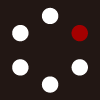
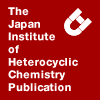
HETEROCYCLES
An International Journal for Reviews and Communications in Heterocyclic ChemistryWeb Edition ISSN: 1881-0942
Published online by The Japan Institute of Heterocyclic Chemistry
e-Journal
Full Text HTML
Received, 22nd July, 2008, Accepted, 5th September, 2008, Published online, 8th September, 2008.
DOI: 10.3987/COM-08-11501
■ Preparation of Unsymmetrical 1,4-Bis[2-ethynyl-3-thienyl]benzene Derivatives
Kozo Toyota,* Yasutomo Tsuji, Kazuyuki Okada, and Noboru Morita
Department of Chemistry, Graduate School of Science, Tohoku University, Aramaki, Aoba-ku, sendai 980-8578, Japan
Abstract
Unsymmetrically substituted 1,4-bis(2-ethynyl-3-thienyl)benzene derivative was prepared, utilizing 1-bromo-4-(2-iodo-3-thienyl)benzene and 5-alkyl-3-bromo-2-ethynylthiophene derivatives as key intermediates.INTRODUCTION
Molecular architectures have attracted current interest.2 Some of them are so called bio-mimetic or bio-inspired molecules, which resemble (from particular point of view concerning to the structure and functionality) to bio-polymers such as peptides, proteins, and so on. In the course of our continuing research on developing novel phosphorus ligands such as DPCBT (Chart 1),3–5 one of the author (K. T.) was inspired by structures of metalloproteins and peptides. We then designed 1,4-bis(2-ethynyl-3-thienyl)arene spacers (Chart 1, hereafter abbreviated to the ETAr spacer; in the case of m = 1, abbreviated to the ETB spacer)6,7 as promising and easily-tunable spacers and we prepared compounds 1–5, containing the ETB or ETAr spacer (Chart 2).
In order to progress the investigation of the ETB/ETAr spacer-linked system (as a peptide-inspired system; general structure is shown as A in Chart 2), establishment of fundamental synthetic technique is necessary. The key concept is a construction with the sequence of the side chains (or branches) under control, like peptide syntheses.
One of the promising synthetic intermediates for sequence-controlled oligomeric ETB molecules is unsymmetrical compound B (Chart 3, R1≠ R2), which has two differently substituted ethynyl groups as side chains. In addition, compound B has one substituted (or protected) site and one unprotected site, both α to the sulfur atoms. The unprotected site is useful for connection of the ETB unit to another substituent or ETB unit. On the other hand, the protected site will be used, after deprotection process, for further elongation of main chain of the oligomer. (It should be mentioned that previously reported preparative methods6,7 for ETB/ETAr derivatives are suitable for only symmetrical derivatives and not for unsymmetrical or sequence-controlled derivatives.)
The unsymmetrical compound B will be obtained by coupling of two units: thienylbenzene moiety (Chart 3, precursor I) and thiophene moiety (precursor II). These precursors will be obtained by utilization of difference in reactivities between bromo- and iodo-substituents. We report here preparation of unsymmetrically substituted ETB species of type B (R1≠ R2), which is an example of promising building blocks for construction of sophisticated and sequence-controlled oligomeric molecules.
RESULTS AND DISCUSSION
1-Bromo-4-(3-thienyl)benzene (6)8 was prepared in 73% yield by Suzuki-Miyaura coupling reaction of 1-bromo-4-iodobenzene with 3-thiopheneboronic acid (Scheme 1). Iodination of 6 with N-iodosuccinimide (NIS) gave 1-bromo-4-(2-iodo-3-thienyl)benzene (7) in 98% yield. The compound 7 is a key intermediate in the synthesis of 8 as well as various compounds of precursor I type (Chart 3), because ethynyl group can be introduced regioselectively to the thiophene ring of 7, utilizing different reactivity of iodo- and bromo-substituent. In fact, Sonogashira coupling reaction of 7 with 1-ethynyl-4-hexylbenzene afforded 1-bromo-4-(2-ethynyl-3-thienyl)benzene derivative 8. Compound 8 was then converted to a mono-substituted 1,4-di(3-thienyl)benzene species 9, by Suzuki-Miyaura coupling reaction with 3-thiopheneboronic acid, in 77% yield.
In order to check the reactivities of the 5-thienyl positions of 9, methylation reaction of 9 was carried out: Reaction of 9 (1 molar amount) with butyllithium (1.3 molar amount) and iodomethane (1.3 molar amount) gave a mixture of 10 (major product) and 11 (minor product) in a 1 : 0.09 ratio. In addition, trace of dimethylated derivative was detected by Mass spectroscopy.
Thus, regioselective connection of 1-(2-ethynyl-3-thienyl)-4-(3-thienyl)benzene derivatives (such as 9) may be possible and such derivatives may be used as building blocks for construction of sequence-controlled oligomeric molecules. However, in the cases of unsymmetrical ETB derivatives such as 1-[2-{2-(4-butylphenyl)ethynyl}-3-thienyl]-4-[2-{2-(4-hexylphenyl)ethynyl}-3-thienyl]benzene, introduction of the third substituent to the 5- or 5’-position of the thiophene rings seems to be much more difficult: In order to prepare sequence-controlled oligomeric ETB species, we need a compound of precursor II type, which was prepared as follows.
As an example of a preparation of precursor II, 3-bromo-2-[2-(4-butylphenyl)ethynyl]-5-methylthiophene (14) was synthesized from 4-bromo-2-methylthiophene via 3-bromo-2-iodo-5-methylthiophene (12)9 in 89% yield (based on 4-bromo-2-methylthiophene) by a route similar to that in the literature9 (Scheme 2, Route A), although NIS instead of I2/HIO3 was used for iodination and Sonogashira coupling was applied instead of Negishi coupling. This compound was converted to a dioxaborolane 15, by a similar manner reported in the literature.10
Compound 14 was also prepared by an alternative route as follows (Route B): 3-Bromo-2-[2-(4-butylphenyl)ethynyl]thiophene (13) was prepared by Sonogashira coupling reaction of 2,3-dibromothiophene with 1-butyl-4-ethynylbenzene. Lithiation of 13 with lithium diisopropylamide (LDA) followed by reaction with iodomethane afforded 14 in 30% yield. In spite of the low yield due to tedious separation process, Route B is more convenient than Route A, because 2,3-dibromothiophene is a market-available reagent. We can introduce various alkyl (to 5-thienyl position) and ethynyl (to 2-thienyl position) groups easily and regioselectively: this is an advantage of both Routes A and B.
As we obtained both precursors I and II, we tried to prepare unsymmetrical 1,4-bis(2-ethynyl-3-thienyl)benzene derivative, containing two different arylethynyl groups (Scheme 3): Suzuki-Miyaura coupling reaction of 8 with 15 afforded unsymmetrical compound 16 (in 49% yield), which belongs to a category of compound B in Chart 3.
In summary, we have prepared unsymmetrical 1,4-bis(2-ethynyl-3-thienyl)benzene derivative, utilizing 7 and 14 as key intermediates. Principally, the present method is applicable to other ETAr spacers such as 4,4’-(2-ethynyl-3-thienyl)biphenyl spacer.7 Various combination of donor-accepter system will be introduced to the ETB spacer by this method. Furthermore, this rather simple preparative method of unsymmetrical ETB species will help construction of sophisticated molecules such as metalloprotein mimetics or artificial enzymes, by introducing side chains of various functionalities such as metal-coordinating ability and molecule-recognizing ability.
EXPERIMENTAL
Melting points were measured on a Yanagimoto MP-J3 micro melting points apparatus and were uncorrected. NMR spectra were recorded on a Bruker Avance-400 or a JEOL JNM-GSX400. UV-vis spectra were measured on a Hitachi U-3210 spectrometer. IR spectra were obtained on a Horiba FT-300 spectrometer or a Shimadzu FTIR-8100M spectrometer. MS spectra were taken on a Hitachi M-2500S spectrometer. FT-ICR-MS spectra were measured on a Bruker APEX III spectrometer.
1-Bromo-4-(3-thienyl)benzene (6). A mixture of 1-bromo-4-iodobenzene (4.43 g, 15.7 mmol), 3-thiopheneboronic acid (2.00 g, 15.6 mmol), tetrakis(triphenylphosphine)palladium (632.2 mg, 0.547 mmol), K2CO3 (10.81 g, 78.2 mmol), toluene (40 mL), THF (40 mL), and water (20 mL) was heated at 85 °C for 20 h. After cooling to rt, CHCl3 and water were added to the reaction mixture and the organic phase was dried over MgSO4. The solvent was removed under reduced pressure. The residue was treated with a silica-gel column chromatography (CH2Cl2) to give 2.72 g (11.37 mmol, 73% yield) of 6: Colorless powder, mp 123.5–124.5 °C (lit.,8a 124–125 °C ); Rf = 0.53 (SiO2-hexane); 1H NMR (400 MHz, CDCl3) δ = 7.35 (1H, dd, J = 5.0 Hz and 1.3 Hz, 4-thienyl), 7.40 (1H, dd, J = 5.0 Hz and 3.0 Hz, 5-thienyl), 7.45 (1H, dd, J = 3.0 Hz and 1.3 Hz, 2-thienyl), 7.46 (2H, AA’BB’, 3- and 5-phenyl), and 7.52 (2H, AA’BB’, 2- and 6-phenyl); 13C{1H} NMR (100 MHz, CDCl3) δ = 120.6 (2-thienyl), 121.0 (1-phenyl), 126.0 (4-thienyl), 126.5 (5-thienyl), 127.9 (3- and 5-phenyl), 131.8 (2- and 6-phenyl), 134.7 (4-phenyl), and 141.1 (3-thienyl).
1-Bromo-4-(2-iodo-3-thienyl)benzene (7). A mixture of 1-bromo-4-(3-thienyl)benzene 6 (1.65 g, 6.90 mmol), N-iodosuccinimide (1.87 g, 8.30 mmol), 2,2’-azobis(2-methylpropionitrile) (AIBN, 127.5 mg, 0.78 mmol), and acetic acid (20 mL) in CHCl3 (28 mL) was stirred at 50 °C for 4 h. CHCl3 and water were added to the reaction mixture and the organic phase was treated with saturated aqueous NaHCO3 and then saturated aqueous Na2S2O3 solution. The organic phase was washed with brine, dried over MgSO4, and the solvent was removed under reduced pressure. The residue was treated with a silica-gel column chromatography (CCl4) to give 2.46 g (6.74 mmol, 98% yield) of 7: Colorless solid, mp 42–44 °C; Rf = 0.39 (SiO2-hexane); 1H NMR (400 MHz, CDCl3) δ = 6.49 (1H, d, J = 5.5 Hz, 4-thienyl), 7.38 (2H, d, J = 8.4 Hz, 3- and 5-phenyl), 7.50 (1H, d, J = 5.5 Hz, 5-thienyl), and 7.57 (2H, d, J = 8.4 Hz, 2- and 6-phenyl); 13C{1H} NMR (100 MHz, CDCl3) δ = 73.4 (2-thienyl), 121.8 (1-phenyl), 128.7 (4-thienyl), 130.4 (3- and 5-phenyl), 131.4 (5-thienyl, 2- and 6-phenyl), 135.3 (4-phenyl), and 145.4 (3-thienyl); IR (KBr) 1591, 1525, 1483, 1466, 1402, 1394, 1338, 1271, 1246, 1182, 1107, 1076, 1064, 1047, 1008, 960, 879, 870, 827, 814, 777, 723, 706, 648, 634, 615, 468, 424, and 412 cm–1; EI-MS (70 eV) m/z (rel intensity) 364 (M+; 98) and 366 (M++2; 100). Calcd for C10H6BrIS: M, 363.8418. Found: m/z 363.8415.
1-Bromo-4-[2-{2-(4-hexylphenyl)ethynyl}-3-thienyl]benzene (8). A mixture of 7 (1.79 g, 4.90 mmol), 1-ethynyl-4-hexylbenzene (1.01 g, 5.41 mmol), dichlorobis(triphenylphosphine)palladium(II) (518.8 mg, 0.74 mmol), copper(I) iodide (102.5 mg, 0.54 mmol), and diisopropylamine (60 mL) in THF (70 mL) was stirred at 50 °C for 3 d. After cooling to rt, CHCl3 and water were added to the reaction mixture. The organic phase was washed with brine and dried over MgSO4. The solvent was removed under reduced pressure and the residue was treated with a silica-gel column chromatography (CCl4) and then with a gel permeation column chromatography (Bio-Rad Labo., Inc., Bio-Beads S-X3, 200-400 Mesh, CH2Cl2 as eluent) to give 584.2 mg (1.38 mmol, 28% yield) of 8: Colorless solid, mp 56–58 °C; Rf = 0.21 (SiO2-hexane); 1H NMR (400 MHz, CDCl3) δ = 0.88 (3H, t, J = 6.9 Hz, Me), 1.30 (6H, m, CH2), 1.56–1.60 (2H, m, CH2), 2.61 (2H, t, J = 7.7 Hz, CH2), 7.16 (2H, d, J = 8.2 Hz, phenyl), 7.18 (1H, d, J = 5.3 Hz, 4-thienyl), 7.29 (1H, d, J = 5.3 Hz, 5-thienyl), 7.37 (2H, d, J = 8.2 Hz, phenyl), 7.56 (2H, d, J = 8.7 Hz, phenyl), and 7.71 (2H, d, J = 8.7 Hz, phenyl); 13C{1H} NMR (100 MHz, CDCl3) δ = 14.0 (Me), 22.5 (CH2), 28.8 (CH2), 31.1 (CH2), 31.6 (CH2), 35.9 (CH2), 82.2 (C≡C), 95.9 (C≡C), 118.8, 119.9, 121.5, 126.4, 127.5, 128.5 (phenyl), 129.5 (phenyl), 131.1 (phenyl), 131.5 (phenyl), 134.2, 143.0, and 143.8; IR (KBr) 1527, 1506, 1068, 1008, 873, 823, 729, 706, 648, and 538 cm–1; EI-MS (70 eV) m/z (rel intensity) 422 (M+; 94) and 424 (M++2; 100). Calcd for C24H23BrS: M, 422.0704. Found: m/z 422.0700.
1-[2-{2-(4-Hexylphenyl)ethynyl}-3-thienyl]-4-(3-thienyl)benzene (9). Reaction was carried out under literature conditions.10 A mixture of 8 (174.9 mg, 0.413 mmol), 3-thiopheneboronic acid (53.5 mg, 0.418 mmol), tetrakis(triphenylphosphine)palladium (9.6 mg, 0.008 mmol), triphenylphosphine (20.6 mg, 0.0785 mmol), K3PO4 (441.7 mg, 2.08 mmol), 1,4-dioxane (20 mL), and water (8 mL) was heated at 90 °C for 16 h. After cooling to rt, CHCl3 and water were added to the reaction mixture. The organic phase was washed with brine and dried over MgSO4. The solvent was removed under reduced pressure and the residue was treated with a silica-gel column chromatography (CCl4) and the crude product was treated with a silica-gel column chromatography (hexane) again to give 136.0 mg (0.319 mmol, 77% yield) of 9: Colorless solid, mp 93–94 °C; Rf = 0.12 (SiO2-hexane); 1H NMR (400 MHz, CDCl3) δ = 0.88 (3H, t, J = 6.8 Hz, Me), 1.30 (6H, m, CH2), 1.60 (2H, quin, J = 7.2 Hz, CH2), 2.61 (2H, t, J = 7.7 Hz, CH2), 7.15 (2H, d, J = 8.2 Hz, phenyl), 7.26 (1H, d, J = 5.3 Hz, 4-thienyl), 7.30 (1H, d, J = 5.3 Hz, 5-thienyl), 7.38 (2H, d, J = 8.2 Hz, phenyl), 7.42 (1H, dd, J = 5.0 Hz and 2.9 Hz, 5’-thienyl), 7.46 (1H, dd, J = 5.0 Hz and 1.4 Hz, 4’-thienyl), 7.52 (1H, dd, J = 2.9 Hz and 1.4 Hz, 2’-thienyl), 7.68 (2H, AA’BB’, phenyl), and 7.90 (2H, AA’BB’, phenyl); 13C{1H} NMR (100 MHz, CDCl3) δ = 14.0 (Me), 22.5 (CH2), 28.9 (CH2), 31.2 (CH2), 31.6 (CH2), 35.9 (CH2), 82.7 (C≡C), 95.6 (C≡C), 118.3, 120.1, 120.3, 126.2, 126.2, 126.3 (phenyl), 127.7, 128.3 (phenyl), 128.5 (phenyl), 131.2 (phenyl), 134.1, 134.9, 141.9, 143.6, and 143.8; UV (CH2Cl2) 236 (log ε 4.42), 283 (4.55), and 330 nm (4.40); IR (KBr) 1541, 1522, 1506, 1466, 1435, 1298, 1203, 1115, 1078, 1020, 866, 844, 819, 785, 738, 711, 646, 632, 569, 540, 522, and 505 cm–1; Found: m/z 449.1367. Calcd for C28H26NaS2: M++Na, 449.1374. Anal. Calcd for C28H26S2: C, 78.83; H, 6.14%. Found: C, 78.60; H, 6.04%.
Methylation reaction of 9. To a solution of 9 (40.5 mg, 0.095 mmol) in THF (10 mL) was added 0.12 mmol of butyllithium (1.55 M solution in hexane, 0.08 mL) at –78 °C, the reaction mixture was stirred for 30 min at that temperature, and 0.12 mmol (7.5 µL) of iodomethane was added. The resulting solution was stirred at –78 °C for 1.5 h and allowed to warm to rt. To the reaction mixture were added CHCl3 (ca. 30 mL) and water (ca. 30 mL), the organic phase was dried over MgSO4, and the solvent was removed under reduced pressure. The residue was treated with a silica-gel column chromatography (CCl4) to give 38.0 mg of a mixture of 10 and 11 (1 : 0.09 ratio, determined by 1H NMR spectroscopy). 10: Rf = 0.11 (SiO2-CCl4); 1H NMR (400 MHz, CDCl3) δ = 0.87 (3H, t, J = 6.6 Hz, Me), 1.29 (6H, m, CH2), 1.59 (2H, m, CH2), 2.49 (3H, s, thienyl-CH3), 2.59 (2H, t, J = 7.7 Hz, CH2), 6.91 (1H, s, 4-thienyl), 7.13 (2H, d, J = 8.0 Hz, phenyl), 7.36–7.40 (1H, m, thienyl), 7.37 (2H, d, J = 8.0 Hz, phenyl), 7.43 (1H, dd, J = 5.0 Hz and 0.9 Hz, thienyl), 7.48 (1H, dd, J = 2.8 Hz and 0.9 Hz, thienyl), 7.64 (2H, d, J = 8.1 Hz, phenyl), and 7.86 (2H, d, J = 8.1 Hz, phenyl); 13C{1H} NMR (100 MHz, CDCl3) δ = 14.1 (Me), 15.5 (thienyl-Me), 22.6 (CH2), 28.9 (CH2), 31.2 (CH2), 31.7 (CH2), 35.9 (CH2), 83.1 (C≡C), 94.9 (C≡C), 115.8 (2-thienyl), 120.3, 120.4, 126.1, 126.2, 126.3, 128.2, 128.4, 131.1, 134.4, 134.7, 140.7 (5-thienyl), 142.0, 143.4, and 143.9.
3-Bromo-2-[2-(4-butylphenyl)ethynyl]thiophene (13). Reaction was carried out under literature conditions.11 A mixture of 2,3-dibromothiophene (1.00 mL, 8.83 mmol), 1-butyl-4-ethynylbenzene (1.70 mL, 9.73 mmol), dichlorobis(triphenylphosphine)palladium(II) (24.5 mg, 0.035 mmol), copper(I) iodide (12.6 mg, 0.066 mmol), and triphenylphosphine (11.4 mg, 0.043 mmol) in triethylamine (20 mL) was stirred at 60 °C for 8 h. After cooling to rt, EtOAc (100 mL) and water (100 mL) were added to the reaction mixture and the organic phase was dried over MgSO4. The solvent was removed under reduced pressure and the residue was treated with a silica-gel column chromatography (hexane) to give 1.9404 g (6.08 mmol, 69% yield) of crude 13. This product was further purified by gel permeation liquid chromatography (Japan Analytical Industry, JAIGEL H1+H2 column) to give 1.4718 g (4.61 mmol, 52% yield) of 13: Colorless oil; Rf = 0.41 (SiO2-hexane); 1H NMR (400 MHz, CDCl3) δ = 0.92 (3H, t, J = 7.3 Hz, Me), 1.29–1.39 (2H, tq, CH2), 1.55-1.62 (2H, tt, CH2), 2.61 (2H, t, J = 7.7 Hz), 6.97 (1H, d, J = 5.4 Hz, 4-thienyl), 7.15 (2H, d, J = 8.2 Hz, 3- and 5-phenyl), 7.18 (1H, d, J = 5.4 Hz, 5-thienyl), and 7.45 (2H, d, J = 8.2 Hz, 2- and 6-phenyl); 13C{1H} NMR (100 MHz, CDCl3) δ = 13.9 (Me), 22.3 (CH2), 33.3 (CH2), 35.6 (CH2), 80.4 (C≡C), 97.3 (C≡C), 115.8 (3-thienyl), 119.6 (2-thienyl or 1-phenyl), 121.1 (2-thienyl or 1-phenyl), 126.8 (5-thienyl), 128.5 (3- and 5-phenyl), 130.0 (4-thienyl), 131.5 (2- and 6-phenyl), and 144.0 (4-phenyl); IR (neat) 2209 (C≡C), 1607, 1522, 1495, 1428, 1412, 1377, 1348, 1183, 1156, 1075, 1019, 864, 839, 826, 760, 708, 606, 567, and 534 cm–1; MS (70 eV) m/z (rel intensity) 320 (M++2; 98), 318 (M+; 95), 277 (M+–Pr+2; 100), and 275 (M+–Pr; 95). Calcd for C16H15BrS: M+, 318.0072. Found: m/z 318.0076.
3-Bromo-2-[2-(4-butylphenyl)ethynyl]-5-methylthiophene (14). Route A. A mixture of 4-bromo-2-methylthiophene (1.00 g, 5.65 mmol), N-iodosuccinimide (1.27 g, 5.64 mmol), AIBN (95.2 mg, 0.580 mmol), and acetic acid (15 mL) in CHCl3 (20 mL) was stirred at 50 °C for 3 h. To the reaction mixture were added CHCl3 (ca. 30 mL) and water (ca. 40 mL) and the resulting mixture was treated with saturated aqueous NaHCO3 and then saturated aqueous Na2S2O3 solution. The organic phase was washed with brine, dried over MgSO4, and the solvent was removed under reduced pressure. The residue was treated with a silica-gel column chromatography (hexane) to give 129 (1.62 g, 5.35 mmol, 95% yield). This product was used in the following reaction. A mixture of 12 (809.6 mg, 2.67 mmol), 1-butyl-4-ethynylbenzene (412.6 mg, 2.61 mmol), dichlorobis(triphenylphosphine)palladium(II) (28.8 mg, 0.041 mmol), copper(I) iodide (7.0 mg, 0.037 mmol), and diisopropylamine (15 mL) in THF (20 mL) was stirred at rt for 5 h. CHCl3 (ca. 100 mL) and water (ca. 100 mL) were added to the reaction mixture, separated, and the organic phase was dried over MgSO4. The solvent was removed under reduced pressure and the residue was treated with a silica-gel column chromatography (hexane) to give 14 (831.8 mg, 2.50 mmol, 94% yield based on 12).
Route B. To a solution of 13 (200.1 mg, 0.627 mmol) in THF (15 mL) was added 0.90 mmol of lithium diisopropylamide (1.8 M solution in heptane/THF/ethylbenzene, 0.5 mL) at 0 °C, the reaction mixture was stirred for 20 min at that temperature, and 0.68 mmol (0.4 mL) of iodomethane was added. The resulting solution was stirred at 0 °C for 45 min. To the reaction mixture were added CHCl3 (ca. 50 mL) and water (ca. 50 mL), the organic phase was dried over MgSO4, and the solvent was removed under reduced pressure. The residue was treated with a silica-gel column chromatography (CCl4) and gel permeation liquid chromatography (Japan Analytical Industry, JAIGEL H1+H2 column) to give 61.9 mg (0.186 mmol, 30% yield) of 14.
14: Pale yellow oil; Rf = 0.28 (SiO2-hexane); 1H NMR (400 MHz, CDCl3) δ = 0.92 (3H, t, J = 7.3 Hz, CH2Me), 1.29–1.39 (2H, m, CH2), 1.54–1.62 (2H, m, CH2), 2.44 (3H, s, thienyl-Me), 2.60 (2H, t, J = 7.7 Hz, CH2), 6.64 (1H, s, 4-thienyl), 7.14 (2H, d, J = 8.0 Hz, phenyl), and 7.44 (2H, d, J = 8.0 Hz, phenyl); 13C{1H} NMR (100 MHz, CDCl3) δ = 13.9 (Me), 15.6 (thienyl-Me), 22.2 (CH2), 33.3 (CH2), 35.6 (CH2), 80.6 (C≡C), 96.4 (C≡C), 115.0 (3-thienyl), 118.4 (2-thienyl or 1-phenyl), 119.8 (2-thienyl or 1-phenyl), 128.0 (4-thienyl), 128.4 (3- and 5-phenyl), 131.3 (2- and 6-phenyl), 141.4 (4-phenyl or 5-thienyl), and 143.7 (4-phenyl or 5-thienyl); IR (neat) 2205 (C≡C), 1908, 1607, 1534, 1510, 1466, 1445, 1412, 1379, 1327, 1281, 1177, 1115, 1088, 1019, 930, 831, 824, 689, 606, 550, and 507 cm–1; MS (70 eV) m/z (rel intensity) 334 (M++2; 100), 332 (M+; 98), 291 (M+–Pr+2; 86), and 289 (M+–Pr;85). Calcd for C17H17BrS: M+, 332.0229. Found: m/z 332.0235.
2-[2-{2-(4-Butylphenyl)ethynyl}-5-methyl-3-thienyl]-4,4,5,5-tetramethyl-1,3,2-dioxaborolane (15). To a solution of 14 (419.3 mg, 1.26 mmol) in THF (15 mL) was added 1.32 mmol of butyllithium (1.55 M solution in hexane, 0.85 mL) at –78 °C and the resulting mixture was stirred at that temperature for 30 mim. To the solution was added 2-isopropoxy-4,4,5,5-tetramethyl-1,3,2-dioxaborolane (0.29 mL, 1.42 mmol) and the reaction mixture was stirred at –78 °C for 1 h. To the resulting mixture were added water (ca. 50 mL) and EtOAc (ca. 50 mL), separated, the organic phase was dried over MgSO4. The solvent was removed under reduced pressure and the residue was treated with a silica-gel column chromatography (hexane) to give 15 (419.3 mg, 1.10 mmol, 92% yield). This product was used in the following reaction without further purification. 15: 1H NMR (400 MHz, CDCl3) δ = 0.92 (3H, t, J = 7.3 Hz, Me), 1.26–1.33 (2H, m, CH2), 1.35 (12H, s, CMe2), 1.56–1.62 (2H, m, CH2), 2.45 (3H, s, thienyl-Me), 2.61 (2H, t, J = 7.7 Hz, CH2), 6.93 (1H, s, 4-thienyl), 7.15 (2H, d, J = 8.2 Hz, phenyl), and 7.43 (2H, d, J = 8.2 Hz, phenyl); 13C{1H} NMR (100 MHz, CDCl3) δ = 13.8 (Me), 14.8 (thienyl-Me), 22.2 (CH2), 24.8 (CMe2), 33.3 (CH2), 35.5 (CH2), 83.4 (CMe2), 83.6 (C≡C), 94.8 (C≡C), 120.8, 128.3 (phenyl), 128.5, 130.9, 131.0 (phenyl), 132.3, 140.5, and 143.0.
1-[2-{2-(4-Butylphenyl)ethynyl}-5-methyl-3-thienyl]-4-[2-{2-(4-hexylphenyl)ethynyl}-3-thienyl]-
benzene (16). A mixture of 8 (140.1 mg, 0.331 mmol), 15 (166.2 mg, 0.437 mmol), tetrakis(triphenylphosphine)palladium (16.1 mg, 0.0139 mmol), triphenylphosphine (22.0 mg, 0.0839 mmol), K3PO4 (455.0 mg, 2.14 mmol), 1,4-dioxane (20 mL), and water (8 mL) was heated at 90 °C for 4 h. After cooling to rt, CHCl3 (ca. 50 mL) and water (ca. 50 mL) were added to the reaction mixture. The organic phase was washed with brine and dried over MgSO4. The solvent was removed under reduced pressure and the residue was treated with a silica-gel column chromatography (hexane-CHCl3) to give 97.5 mg (0.163 mmol, 49% yield) of 16: Colorless solid, mp 91–93 °C; Rf = 0.11 (SiO2-hexane); 1H NMR (400 MHz, CDCl3) δ = 0.88 (3H, t, J = 7.3 Hz, Me), 0.92 (3H, t, J = 7.3 Hz, Me), 1.29–1.36 (8H, m, CH2), 1.52–1.61 (4H, m, CH2), 2.52 (3H, s, thienyl-Me), 2.59 (4H, t, J = 7.6 Hz, CH2), 6.95 (1H, s, 4-thienyl), 7.09 (2H, d, J = 8.1 Hz, phenyl), 7.10 (2H, d, J = 8.1 Hz, phenyl), 7.27 (1H, d, J = 5.4 Hz, thienyl), 7.30 (1H, d, J = 5.4 Hz, thienyl), 7.37 (2H, d, J = 8.1 Hz, phenyl), 7.39 (2H, d, J = 8.1 Hz, phenyl), and 7.92 (4H, pseudo s, phenyl); 13C{1H} NMR (100 MHz, CDCl3) δ = 13.9 (Me), 14.0 (Me), 15.5 (thienyl-Me), 22.2 (CH2), 22.5 (CH2), 28.9 (CH2), 31.1 (CH2), 31.6 (CH2), 33.3 (CH2), 35.5 (CH2), 35.9 (CH2), 82.7 (C≡C), 83.0 (C≡C), 94.9 (C≡C), 95.7 (C≡C), 115.9, 118.3, 120.1, 120.3, 126.1, 126.2, 127.7 (phenyl), 127.8 (phenyl), 128.4 (phenyl), 128.4 (phenyl), 131.1 (phenyl), 131.1 (phenyl), 134.3, 134.7, 140.7, 143.2, 143.5, and 143.8; UV (CH2Cl2) 286 (log ε 4.62), 323 (4.56), and 346 nm (sh, 4.49); IR (KBr) 2195 (C≡C), 1607, 1534, 1512, 1501, 1460, 1412, 1377, 1293, 1273, 1183, 1084, 1019, 876, 858, 828, 747, 714, 575, 536, and 511 cm–1; Found: m/z 619.2463. Calcd for C41H40NaS2: M++Na, 619.2464. Anal. Calcd for C41H40S2(H2O)1/2: C, 81.28; H, 6.82%. Found: C, 81.88; H, 7.02%.
ACKNOWLEDGEMENTS
This work was supported in part by the Grants-in-Aid for Scientific Research (No. 20550030) from the Ministry of Education, Culture, Sports, Science and Technology.
References
1. Chemistry of bis(2-ethynyl-3-thienyl)arene system, Part 3. For Part 2, see Ref. 7.
2. A. C. Reed, Acc. Chem. Res., 2005, 38, 215 and papers in this issue of the journal. For recent reports on oligophenylene rods, see for example:; CrossRef A. L. Sisson, N. Sakai, N. Banerji, A. Fürstenberg, E. Vauthey, and S. Matile, Angew. Chem. Int. Ed., 2008, 47, 3727; CrossRef K. Tsubaki, K. Takaishi, D. Sue, K. Matsuda, Y. Kanemitsu, and T. Kawabata, J. Org. Chem., 2008, 73, 4279; CrossRef J.-H. Ryu, D.-J. Hong, and M. Lee, Chem. Commun., 2008, 1043 and references cited therein. CrossRef
3. K. Toyota, K. Abe, K. Horikawa, and M. Yoshifuji, Bull. Chem. Soc. Jpn., 2004, 77, 1377; CrossRef K. Toyota, K. Horikawa, R. S. Jensen, K. Omori, S. Kawasaki, S. Ito, M. Yoshifuji, and N. Morita, Bull. Chem. Soc. Jpn., 2007, 80, 1580. CrossRef
4. For DPCBT polymers, see: a) K. Toyota, J. Ujita, S. Kawasaki, K. Abe, N. Yamada, and M. Yoshifuji, Tetrahedron Lett., 2004, 45, 7609; CrossRef b) S. Kawasaki, J. Ujita, K. Toyota, and M. Yoshifuji, Chem. Lett., 2005, 34, 724. CrossRef
5. For application of DPCBT and related ligands, see: A. Nakamura, S. Kawasaki, K. Toyota, and M. Yoshifuji, J. Organomet. Chem., 2007, 692, 70; CrossRef K. Toyota, K. Masaki, T. Abe, and M. Yoshifuji, Chem. Lett., 1995, 221; CrossRef A. S. Gajare, R. S. Jensen, K. Toyota, M. Yoshifuji, and F. Ozawa, Synlett, 2005, 144.
6. K. Toyota, Y. Goto, K. Okada, and N. Morita, Heterocycles, 2007, 71, 2227. CrossRef
7. K. Toyota, K. Okada, H. Katsuta, and N. Morita, Tetrahedron, submitted.
8. a) G. Martelli, P. Spagnolo, and M. Tiecco, J. Chem. Soc. (B), 1968, 901; CrossRef b) S. Hauptmann and E.-M. Werner, J. Prakt. Chem., 1972, 314, 499; CrossRef c) D. J. Guerrero, D. X. Ren, J. P. Ferraris, Chem. Mater., 1994, 6, 1437. CrossRef
9. J. O. Karlsson, A. Svensson, and S. Gronowitz, J. Org. Chem., 1984, 49, 2018. CrossRef
10. S. Kawai, T. Nakashima, K. Atsumi, T. Sakai, M. Harigai, Y. Imamoto, H. Kamikubo, M. Kataoka, and T. Kawai, Chem. Mater., 2007, 19, 3479. CrossRef
11. R. Pereira, B. Iglesias, and A. R. de Lera, Tetrahedron, 2001, 57, 7871 CrossRef