mortality rate as high as 30%. At the present time, the only prevention against smallpox is vaccination. Owing to the efforts of a successful worldwide vaccination program, the disease was declared eradicated by the World Health Organization in 1980. Consequently, vaccination of the general population in the United States and the rest of the world ceased after this date. Because it is estimated that vaccinated individuals retain immunity against smallpox for approximately ten years, the current populace of the world is considered to be immunonaïve to the variola virus (3).
Despite the fact that the last naturally occurring case of smallpox was in 1997, the high transmission rate of variola virus, coupled with the deadly nature of smallpox, makes this virus a potentially devastating weapon for bioterrorism (1, 2). Currently, there is no specific treatment for smallpox; however, a recent study by Perry et al. on the structure of the variola topoisomerase IB–DNA complex provides an intriguing starting point for the rational design of drugs with potential activity against smallpox (4).
Topoisomerases are ubiquitous enzymes that participate in virtually every DNA process (5–9). They regulate torsional stress in the double helix, alleviate DNA overwinding that is induced by the movement of tracking systems such as replication forks and transcription complexes, and remove knots and tangles from the genetic material (Box 1).
DNA Topology
Topology is the branch of mathematics that is concerned with relationships that are not altered by elastic deformation, twisting, or stretching. How does this apply to DNA? DNA is essentially an extremely long double-stranded rope in which the two strands are coiled about each other. As long as the ends of DNA are fixed in space, topological properties are not altered unless one or both chains of the double helix are broken (9–11). Topological properties of DNA include relationships between the two strands of the double helix (DNA under- and overwinding) and relationships between helices (DNA knotting and tangling) (9–11). DNA is globally underwound (i.e., negatively supercoiled) in living systems from bacteria to humans. Underwinding makes it easier to separate the two strands of the double helix and accelerates rates of replication and transcription. Conversely, overwinding (i.e., positive supercoiling) occurs ahead of DNA tracking systems. It makes it more difficult to open the double helix and therefore blocks essential processes. Knots (intramolecular) and tangles (intermolecular) are formed in DNA during recombination and replication. Knots must be resolved in order for replication and transcription to proceed. Tangles must be resolved in order for daughter chromosomes to segregate properly during meiosis and mitosis.
All topoisomerases function by generating transient breaks in the backbone of DNA (6, 7, 9, 12, 13). These enzymes utilize a transesterification mechanism that conserves the energy of the sugar-phosphate bond by transferring the newly generated DNA terminus to an active-site tyrosine residue via a tyrosine–phosphate bond. This covalent enzyme-cleaved DNA intermediate is known as the cleavage complex.
Topoisomerases are classified by the number of strands that they cleave during their catalytic event (6, 7, 9, 12, 13). Type I topoisomerases act by creating a transient nick in one strand of the double helix. This mechanism allows them to regulate DNA under- and overwinding but will not allow them to remove knots or tangles from the genetic material. Type IA enzymes link to the 5′-terminus of the cleaved DNA and mediate passage of the opposite strand through the break. Type IB enzymes link to the 3′-terminus of the cleaved DNA and allow controlled rotation about the break. In contrast, type II topoisomerases function by passing an intact double helix through a transient double-stranded break that they generate in a separate segment of DNA. As a result, these latter enzymes are able to control all aspects of DNA topology. The topoisomerase encoded by the variola virus is a type IB enzyme.
Proliferating cells cannot survive without the activities of topoisomerases (5–9). However, because these enzymes generate DNA strand breaks as requisite intermediates in their catalytic cycles, they pose a threat to genomic integrity every time they function (6, 7, 9, 12). This latter aspect of topoisomerases has been exploited to develop a number of important cytotoxic pharmaceuticals. Human topoisomerase IB is the target for important new anticancer drugs derived from the natural product camptothecin (13–15). Human type II topoisomerases are targets for several widely used anticancer drugs, including etoposide and doxorubicin (6, 14, 16). Finally, prokaryotic type II topoisomerases are targets for quinolones, the most efficacious and broad-spectrum class of oral antibacterials currently in clinical use (17, 18).
Although topoisomerase-targeted drugs represent a structurally diverse group of compounds, they share a common mechanism. All of these agents act by increasing the cellular concentration of covalent enzyme–DNA cleavage complexes. When nucleic acid tracking systems (including replication or transcription complexes) attempt to traverse these proteinaceous roadblocks in the genetic material, the resulting strand breaks initiate multiple damage response pathways that can culminate in cell death (6, 19, 20). Because topoisomerase-targeted drugs convert these enzymes from essential proteins to potent cellular toxins that fragment the genetic material, these drugs are referred to as topoisomerase poisons.
It is unusual for viruses to express their own topoisomerases. Of the known viral families with eukaryotic hosts, only five encode topoisomerase genes (21, 22) (Table 1⇓). Collectively, these viruses are commonly referred to as nucleocytoplasmic large DNA viruses (NCLDV) and probably have a common evolutionary ancestor (21, 22). At the present time, the only viral topoisomerase proteins that have been characterized are the Poxviridae (primarily vaccinia virus) (23) and Mimiviridae type IB enzymes (24), and the Phycodnaviridae (Chlorella virus) type II enzyme (25–27).
It is not known why these viral families encode their own DNA topoisomerases. Unlike other viruses, all of the NCLDVs possess large double-stranded DNA genomes that are characterized by either hairpin telomeres or circularly permuted ends (22, 31, 32). In addition, they encode many of the proteins required for viral replication and transcription and replicate in part or entirely in the host cytoplasm (22, 31, 32). The above information notwithstanding, the physiological functions of virally encoded topoisomerases are poorly understood. The only viral system for which there are biological data in this regard is vaccinia virus (33). Vaccinia topoisomerase IB was long thought to be essential for the viral life cycle and was believed to participate in DNA replication, recombination, or concatemer resolution (33). However, a knockout mutant of the vaccinia enzyme revealed that it was not essential for viral replication in cultured cells (although deletion significantly reduced infectivity). The primary physiological function of vaccinia topoisomerase IB appears to be to increase early transcription within the virus core in the cytoplasm of infected cells (33).
Most of what we understand about Poxviridae topoisomerase comes from extensive biochemical and structural studies of the vaccinia enzyme (23). Several features distinguish this viral type IB topoisomerase from its eukaryotic counterpart. First, the Poxviridae enzyme, with a molecular mass of only ~32 kDa, is the smallest known topoisomerase, being less than one-third the size human topoisomerase IB (molecular mass ~100 kDa) (7). Second, it maintains higher levels of covalent enzyme-cleaved DNA intermediates during its catalytic cycle than does the eukaryotic type IB enzyme. Third, although human topoisomerase IB is exquisitely sensitive to camptothecin and related anticancer agents (7, 13, 14), Poxviridae topoisomerase is refractory to the drug. Curiously, small size, robust DNA cleavage, and a lack of sensitivity to established topoisomerase poisons also distinguish viral (e.g., Chlorella virus) topoisomerase II from the eukaryotic type II enzymes (25–27). Thus, these features may be important for the biology of the viral enzymes. Finally, although all eukaryotic topoisomerases (both type I and II) display only a weak consensus sequence for DNA cleavage, Poxviridae topoisomerase IB is highly sequence specific. The site of DNA scission mediated by the viral enzyme is 5′-(T/ C)CCTT↓-3′, where the arrow represents the scissile bond (23).
Despite the wealth of biochemical information on vaccinia topoisomerase IB, the field has lacked a structural framework to examine the interactions of the Poxviridae enzyme with DNA. A recent study by Perry et al. (4) finally has added this critical piece of the puzzle. The authors solved the crystal structure of the non-covalent variola topoisomerase IB–DNA complex, as well as that of the covalent enzyme–DNA cleavage complex. These structures provide an explanation for some of the unique features of Poxviridae topoisomerase IB.
Figure 1⇓ compares the structure of the variola topoisomerase IB–DNA cleavage complex (4) with that of the human topoisomerase IB–DNA cleavage complex formed in the presence of camptothecin (34). Camptothecin is modeled into the viral complex. There are two striking differences between the structures. The first difference forms the basis for the DNA sequence specificity of Poxviridae topoisomerase IB: the β5 strand in the N-terminal domain and the α5 helix in the catalytic domain of the variola enzyme form an extensive network of major-groove contacts within the DNA cleavage sequence. Interactions with the β5 strand specify the bases that are in the +2, +3, and +4 positions 5′ to the scissile bond, and interactions with the α5 helix specify the bases in the +4 and +5 positions. In contrast, the equivalent β strand in the structure of human topoisomerase IB lies ~3 Å outside of the major groove and, therefore, does not form direct contacts to the bases. Similarly, the equivalent α helix in the human enzyme interacts primarily with the sugar-phosphate backbone of the double helix but not with the bases.
The second difference suggests the underlying reason why Poxviridae topoisomerase IB is insensitive to camptothecin. As seen in the structure of human topoisomerase IB, camptothecin binds between the +1 and −1 bases on the scissile strand of DNA. The drug prevents ligation by placing a physical barrier between the terminal 5′-OH and 3′-PO4 moieties. Camptothecin is held in place by extensive contacts in the N-terminal domain of the human enzyme, which encapsulates the anticancer agent. In marked contrast, variola topoisomerase IB has no corresponding protein domain. Consequently, camptothecin modeled into the viral enzyme–DNA cleavage complex is largely exposed to the solvent in this region and lacks critical protein contacts.
The structure of the variola topoisomerase IB–DNA cleavage complex, together with the unique biochemical features of the enzyme, provides a starting point for the rational design of anti-smallpox therapeutics. Because Poxviridae topoisomerase IB is not essential for the viral life cycle (33), the preferred strategy would be to develop agents that act as topoisomerase poisons rather than catalytic inhibitors. By trapping the cleavage complex, drug treatment could either fragment the viral genome or block early transcription by placing a covalently attached protein in the path of RNA polymerase.
The minimal nature of variola topoisomerase IB provides a mixed blessing for potential drug development. On the one hand, it is clear that the viral enzyme can accommodate bulky drug substituents that would not be tolerated by human topoisomerase IB. This provides the potential for a manageable therapeutic window. On the other hand, it is not a given that the viral enzyme will form enough contacts with a drug to provide the necessary affinity and specificity to generate an effective therapeutic. It may be possible to overcome this latter pitfall. Indeed, a recent high-throughput screen identified nineteen compounds with activity against vaccinia topoisomerase IB with IC50 values in the low micromolar range (35). All of the compounds were catalytic inhibitors and did not increase levels of cleavage complexes. However, they acted within the enzyme-DNA complex (i.e., they did not function by disrupting the binding of DNA) and displayed no inhibition of human topoisomerase IB, even at concentrations ~1,000-fold greater than their IC50 values. Thus, in principle, it should be possible to design agents that preferentially interact with Poxviridae topoisomerase IB over the human type IB enzyme.
An alternative approach would be to design a drug that is directed to the active site of variola topoisomerase through its specific DNA recognition sequence. In this regard, intercalating polycyclic aromatic hydrocarbon–DNA adducts that flank the scissile phosphodiester bond act as poisons of vaccinia topoisom-erase IB (36). Moreover, conjugation of camptothecin to specific triplex-forming oligonucleotides induces DNA cleavage by human topoisomerase IB at specific sites in vitro and in treated cells, and decreases transcription at these sites in model cellular systems (37, 38). Thus, it may be possible to apply these same approaches to generate conjugated drugs that act specifically at the recognition sequence of the variola enzyme.
In summary, because of the ease of infection and high degree of fatality associated with the variola virus, smallpox is a disease with the potential to be a devastating biological weapon. Unfortunately, at the present time there are no specific treatments for the disease. The recent study by Perry et al. on the structure of the variola topoisomerase IB–DNA complex (4) provides a structural context that may facilitate the design of novel therapeutics with activity against smallpox. doi:10.1124/mi.6.5.4
Topoisomerases Encoded by Nucleocytoplasmic Large Viruses
Crystal structures of the variola and human topoisomerase IB-DNA cleavage complexes. A. The variola topoisomerase IB–DNA cleavage complex. The anticancer drug camptothecin has been modeled into the complex. B. The human topoisomerase IB–DNA cleavage complex crystallized in the presence of camptothecin. Adapted from Perry et al. (4) Reprinted from
- © American Society for Pharmacology and Experimental Theraputics 2006
References
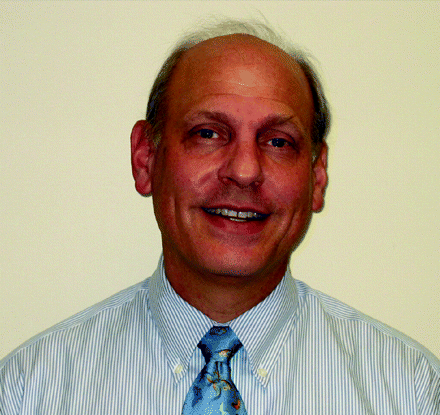
Neil Osheroff, PhD, is a Professor of Biochemistry and Medicine (Hematology/Oncology) and holds the John G. Coniglio Chair in Biochemistry at the Vanderbilt University School of Medicine. He received his bachelor’s degree in Chemistry from Hobart College and his doctoral degree in Biochemistry and Molecular Biology from Northwestern University. Following a postdoctoral fellowship in Biochemistry at the Stanford University School of Medicine, he joined the Vanderbilt faculty in 1983. His laboratory has a long-standing interest in the mechanism and biological functions of DNA topoisomerases, anticancer and antibacterial drugs that target these enzymes, the potential roles of topoisomerases in initiating leukemia, and DNA mutagenesis and repair. E-mail: neil.osheroff{at}vanderbilt.edu; fax 615 343-1166.