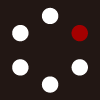
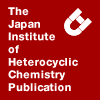
HETEROCYCLES
An International Journal for Reviews and Communications in Heterocyclic ChemistryWeb Edition ISSN: 1881-0942
Published online by The Japan Institute of Heterocyclic Chemistry
e-Journal
Full Text HTML
Received, 25th July, 2008, Accepted, 24th September, 2008, Published online, 25th September, 2008.
DOI: 10.3987/COM-08-S(F)59
■ Studies toward the Total Synthesis of Carba Analogue of Motif of M. TB Cell Wall AG Complex
Mukund K. Gurjar,* Challa Nageswar Reddy, Uttam R. Kalkote, and Mukund S. Chorghade
Division of Organic Chemistry, National Chemical Laboratory, Pashan Road, Pune 411 008, India
Abstract
Herein we describe the synthesis of the carba analogue of motif C of arabinogalactan complex present in M. tuberculosis cell wall. Pd(0) catalyzed allylic alkylation and Fraser-Reid’s glycosidation are the two key reactions that were employed for the synthesis of central glycosyl accepter unit and the glycosylation respectively.The mycolic arabinogalactan (AG) complex present on the surface of the cell wall of Mycobacterium tuberculosis has unique structural features unknown in actinomycetes. The furanoside rings of AG complex are conformationally more mobile than the pyranosides and are largely linked through primary hydroxyl groups. These characteristics enable the crowded AG complex to adopt a structure in which mycolic acids are closely arranged in parallel arrays.1 The AG complex is critical for the survival of M. tuberculosis. The hydrophobic AG complex acts as a strong barrier for the passage of antibiotics into the cell and therefore, plays an important role in developing resistance of mycobacteria to many antibiotics. The drug ethambutol blocks the biosynthetic pathway of arabinose. The inhibition of biosynthetic pathway, involved in displacement of M. tuberculosis cells, is considered as an attractive strategy for drug discovery and development against M. tuberculosis. The structures of the oligosaccharides present on various motifs of AG complex have been elucidated and their synthesis has dominated the area in recent times.
There has been increasing interest in the synthesis of “glycoconjugates” containing carbasugar residues for use as potential therapeutic agents. It is believed that such species will be more efficacious than their glycoside counterparts due to increased acidic and metabolic stability. The approach has already been validated by many carbasugar-containing nucleoside analogues possessing demonstrated antiviral activity.2 Oligosaccharide analogues containing carbasugar residues have been shown to be competent glycosyltranferases: we postulated that arabinosyltransferase inhibitors containing carbasugar residues would manifest similar action and constitute attractive synthetic targets.3
The logical extension of this effort was to design a synthetic strategy to assemble carba analogue of disaccharide of motif C. The synthetic target of interest is 5-O-(4a-carba-β-D-arabinofuranosyl)- α-D-arabinofuranoside (2) as shown in Figure 1.
As depicted in the retrosynthetic plan (Scheme 1), the acylated disaccharide 3 can be obtained by O-glycosidation between glycosyl donor 4 and glycosyl acceptor 5 with Fraser Reid glycosidation protocol. The pentenyl glycoside donor 4 could be derived from D-arabinose. The carba sugar4 derivative 5 can be elaborated from 6 through m-CPBA epoxidation followed by acid catalysed regioselective epoxide opening.
The synthesis of homoallylic alcohol 6 was planned from 7 using a Pd(0) mediated allylic alkylation with phenylsulfonylnitromethane. Cyclopentadiene 8 was chosen as starting point for the synthesis of 7 through enzymatic desymmetrization and protecting group manipulations.
The synthetic sequence started with cyclopentadiene (8) to obtain the known cis-2-cyclopentene-1,4-diol (9) as reported by Kaneko et al.5 Cyclopentadiene (8) was treated with molecular oxygen in the presence of thiourea and Rose Bengal in methanol and irradiated with a 450 W high pressure mercury immersion lamp to give cis-2-cyclopentene-1,4-diol (9) in quantitative yield. Diol 9 was acetylated with acetic anhydride in pyridine using a catalytic amount of DMAP to give cis-2-cyclopentene-1,4-diacetate (10). The meso-di-acetate 10 was enzymatically hydrolysed with Trichosporone beigelii (NCIM 3326) in 0.1M sodium phosphate buffer (pH 7) with 10% v/v ethanol to give 4-R-hydroxycyclopent-2-en-1-S-acetate (11) optical purity > 98% (Scheme 2).6
We then proceeded towards the synthesis of glycosyl acceptor 5. The hydroxyl acetate 11 was treated with BnBr and Ag2O in dry CH2Cl2 to afford benzylic derivative 12 (Scheme 3). The 1H NMR and 13C NMR spectra were in full agreement with the assigned structure. In 1H NMR spectrum the benzylic protons were observed at δ 4.52-4.59 as two doublets and in 13C NMR the carbonyl carbon was observed at δ 170.5. The benzylic derivative 12 was subjected to deacetylation by using K2CO3 in methanol–H2O (3:1) to furnish 13. In the 1H NMR spectrum of 13, the olefinic protons were observed at δ 6.02 and in the 13C NMR spectrum, the benzylic carbon resonated at δ 70.8.
The free hydroxyl group in 13 was protected as its benzoate by using benzoyl chloride in the presence of Et3N in CH2Cl2 to afford 7 in 94% yield (Scheme 4). The introduction of hydroxymethyl functionality at the benzoate position was accomplished in a highly stereospecific manner through palladium (0) catalyzed allylic alkylation.7 Thus, the benzoate 7 was subjected to a palladium (0) catalyzed cross-coupling reaction with a phenylsulfonylnitromethane anion to afford 14 as a 1:1 diastereomeric mixture almost quantitatively. The NMR analysis and other analytical data were in full agreement with the assigned structure. Further, in the IR spectrum of compound 14, the characteristic absorption for NO2 group was observed at 1560 and 1345 cm-1. Treatment of 14 with tetramethylguanidine salt and tetrabutylammonium oxone (TBA-Oxone)8 in methanol/CH2Cl2 buffered with sodium carbonate gave the methyl ester 15 in 43% yield. The methyl ester 15 was treated with DIBAL-H in CH2Cl2 at –78 oC to procure the hydroxymethyl derivative 6 in 68% yield. In the 1H NMR spectrum, the methyl ester peak disappeared and two methylene protons were observed at δ 3.62 as a doublet. Moreover, the 13C NMR spectrum revealed the absence of carbonyl peak and a triplet carbon at δ 65.0.
Having synthesized the hydroxymethyl derivative 6, our next objective was to introduce the trans diol originating from the ring olefin of 6. Towards this goal, treatment of 6 with m-CPBA gave a diastereomeric mixture of epoxides (β-and α-epoxides) in 7:3 ratio, which were separated by silica gel column chromatography (Scheme 5).
In 1H NMR spectrum of 16 the epoxide protons were observed as a doublet at δ 3.49; the same resonance for 17 was observed at δ 3.53 as a singlet. To determine the relative configuration of epoxides 16 and 17, both the epoxides were independently treated with PivCl and Et3N in CH2Cl2 to furnish the corresponding pivaloate ester derivatives 18 and 19. Further, COSY/NOESY experiments were carried out on epoxides 18 and 19 and the relative stereochemistry was assigned based on observed spatial interactions (Figure 2).
In the NOESY spectrum of 18, the observed spatial interactions between H-C(1) and H-C(2), H-C(1) and Hα-C(4a), H-C(3) and H-C(4) established the assigned structure. Along similar lines, the observed correlations for 19, i e. H-C(1) and Hα-C(4a), H-C(2) and Hβ-C(4a), H-C(3) and Hβ-C(4a) supported the assigned structure.
We proceeded with β-epoxide 18 for regioselective epoxide opening.9 Epoxide 18 was treated with catalytic amount of HClO4 in DMSO-H2O (3:1) solvent mixture to afford the diol 20 quantitatively. The structure of 20 was fully confirmed by 1H and 13C NMR spectral analysis. Further, the IR spectrum of 20 revealed the presence of –OH group with absorption at 3432 cm-1. To confirm whether the epoxide opening took place at C2 or C3 position, 20 was subjected to debenzylation using Pd/C (catalytic amount) in methanol under H2 pressure to give intermediate triol (Scheme 6). The syn-diol functionality of triol was protected as its acetonide using 2,2-dimethoxypropane in acetone with catalytic amount of p-TSA to give acetonide 21. In the 1H NMR spectrum, the two isopropylidene methyl groups were observed at δ 1.29 and 1.48 as two distinct singlets. Further, in 13C NMR spectrum, the quaternary isopropylidene carbon was observed at δ 111.8. Rest of the spectral and analytical data are supportive of the assigned structure.
The stereochemistry of 21 was unambiguously confirmed by its COSY/NOESY spectral analysis (Figure 3). The observed spatial interaction between H-C(1) and H-C(2), H-C(1) and Hα-C(4a), Hβ-C(3) and Hβ-C(4a) confirmed the assigned structure of 21. Rest of the correlations are in accordance with the given structure of 21 also unambiguously confirms the stereochemistry of epoxide 18.
The pivaloate 20 was subjected to DIBAL-H reduction in CH2Cl2 at -78 oC to give triol 22 in 74% yield. Further, in the IR spectra the characteristic –OH stretch was observed at 3369 cm-1. Primary hydroxyl functionality of triol 22 was protected as its TBS ether using TBS-Cl and imidazole in CH2Cl2 to obtain diol 23 quantitatively (Scheme 7). The diol 23 was acetylated using Ac2O and pyridine in CH2Cl2 to furnish the diacetate 24. Diacetate 24 was subjected to TBS deprotection using catalytic 0.8% H2SO4 to afford the glycosyl acceptor 5 in 63% yield. Spectral and analytical data for 5 are supportive of the assigned structure.
Similarly, the α-epoxide 19 was opened under the same reaction conditions as described for epoxide 18. Surprisingly, it gave the epoxide opened product with pivaloyl group migration from primary to secondary hydroxyl group. This was confirmed by extensive spectral studies of 25 (Scheme 8).
COSY/NOESY experiments were performed on diol 25 and the observed correlations were shown in Figure 4.
The observed spatial interactions between H-C(1) and H-C(4), H-C(1) and Hα-C(4a), H-C(3) and H-C(4), Hβ-C(4a) and H-C(5) confirmed the assigned structure of 25. Rest of the correlations are in complete accord with the structure, which unambiguously confirms the stereochemistry of epoxide 25.
Our next endeavor was to synthesize the n-pentenyl glycosyl donor 4 starting from D-arabinose. Methyl D-arabinofuranoside (26) was prepared from D-arabinose and methanolic HCl at rt, which was subsequently treated with Ac2O, pyridine to afford methyl 2,3,5-tri-O-acetyl-D-arabinofuranoside (27). The per-O-acetyl-D-arabinose (28) was prepared from 27 with Ac2O/AcOH/H2SO4 in quantitative yield. Treatment of 28 with 4-penten-1-ol with a catalytic amount of BF3·OEt2 and 4Å MS powder in CH2Cl2 at rt furnished pentenyl glycosyl donor 4 (Scheme 9).
With both glycosyl donor (4) and glycosyl acceptor (5) in hand, we planned for the crucial O-glycosylation. as per n-pentenyl mediated O-glycosylation first disclosed by Fraser-Reid.10
The Fraser-Reid glycosidation reaction between glycosyl donor 4 and glycosyl acceptor 5 in the presence of NIS, TfOH (catalytic) and 4Å MS powder in CH2Cl2 at rt gave the disaccharide 29 in 46% yield (Scheme 10).
The 1,2-trans configuration of disaccharide of 29 was evident from its 1H and 13C NMR spectra studies. In the 1H NMR spectrum of 29, the anomeric proton was observed at δ 5.01 as a characteristic singlet. Further, the location of the signal due to C-1’ at 105.3 ppm in the 13C NMR spectrum confirmed the α-linkage at the newly formed glycosidic bond and satisfactory elemental analysis supported the structure of 29. Finally the disaccharide 29 was subjected to debenzylation, under hydrogen, using Pd(OH)2/C in MeOH at rt to give the alcohol which was subsequently acetylated using Ac2O and pyridine to give hexa-acetyl disaccharide 3 in 78% overall yield (Scheme 11).
The structure of 3 was unequivocally confirmed by its spectral and analytical data. In the 1H NMR spectrum, the anomeric proton was observed at δ 4.94 as a singlet and rest of the spectrum was in full accordance with the structure. In the 13C NMR spectrum the characteristic C-1 carbon resonated at δ 105.23. The six carbonyl carbons were observed at δ 169.6, 170.0, 170.0, 170.2, 170.3, 170.6.
EXPERIMENTAL
1H NMR spectra were recorded on AV-200 MHz, AV-400 MHz, and DRX-500 MHz spectrometer using tetramethylsilane (TMS) as an internal standard. Chemical shifts have been expressed in ppm units downfield from TMS 13C NMR spectra were recorded on AV-50 MHz, AV-100 MHz, and DRX-125 MHz spectrometer. EI Mass spectra were recorded on Finnegan MAT-1020 spectrometer at 70 eV using a direct inlet system. X-Ray Crystal data were collected on Bruker SMART APEX CCD diffractometer using MoKα radiation with fine focus tube with 50 kV and 30 mA.Infrared spectra were scanned on Shimadzu IR 470 and Perkin-Elmer 683 or 1310 spectrometers with sodium chloride optics and are measured in cm-1.Optical rotations were measured with a JASCO DIP 370 digital polarimeter. All reactions are monitored by Thin Layer chromatography (TLC) carried out on 0.25 mm E-Merck silica gel plates (60F-254) with UV light, I2, and anisaldehyde in ethanol as developing agents.
(1S,4R)-4-(Benzyloxy)cyclopent-2-enyl acetate (12).
To a solution of 1 [α]D –69.3 ( c 1, CHCl3) and ee > 99% (6 g, 45.3 mmol) in CH2Cl2 (720 mL) were added silver oxide (29.4 g, 126.8 mmol) and benzyl bromide (10.8 g, 63.4 mmol) and the reaction mixture was stirred at rt for 12 h. The mixture was filtered through a Celite bed. The filtrate was dried over Na2SO4, concentrated and the crude was purified by column chromatography (10% EtOAc in petroleum ether) to yield 12 (9.2 g, 93%) as colorless oil. [α]D –12.7 (c 1.0, CHCl3). IR (CHCl3): 3064, 2860, 1735, 1454, 1364, 1240, 1026, 909, 738, 698, 608 cm-1. 1H NMR (CDCl3, 500 MHz) δ: 1.75 (dt, J = 4.6, 14.2 Hz, 1H), 2.05 (s, 3H), 2.76 (dt, J = 7.3, 14.7 Hz, 1H), 4.48-4.50 (m, 1H), 4.55 (qt, J = 11.7 Hz, J = 22.0 Hz, 2H), 5.48–5.50 (m, 1H), 5.98–5.99 (m, 1H), 6.11–6.12 (m, 1H), 7.25–7.33 (m, 5H). 13C NMR (CDCl3, 125 MHz) δ: 21.1 (q), 37.5 (t), 70.9 (t), 76.8 (d), 81.1 (d), 127.5 (d), 127.6 (d), 128.3 (d), 132.8 (d), 136.1 (d), 138.2 (s), 170.6 (s). Anal. Calcd for C14H16O3: C, 72.39; H, 6.94. Found: C, 72.25; H, 7.20.
(1S,4R)-4-(Benzyloxy)cyclopent-2-enol (13).
A suspension of 12 (4.0 g, 17.2 mmol), K2CO3 (3.65 g, 34.5 mmol) in MeOH:H2O (50 mL, 3:1) was stirred at rt for 3 h. The mixture was concentrated and extracted with CH2Cl2 (60 mL). The aqueous layer was back extracted with CH2Cl2 (100 mL). The combined organic extracts were dried over Na2SO4, filtered and concentrated. The crude product was purified by column chromatography (20% EtOAc in petroleum ether) to give 13 (3 g, 92%) as colorless oil. [α]D +21.9 (c 1.0, CHCl3): IR (CHCl3): 3387, 2862, 1454, 1359, 1072, 750, 698 cm-1. 1H NMR (CDCl3, 200 MHz) δ: 1.65 (dt, J = 3.9, 14.1 Hz, 1H), 2.23 (br s, 1H), 2.64 (dt, J = 7.1, 14.2 Hz, 1H), 4.39–4.64 (m, 4H), 6.02 (s, 2H), 7.25–7.35 (m, 5H). 13C NMR (CDCl3, 50 MHz) δ: 40.6 (t), 70.9 (t), 74.4 (d), 81.5 (d), 127.5 (d), 127.7 (d), 128.3 (d), 133.4 (d), 137.3 (d), 138.0 (s). Anal. Calcd for C12H14O2: C, 75.76; H, 7.42. Found: C, 75.74; H, 7.39.
(1S,4R)-4-(Benzyloxy)cyclopent-2-enyl benzoate (7).
To a solution of 13 (5 g, 26.3 mmol) in CH2Cl2 (100 mL) were added triethylamine (5.3 g, 52.6 mmol) was added benzoyl chloride (5.5 g, 39.5 mmol) slowly. The reaction mixture was stirred at rt for 12 h. The mixture was extracted with CH2Cl2 (100 mL), dried over Na2SO4, filtered and concentrated. The crude was purified by column chromatography (10% EtOAc in petroleum ether) to produce 7 (7.2 g, 94%) as colorless oil. [α]D –45.5 (c 1.0, CHCl3). IR (CHCl3): 3019, 1713, 1274, 1215, 756, 668 cm-1. 1H NMR (CDCl3, 500 MHz) δ: 1.91 (dt, J = 4.3, 14.2 Hz, 1H), 2.9 (dt, J = 7.3, 14.2 Hz, 1H), 4.55–4.62(m, 3H), 5.75–5.77 (m, 1H), 6.10–6.18 (m, 2H), 7.26-7.35(m, 4H), 7.42 (t, J = 7.3 Hz, 2H), 7.53 (q, J = 7.3, 13.7 Hz, 2H), 8.04 (d, J = 7.3 Hz), 4.55–4.62 (m, 3H), 5.75-5.77 (m, 1H), 6.10–6.18(m, 2H) 7.26-7.35(m, 4H), 7.42 (t, J = 7.3 Hz, 2H), 7.53 (q, J = 7.3, 13.7 Hz, 2H), 8.04 (d, J = 7.3 Hz, 2H). Anal. Calcd for C19H18O3: C, 77.53; H, 6.16. Found: C, 77.48; H, 6.57.
(((1S,4R)-4-(Benzyloxy)cyclopent-2-enyl)(nitro)methylsulfonyl)benzene (14).
To a deoxygenated solution of triphenylphosphine (89 mg, 0.34 mmol) in THF (20 mL) was added Pd2(dba)3·CHCl3 (35 mg, 0.034 mmol). The mixture was stirred for 20 min. It was then added to a deoxygenated solution of monobenzoate 7 (1 g, 3.4 mmol), phenylsulfonylnitromethane (821 mg, 4.1 mmol) and triethylamine (860 mg, 8.5 mmol) in THF (20 mL). After stirring for 12 h the reaction mixture was diluted with CHCl2 (25 mL) and washed with water (100 mL). The aqueous phase was extracted with CHCl2 (250 mL), and the combined organic layers were dried over Na2SO4 and concentrated. The residue was purified by column chromatography (6% EtOAc in petroleum ether) to give 14 (0.9 g, 72%) as 1:1 diastereomeric mixture. IR (CHCl3): 3030, 2864, 1560, 1449, 1345, 1082, 755, 686 cm-1. 1H NMR (CDCl3, 500 MHz) δ: 1.62 (dt, J = 4.1, 13.7 Hz, 1H), 2.27 (dt, J = 3.6, 14.2 Hz, 1H), 2.36 (dt, J = 6.8, 14.2 Hz, 1H), 2.50 (dt, J = 7.3, 14.6 Hz, 1H), 3.50-3.61 (m, 2H), 4.45-4.53 (m, 4H), 4.57-4.60 (m, 2H), 5.47 (qt, J = 10.5, 16.9 Hz, 2H), 5.63-5.64 (m, 1H), 6.13-6.16 (m, 2H), 6.30-6.31 (m, 1H), 7.26–7.36 (m, 9H), 7.60 (t, J = 7.5, 4H), 7.75 (t, J = 7.3 Hz, 2H), 7.89-7.91 (m, 4H). 13C NMR (CDCl3, 125 MHz) δ: 33.6 (d), 34.6 (d), 43.2 (d), 43.51 (d), 71.38 (d), 81.5 (d), 82.8 (d), 96.2 (d), 105.3 (s), 105.6 (s), 127.8 (d), 128.4 (d), 129.4 (d), 129.96 (d), 129.99 (d), 131.6 (d), 131.9 (d),135.3 (s), 135.9 (s), 137.06 (s). Anal. Calcd for C19H19NO5S: C, 61.11; H, 5.13; N, 3.75; S, 8.59. Found: C, 61.14; H, 5.09; N, 3.71; S, 8.60.
(1S,4R)-Methyl 4-(benzyloxy)cyclopent-2-enecarboxylate (15).
To an ice cold solution of nitrosulfone 14 (2.0 g, 5.36 mmol) in MeOH (40 mL) was added tetramethylguanidine (0.93 g, 8.1 mmol) and the mixture was stirred a 0 oC for 15 min. This was followed by addition of TBA-Oxone (22.98 g, 26.8 mmol), Na2CO3 (2.84 g, 26.8 mmol) and CH2Cl2 (50 mL). The solution was stirred at rt for 16 h. It was then diluted with CHCl3 and washed with water (100 mL). The aqueous layer was extracted with CHCl3 (200 mL), and the combined CHCl3 layers were dried over Na2SO4, and concentrated. The residue was purified by column chromatography (6% EtOAc in petroleum ether) to give 15 (0.53 g, 43%) as colourless oil. [α]D –46.8 (c 1.0, CHCl3). IR (CHCl3): 3019, 2863, 1733, 1360, 1216, 1068, 756, 667 cm-1. 1H NMR (CDCl3, 500 MHz) δ: 2.13 (dt, J = 6.4, 13.7 Hz, 1H), 2.51 (dt, J = 8.7, 13.7 Hz, 1H), 3.43-3.46 (m, 1H), 3.71 (s, 3H), 4.52 (d, J = 11.9 Hz, 1H), 4.57 (d, J = 11.9 Hz, 1H), 4.64 (t, J = 6.4 Hz, 1H), 5.96-5.99 (m, 2H), 7.24-7.34 (m, 5H). 13C NMR (CDCl3, 125 MHz) δ: 33.3 (t), 48.9 (q), 51.9 (d), 70.8 (t), 83.3 (d), 127.5 (d), 127.8 (d), 128.4 (d), 132.0 (d), 133.9 (d), 138.7 (s), 173.5 (s). Anal. Calcd for C14H16O3: C, 72.39; H, 6.94. Found: C, 72.34; H, 6.92.
((1S,4R)-4-(Benzyloxy)cyclopent-2-enyl)methanol (6).
At –78 oC a solution of ester 15 (1.0 g, 4.31 mmol) in CH2Cl2 (20 mL) was treated with DIBAL-H (1.53 g, 10.77 mmol) –78 oC for 1 h and quenched with saturated solution of sodium potassium tartarate (2 mL) at the same temperature. The mixture was warmed to rt and stirred for 3 h. The organic phase was separated and the aqueous phase was extracted with CH2Cl2. The combined extracts were dried over Na2SO4 and concentrated. The crude product was purified by column chromatography (15% EtOAc in petroleum ether) to yield 6 (0.6 g, 68%) as colourless oil. [α]D +21.9 (c 1, CHCl3). IR (CHCl3): 3400, 2865, 1364, 1091, 1043, 736, 697 cm-1. 1H NMR (CDCl3, 500 MHz) δ: 1.57 (br s, 1H), 1.68 (dt, J = 3.2, 13.7 Hz, 1H), 2.28 (dt, J = 7.3, 14.2 Hz, 1H), 2.86 (br s, 1H), 3.62 (d, J = 4.6 Hz, 2H), 4.52-4.58 (m, 3H), 5.95-6.02 (m, 2H), 7.24-7.32 (m, 5H).13C NMR (CDCl3, 125 MHz) δ: 33.9 (t), 46.8 (d), 65.0 (t), 70.9 (t), 82.9 (d), 127.6 (d), 127.7 (d), 128.4 (d), 132.6 (d), 136.8 (d), 138.4 (s). Anal. Calcd for C13H16O2: C, 76.44; H, 7.90. Found: C, 75.47; H, 7.84.
((1S,2R,4R,5R)-4-(Benzyloxy)-6-oxabicyclo[3.1.0]hexan-2-yl)methanol (16).
Epoxidation of cyclopentene 6.
To a solution of 6 (1 g, 4.9 mmol) in CH2Cl2 (16 mL), was added m-CPBA (2.11 g, 12.25 mmol) at 0 oC and the mixture was stirred at rt for 12 h. Precipitated m-chloroperbenzoic acid was filtered. The filtrate was concentrated and the residue was purified by column chromatography (40% EtOAc in petroleum ether) to obtain 16 (570 mg, 52.5%) and 17 (240 g, 22.5%) as colorless liquids. [α]D –10.1 (c 1.2, CHCl3). IR (CHCl3): 3412, 3019, 1716, 1215, 757, 669 cm-1. 1H NMR (CDCl3, 500 MHz) δ: 1.17-1.23 (m, 1H), 1.86-1.91 (m, 1H), 2.11-2.16 (m, 1H), 3.49 (d, J = 15.6 Hz, 2H), 3.75 (d, J = 6.4, 2H), 4.05 (t, J = 7.8 Hz, 1H), 4.61 (dt, J = 12.4, 16.0 Hz, 2H), 7.27-7.36 (m, 5H).13C NMR (CDCl3, 125 MHz) δ: 26.5 (t), 40.2 (d), 55.8 (d), 56.5 (d), 62.8 (t), 71.6 (t), 78.8 (d), 127.7 (d), 128.4 (d), 138.0 (s). Anal. Calcd for C13H16O3: C, 70.89; H, 7.32. Found: C, 70.87; H, 7.29.
((1S,2R,4R,5R)-4-(Benzyloxy)-6-oxabicyclo[3.1.0]hexan-2-yl)methanol (17).
[α]D –16.3 (c 1, CHCl3). IR (CHCl3): 3426, 3032, 2973, 2873, 1729, 1480, 1153, 840 cm-1. 1H NMR (CDCl3, 500 MHz) δ: 1.57 (d, J = 14.8 Hz, 1H), 1.80-1.94 (m, 1H), 2.43-2.51 (m, 1H), 2.90 (br s, 1H), 3.53 (s, 2H), 3.70 (dd, J = 4.30, 7.83 Hz, 2H), 4.09 (d, J = 5.5 Hz, 1H), 4.61 (d, J = 3.1 Hz, 2H), 7.31 (s, 5H). Anal. Calcd for C13H16O3: C, 70.89; H, 7.32. Found: C, 70.79; H, 7.12.
((1S,2R,4R,5R)-4-(Benzyloxy)-6-oxabicyclo[3.1.0]hexan-2-yl)methyl pivaloate (18).
To a solution of 16 (500 mg, 2.27 mmol) in CH2Cl2 (12 mL) at 0 oC were added pyridine (530 mg, 4.54 mmol) and pivaloyl chloride (550 mg, 4.54 mmol). The reaction mixture was stirred for 6 h and concentrated. The crude product was purified by column chromatography (10% EtOAc in petroleum ether) to yield 18 (640 mg, 88%) as colourless oil. [α]D –17.9 (c 1.0, CHCl3). IR (CHCl3): 3028, 2974, 1725, 1480, 1273, 1158, 754, 715 cm-1. 1H NMR (CDCl3, 500 MHz) δ: 1.21 (s, 10H), 1.88 (dt, J = 7.3, 12.8 Hz, 1H), 2.20-2.26 (m, 1H), 3.30 (d, J = 2.7 Hz, 1H), 3.50 (d, J = 2.7 Hz, 1H), 4.03 (t, J = 8.5 Hz, 1H), 4.07-4.10 (q, J = 8.2 Hz, 1H), 4.17-4.22 (m, 1H), 4.61 (s, 2H), 7.26–7.45 (m, 5H).13C NMR (CDCl3, 125 MHz) δ: 26.9 (t), 27.2 (q), 37.4 (d), 38.8 (s), 55.2 (d), 56.4 (d), 64.0 (t), 71.6 (t), 78.6 (d), 127.8 (d), 128.4 (d), 138.0 (s), 178.3 (s). Anal. Calcd for C18H24O4: C, 71.05; H, 7.89. Found: C, 71.09; H, 7.85.
((1R,2R,4R,5S)-4-(Benzyloxy)-6-oxabicyclo[3.1.0]hexan-2-yl)methyl pivalate (19).
To a solution of 17 (500 mg, 2.27 mmol) in CH2Cl2 (12 mL) at 0 oC were added pyridine (530 mg, 4.54 mmol) followed by pivaloyl chloride (550 mg, 4.54 mmol). The reaction mixture was stirred at rt for 6 h., concentrated and the crude was purified by column chromatography (10% EtOAc in petroleum ether) to yield 19 (640 mg, 88%) as colourless oil. [α]D –15.0 (c 1, CHCl3). IR (CHCl3): 3032, 2973, 2873, 1729, 1480, 1282, 1153, 1094, 840, 697 cm-1. 1H NMR (CDCl3, 500 MHz) δ: 1.15 (s, 9H), 1.48 (d, J = 14.6 Hz, 1H), 1.62-1.68 (m, 1H), 2.50 (q, J = 7.8 Hz, 1H), 3.43 (d, J = 6.9 Hz, 2H), 3.95-4.03 (m, 2H), 4.05-4.12 (m, 1H), 4.42 (d, J = 11.9 Hz, H), 4.51 (d, J = 11.5 Hz, 1H), 7.19-7.27 (m, 5H). 13C NMR (CDCl3, 125 MHz) δ: 27.3 (q), 30.1 (t), 38.4 (s), 56.9 (d), 58.5 (d), 64.9 (d), 71.7 (t), 78.3 (d), 127.6 (d), 127.8 (d), 128.5 (d), 128.5 (s), 129.7 (s), 133.3 (s), 138.0 (s), 177.9 (s). Anal. Calcd for C18H24O4: C, 71.03; H, 7.95. Found: C, 70.83; H, 7.75.
(1S,2S,3R,5R)-3-(Benzyloxy)-2-hydroxy-5-(hydroxymethyl)cyclopentyl pivalate (25).
To a solution of 19 (500 g, 1.54 mmol) in 3:1 of DMSO: H2O (10 mL) was added a catalytic amount of perchloric acid (70%) at 0 oC. The reaction mixture was stirred at rt for 16 h. The mixture was extracted with EtOAc (3 X 10 mL) and the combined organic layers were dried over Na2SO4, filtered and concentrated. The residue was purified by column chromatography (6% EtOAc in petroleum ether) to give 25 (370 mg, 76%) as colorless oil. [α]D –24.5 (c 1.0, CHCl3). IR (CHCl3): 3416, 3021, 1740, 1115, 736, 643 cm-1. 1H NMR (CDCl3, 500 MHz) δ: 1.23 (s, 9H), 1.62-1.70 (m, 1H), 2.16 (dt, J = 6.9, 13.1 Hz, 1H), 2.38-2.52 (m, 1H), 3.16 (br s, 1H), 3.63-3.66 (m, 2H) 3.81-3.9, (m, 1H), 4.05 (t, J = 3.9 Hz, 1H), 4.59 (d, J = 5.3 Hz, 2H), 4.77-4.82 (q, J = 3.3, 6.7 Hz, 1H), 7.28-7.34 (m, 5H). 13C NMR (CDCl3, 125 MHz) δ: 27.2 (q), 31.9 (t), 41.3 (d), 61.5 (t), 71.7 (t), 76.8 (d), 81.9 (d), 83.0 (d), 83.6 (d), 127.7 (d), 127.8 (d), 128.4 (d), 128.5 (d), 129.8 (s), 138.1 (s), 179.8 (s). Anal. Calcd for C18H26O5: C, 67.06; H, 8.13. Found: C, 66.98; H, 8.03.
((1R,2R,3S,4R)-4-(Benzyloxy)-2,3-dihydroxycyclopentyl)methyl pivaloate (20).
To a solution of 18 (500 mg, 1.54 mmol) in 3:1 of DMSO: H2O (10 mL) was added a catalytic amount of perchloric acid (70%) at 0 oC. The reaction mixture was stirred at rt for 16 h. The mixture was extracted with EtOAc (3 X 10 mL) and the combined organic layers were dried over Na2SO4, filtered and concentrated. The residue was purified by column chromatography (6% EtOAc in petroleum ether) to give 20 (370 mg, 76%) as colorless oil. [α]D –35.5 (c 0.4, CHCl3). IR (CHCl3): 3432, 3019, 1720, 1215, 757, 668 cm-1. 1H NMR (CDCl3, 500 MHz) δ: 1.13 (s, 9H), 1.45-1.59 (m, 1H), 1.76 (br s, 1H), 1.95-2.15 (m, 2H), 3.77-3.88 (m, 2H), 4.00-4.13 (m, 2H), 4.4 (d, J = 11.6 Hz, 1H), 4.56 (d, J = 11.6 Hz, 1H), 7.23-7.29 (m, 5H). 13C NMR (CDCl3, 125 MHz) δ: 27.1 (q), 29.8 (t), 38.8 (s), 40.7 (d), 65.5 (t), 71.4 (t), 76.7 (d), 78.4 (d), 78.5 (d), 127.7 (d), 127.9 (d), 128.4 (d), 137.5 (s), 178.6 (s). Anal. Calcd for C18H26O5: C, 67.08; H, 8.13. Found: C, 66.89; H, 7.15.
((3aS,4R,5R,6aR)-4-Hydroxy-2,2-dimethyltetrahydro-3aH-cyclopenta[d][1,3]dioxol-5-yl)methyl
pivaloate (21).
To a solution of 20 (100 mg) in MeOH (5 mL), was added Pd/C (10%, 50 mg) and stirred at rt under H2 atmosphere for 6 h. The mixture was filtered through a Celite bed and the filtrate was concentrated. The crude triol was used directly for the next reaction. To a solution of triol (80 mg, 0.34 mmol) in acetone (6 mL), were added dimethoxypropane (530 mg, 0.5 mmol) and a catalytic amount of p-TSA (5 mg). The solution was stirred at 0 oC for 0.5 h and quenched with solid NaHCO3 and the neutral reaction mixture was concentrated in vacuo. The residue obtained was purified by column chromatography (20% EtOAc in petroleum ether) to obtain 21 (60 mg, 75%) as colorless oil. [α]D –26.4 (c 1, CHCl3). IR (CHCl3): 3435, 1721, 1480, 1215, 928, 758, 669 cm-1. 1H NMR (CDCl3, 500 MHz) δ: 1.22 (s, 9H), 1.29 (s, 3H), 1.48 (s, 3H), 1.63 (br s, 1H), 1.14-1.70 (m, 1H), 2.16-2.24 (m, 2H), 3.96 (dd, J = 3.21, 5.04 Hz, 1H), 4.16 (dddd, J = 5.5, 11.0, 16.9 Hz, 2H), 4.39 (dd, J = 3.2, 6.9 Hz, 1H), 4.67-4.70 (m, 1H). 13C NMR (CDCl3, 125 MHz) δ: 24.4 (q), 26.8 (q), 27.3 (q), 32.5 (t), 46.1 (d), 64.3 (t), 78.5 (d), 78.7 (d), 87.0 (d), 111.77 (d), 178.4 (s), 178.8 (s). Anal. Calcd for C14H24O5: C, 61.74 H, 8.88. Found: C, 61.78; H, 8.85.
(1R,2S,3R,5R)-3-(Benzyloxy)-5-(hydroxymethyl)cyclopentane-1,2-diol (22).
A solution of 20 (400 mg, 1.24 mmol) in CH2Cl2 (15 mL) was cooled to –78 oC. DIBAL-H (440 mg, 3.1 mmol) was added dropwise and the mixture was stirred at –78 oC for 1 h. The reaction was quenched with saturated solution of sodium potassium tartarate (5 mL), warmed to rt and stirred for additional 3 h. Organic phase was separated and the aqueous phase was extracted with CH2Cl2. The combined organic extracts were dried over Na2SO4 and concentrated. The crude residue was purified by column chromatography (80% EtOAc in petroleum ether) to afford 22 (220 mg, 74.5%) as a colorless oil. [α]D –2.9 (c 1.0, MeOH). IR (CHCl3): 3369, 2930, 1454, 1046, 753, 698 cm-1. 1H NMR (CDCl3, 500 MHz) δ: 1.44 (br s, 1H), 1.87 (br s, 1H), 1.96-2.03 (m, 1H), 3.53 (t, J = 7.3 Hz, 1H), 3.62 (br s, 1H), 3.81 (br s, 2H), 3.82-3.96 (br s, 4H), 4.44 (d, J = 11.4 Hz, 1H), 4.53 (d, J = 11.4 Hz, 1H), 7.22-7.31 (m, 5H). 13C NMR (CDCl3, 125 MHz) δ: 29.4 (t), 43.4 (d), 65.0 (t), 71.6 (t), 77.5 (d), 78.6(d), 79.2 (d), 127.9 (d), 128.5 (d), 137.9 (s). Anal. Calcd for C13H18O4: C, 65.53; H, 7.61. Found: C, 65.37; H, 7.26.
(1R,2S,3R,5R)-3-(Benzyloxy)-5-((tert-butyldimethylsilyloxy)methyl)cyclopentane-1,2-diol (23).
To an ice-cold solution of 22 (500 mg, 2.1 mmol) in CH2Cl2 (12 mL), were added imidazole (420 mg, 6.3 mmol) and TBS-Cl (340 mg, 2.31 mmol). The reaction mixture was stirred for 0.5 h concentrated and purified by column chromatography (40% EtOAc in petroleum ether) to yield 23 (490 mg, 66%) as colorless oil. [α]D –18.9 (c 0.8, CHCl3). IR (CHCl3): 3424, 2929, 1255, 1216, 1067, 837, 758, 668 cm-1. 1H NMR (CDCl3, 500 MHz) δ: 0.06 (d, J = 1.9 Hz, 6H), 0.90 (s, 9H), 1.50 (dddd, J = 4.4, 8.8, 13.2, 18.1 Hz, 1H), 1.62 (br s, 1H), 1.9 (dt, J = 7.8, 16.6 Hz, 1H), 2.6 (dq, J = 6.3, 8.8, 15.1Hz, 1H), 2.69–2.71 (d, J = 5.8, 1H), 3.60 (t, J = 7.83 Hz, 1H), 3.74 (qt, J = 5.4, 9.8 Hz, 1H), 3.85 (br s, 1H), 3.89–3.94 (m, 2H), 4.49 (d, J = 11.7 Hz, 1H), 4.61 (d, J = 11.7 Hz, 1H), 7.30-7.37 (m, 5H). 13C NMR (CDCl3, 125 MHz) δ: 5.4 (q), 18.2 (s), 25.9 (q), 29.4 (t), 43.2 (d), 66.0 (t), 71.4 (t), 77.0 (d), 78.3 (d), 80.5 (d), 127.7 (d), 127.8 (d), 128.5 (d), 137.9 (s). Anal. Calcd for C19H32O4Si: C, 64.73; H, 9.15. Found: C, 64.66; H, 9.12.
(1R,2R,3R,5R)-3-(Benzyloxy)-5-((tert-butyldimethylsilyloxy)methyl)cyclopentane-1,2-diyl diacetate (24).
To a solution of 23 (400 mg, 1.13 mmol) in pyridine (8 mL), was added acetic anhydride (460 mg, 4.54 mmol) at 0 oC. The reaction mixture was stirred for 12 h, and concentrated. The residue obtained was purified by column chromatography (10% EtOAc in petroleum ether) to give 24 (340 mg, 73%) as colorless oil. [α]D –8.6 (c 1.7, MeOH). IR (CHCl3): 3019, 2929, 1737, 1216, 1108, 838, 758, 668 cm-1. 1H NMR (CDCl3, 500 MHz) δ: 0.02 (d, J = 1.3 Hz, 6H), 0.87 (s, 9H), 1.73-1.81 (m, 1H), 2.02-2.08 (m, 8H), 3.56-3.76 (m, 2H), 3.96-4.05 (m, 1H), 4.50-4.51 (m, 2H), 5.10 (qt, J = 4.4, 3.8 Hz, 2H), 7.26-7.34 (m, 5H).13C NMR (CDCl3, 125 MHz) δ: 5.53 (q), 5.44 (q), 18.2 (s), 20.9 (q), 21.02 (q), 25.9 (q), 30.8 (t), 42.9 (d), 64.4 (t), 71.9 (t), 78.0 (d), 127.5 (d), 128.4 (d), 138.2 (s), 170.1 (s), 170.4 (s). Anal. Calcd for C23H36O6Si: C, 63.27; H, 8.31. Found: C, 63.54; H, 8.26.
(1R,2R,3R,5R)-3-(Benzyloxy)-5-(hydroxymethyl)cyclopentane-1,2-diyl diacetate (5).
To a solution of 24 (300 mg) in EtOH (8 mL), 0.8% H2SO4 (catalytic amount) was added stirred at rt for 4 h. The reaction mixture was concentrated and the crude was purified by column chromatography (15% EtOAc in petroleum ether) to obtain 5 (150 mg, 63%) as colorless oil. [α]D –9.1 (c 1.5, MeOH). IR (CHCl3): 3392, 1737, 1373, 908, 733, 650 cm-1. 1H NMR (CDCl3, 500 MHz) δ: 1.62-1.74 (m, 1H), 2.09-2.10 (d, J = 1.5 Hz, 6H), 2.14-2.24 (m, 1H), 2.81 (br s, 1H), 3.58-3.71 (m, 2H), 4.04-4.14 (m, 1H), 4.52 (s, 2H), 5.08-5.13 (m, 2H), 7.29-7.38 (m, 5H). 13C NMR (CDCl3, 125 MHz) δ: 20.9 (q), 21.1 (q), 30.9 (t), 43.5 (d), 64.7 (t), 71.93 (t), 76.2 (d), 77.4 (d), 78.7 (d), 127.5 (d), 127.7 (d), 128.4 (d), 137.9 (s), 170.4 (s), 171.7 (s). Anal. Calcd for C17H22O6: C, 63.34; H, 6.88. Found: C, 63.23; H, 7.84.
(2R,3R,4S)-2-(Acetoxymethyl)-5-(pent-4-enyloxy)tetrahydrofuran-3,4-diyl diacetate (4).
A solution of per-O-acetyl-D-arabinofuranose (28) (1.0 g, 3.14 mmol), 4-penten-1-ol (400 mg, 4.71 mmol), and 4Å MS powder (100 mg) in CH2Cl2 (25 mL) under nitrogen was treated with BF3·Et2O (0.1 mL) at 0 oC. After 2 h, solid NaHCO3 was added and the mixture filtered through Celite. The filtrate was washed with brine, dried (Na2SO4) and concentrated. The residual syrup was purified by column chromatography (20% EtOAc in petroleum ether) to obtain 4 (870 mg, 80%) as colorless oil. [α]D +65.9 (c 1, CHCl3). IR (CHCl3): 3024, 2940, 1745, 1371, 1230, 1047, 913, 667 cm-1. 1H NMR (CDCl3, 500 MHz) δ: 1.63-1.78 (m, 3H), 2.11 (s, 10H), 3.46 (qt, J = 6.3, 12.5 Hz, 1H), 3.72 (dt, J = 6.7, 9.6 Hz, 1H), 4.17-4.28 (m, 2H), 4.39-4.48 (m, 1H), 4.94-5.08 (m, 5H), 5.72-5.92 (m, 1H). 13C NMR (CDCl3, 125 MHz) δ: 20.6 (q), 28.4 (t), 30.0 (t), 63.1 (t), 66.6 (t), 76.9 (d), 80.0 (d), 81.1 (d), 95.9 (s), 105.3 (d), 114.8 (t), 137.8 (d), 169.3 (s), 169.9 (s), 170.3 (s). Anal. Calcd for C16H24O8: C, 55.81; H, 7.02. Found: C, 56.79; H, 7.36.
2,3,2',3',5'-O-Pentaacetyl-1-O-benzyl-5-O-(4a-carba-β-D-arabinofuranozyl)-α-D-arabinofuranoside (29).
To a stirred suspension of 4 (160 mg, 0.62 mmol) and 5 (100 mg, 0.31 mmol) and 4Å MS powder in dry CH2Cl2 (5 mL), were added NIS (130 mg, 0.62 mmol) and cat. TfOH. The reaction mixture was stirred in the dark at rt for 24 h and filtered through a Celite bed. The filtrate was washed with saturated aqueous solution of NaHSO3 and aqueous NaHCO3 followed by brine solution. The organic layer was dried (Na2SO4), concentrated. The crude product was purified by column chromatography (20% EtOAc in petroleum ether) to obtain 29 (40 mg, 45%) as colorless oil. [α]D +30.9 (c 0.8, CHCl3). IR (CHCl3): 3020, 2927, 1742, 1372, 1216, 1083, 758, 669 cm-1. 1H NMR (CDCl3, 500 MHz) δ: 1.77-1.84 (m, 1H), 2.05 (s, 3H), 2.07 (s, 3H), 2.08 (s, 6H), 2.11 (s, 3H), 2.13-2.18 (m, 2H), 3.57 (dd, J = 5.0, 9.6 Hz, 1H), 3.73 (dd, J = 6.9, 9.6 Hz, 1H), 4.04 (qt, J = 5.5, 10.5 Hz, 1H), 4.16-4.24 (m, 3H), 4.38 (dd, J = 3.2 Hz, 11.5 Hz, 1H), 4.50 (s, 2H), 4.93 (dd, J = 1.4, 4.6 Hz, 1H), 5.01 (s, 1H), 5.04-5.12 (m, 2H), 5.17 (t, J = 5.9 Hz, 1H), 7.27-7.34 (m, 5H). 13C NMR (CDCl3, 125 MHz) δ: 20.6 (q), 20.7 (q), 20.8 (q), 20.9 (q), 30.9 (t), 40.0 (d), 63.2 (t), 68.2 (t), 71.9 (t), 76.4 (d), 77.11 (d), 77.14 (d), 77.3 (d), 80.4 (d), 81.0 (d), 105.3 (d), 127.5 (d), 127.7 (d), 128.4 (d), 128.6 (d), 138.1 (s), 169.5 (s), 169.9 (s), 170.1 (s), 170.2 (s), 170.4 (s). Anal. Calcd for C28H36O13: C, 57.93; H, 6.25. Found: C, 59.86; H, 6.22.
1,2,3,2',3',5'-O-Hexaacetyl-5-O-(4a-carba-β-D-arabinofuranozyl)-α-D-arabinofuranoside (3).
To solution of 29 (40 mg) in EtOAc (3 mL), was added Pd(OH)2/C (20%, 20 mg) The mixture was stirred for 5 h under hydrogen, filtered through a Celite bed and concentrated. The crude alcohol was directly taken for the next reaction. To a solution of crude alcohol (35 mg, 0.071 mmol) in pyridine (2 mL) was added acetic anhydride (140 mg, 0.14 mmol). The solution was stirred at rt for 6 h. Pyridine and acetic anhydride were removed under reduced pressure. Purification of the crude residue by column chromatography (30% EtOAc in petroleum ether) afforded 3 (25 mg, 78%) as colorless oil. [α]D +36.6 (c 1, CHCl3). IR (CHCl3): 3020, 2927, 2400, 1743, 1371, 1215, 1052, 758, 669, 529 cm-1. 1H NMR (CDCl3, 500 MHz) δ: 1.76-1.82 (m, 1H), 2.04 (s, 3H), 2.06 (s, 3H), 2.07 (s, 3H), 2.11 (d, J = 2.0 Hz, 9H), 2.22-2.39 (m, 2H), 3.55 (dd, J = 4.9, 9.5 Hz, 1H), 3.75 (dd, J = 6.7, 6.4 Hz, 1H), 4.18-4.29 (m, 2H), 4.39-4.49 (m, 1H), 4.96 (dd, J = 1.0, 4.2 Hz, 1H), 5.04 (s, 1H), 5.07 (d, J = 1.5 Hz, 1H), 5.12-5.18 (m, 1H), 5.21-5.30 (m, 2H). 13C NMR (CDCl3, 125 MHz) δ: 20.6 (q), 20.76 (q), 20.79 (q), 20.8 (q), 20.9 (q), 30.2 (t), 39.6 (d), 63.3 (t), 67.6 (t), 71.0 (d), 76.18 (d), 76.2 (d), 77.1 (d), 77.2 (d), 80.6 (d), 80.9 (d), 105.2 (d), 169.6 (s), 170.0 (s), 170.1 (s), 170.2 (s), 170.3 (s), 170.6 (s). Anal. Calcd for C23H32O14: C, 51.87; H, 6.06. Found: C, 51.84; H, 6.10.
ACKNOWLEDGEMENTS
C. N. R. thanks UGC (New Delhi) for the financial support in the form of a research fellowship.
This paper is dedicated to Professor Emeritus Keiichiro Fukumoto on the occasion of his 75th birthday.
References
1. N. D. Connell and H. M. Nikaido, In Tuberculosis: Pathogenesis, Protection and Control, ed. by B. R. Bloom, American Chemical Society for Microbiology, Washington, DC, 1994, p. 333.
2. (a) Xiaolei Tan, Chung K. Chu, and F. Douglas Boudinot, Adv. Drug Delivery Rev., 1999, 39, 117; CrossRef (b) T. S. Mansour and R. Storer, Curr. Pharm. Des., 1997, 3, 227; (c) V. E. Marquez, Adv. Antiviral Drug Des., 1996, 2, 89. CrossRef
3. (a) Y. Kajihara, H. Hashimoto, and S. Ogawa, Carbohydr. Res., 2000, 323, 44; CrossRef (b) S. Ogawa, N. Matsunaga, H. Li, and M. M. Palcic, Eur. J. Org. Chem., 1999, 631; CrossRef (c) S. Ogawa, T. Furuya, H. Tsunoda, O. Hindsgaul, K. Stangier, and M. M. Palcic, Carbohydr. Res., 1995, 271, 197. CrossRef
4. K. Tadano, H. Kimura, M. Hoshino, S. Ogawa and T. Suami, Bull. Chem. Soc. Jpn., 1987, 60, 3673. CrossRef
5. C. Kaneko, A. Sugimoto, and S. Tanaka, Synthesis, 1974, 876. CrossRef
6. U. R. Kalkote, S. R. Ghorpade, R. R. Joshi, T. Ravindranathan, K. B. Bastawade, and D. V. Gokhale, Tetrahedron: Asymmetry, 2000, 11, 2965. CrossRef
7. L. A. Agrofoglio, I. Gillaizeau, and Y. Saito, Chem. Rev., 2003, 103, 1875. CrossRef
8. B. M. Trost, R. Madsen, S. D Guile, and B. Brown, J. Am. Chem. Soc., 2000, 122, 5947. CrossRef
9. C. H. Behrens and K. Barry Sharpless, J. Org. Chem., 1985, 50, 5696. CrossRef
10. (a) D. R. Mootoo, Peter Konradsson, U. Udodong, and B. Fraser-Reid, J. Am. Chem. Soc., 1988, 110, 5583; CrossRef (b) P. Konradsson, D. R. Mootoo, R. E. McDevitt, and B. Fraser-Reid, J. Chem. Soc., Chem. Commun., 1990, 270; CrossRef (c) B. Fraser-Reid, U. E Udodong, Z. Wu, H. Ottosson, J. R. Merritt, C. S. Rao, C. Roberts, and R. Madsen, Synlett, 1992, 927 CrossRef