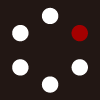
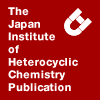
HETEROCYCLES
An International Journal for Reviews and Communications in Heterocyclic ChemistryWeb Edition ISSN: 1881-0942
Published online by The Japan Institute of Heterocyclic Chemistry
e-Journal
Full Text HTML
Received, 25th July, 2008, Accepted, 18th September, 2008, Published online, 22nd September, 2008.
DOI: 10.3987/COM-08-11504
■ Reaction of Isatins with Active Methylene Compounds on Neutral Alumina: Formation of Knoevenagel Condensates and Other Interesting Products
Manas Chakrabarty,* Ratna Mukherjee, Shiho Arima, and Yoshihiro Harigaya
Department of Chemistry, Bose Institute, 93/1, A. P. C. Road, Kolkata-700009, India
Abstract
Isatin and its N-methyl and 5-nitro derivatives underwent smooth reaction with a variety of acyclic and cyclic active methylene compounds on neutral alumina at room temperature to efficiently furnish Knoevenagel condensates in the majority of the cases and tandem Knoevenagel condensation-Michael addition products including spiro compounds in two cases.INTRODUCTION
The Knoevenagel condensation is one of the most useful and widely employed methods for carbon-carbon bond formation in organic synthesis, resulting in functionalised ylidenes.1 Homogeneous base catalysts such as ammonia or ammonium salts, primary and secondary amines, pyridine and piperidine have been mostly used for this reaction.2 The condensation has also been accomplished (i) using heterogeneous catalysts including Lewis acids,3 inorganic salts,4 resins5 and ionic liquids,6 (ii) in water, both catalysed and uncatalysed7 as well as (iii) via electrochemical, microwave-irradiated and ultrasound-mediated methods.8 Knoevenagel condensates are useful as ene-components in Diels-Alder reactions, as π-electron acceptors in the study of charge-transfer complexes and as synthons for complex molecules, many of which possess bioactivities.9 Moreover, the condensates find increasing applications in medicine, agriculture, dye industries and biological science.10
In continuation of our programmes on the synthesis of condensed heterocycles on solid catalysts,11-15 we became interested in isatin16 for use in Knoevenagel condensation primarily since the Knoevenagel condensates of isatin with malononitrile, cyanoacetamide and ethyl cyanoacetate have recently been reported to be selective inhibitors of cyclin dependent kinases (CDKs) of Plasmodium falciparum.17
A perusal of the methods reported2, 18-29 for the successful Knoevenagel condensation of isatin led to three revealing observations. Firstly, N-methylisatin and N-(2,6-dichlorophenyl)isatin had earlier been reported to efficiently react with malononitrile in methanol and ethanol, respectively at rt without any catalyst to furnish the corresponding Knoevenagel condensates in 95% and 93% respective yields.18, 19 Therefore, we became curious to check if isatin behaves similarly. Hence, isatin (1a) was treated with malononitrile (2a) in methanol at rt without using any catalyst. The reaction, complete in five minutes, furnished the Knoevenagel condensate in 99% yield.
In contrast to these uncatalysed Knoevenagel condensations, the second important observation was that, in most cases, the Knoevenagel condensates in general had earlier been prepared in refluxing alcohols using strong bases or acids as homogeneous catalysts.2, 20-28 We realised that malononitrile is too strong an active methylene compound to need any catalyst for its reaction with isatin and substituted isatins. But for other active methylene compounds, the use of a catalyst was necessary. Since none of the syntheses cited above was carried out on solid surfaces, which was our prime interest, our search for a suitable solid catalyst for this purpose still remained on.
At this stage, we stumbled upon the third significant observation,29 in which isatin was made to undergo successful Knoevenagel condensation with only malononitrile on neutral alumina by heating at 85 °C for 60 min and separately under microwave irradiation (MWI) (40% power) for 5 min to furnish the expected Knoevenagel condensate in 50% and 78% yields, respectively. Clearly, the application of heating or microwave irradiation was unnecessary in this case. But, taking the clue from this report, we planned to check if neutral alumina alone (i.e. without the application of heat or microwave irradiation) is effective for the Knoevenagel condensation of isatin and substituted isatins with active methylene compounds in general.
Accordingly, isatin and two substituted isatins were separately treated with a host of acyclic and cyclic active methylene compounds on neutral alumina at rt in a so called solvent-free manner.30 The outcome of our experiments was quite interesting and is, therefore, presented herein.
RESULTS AND DISCUSSION
As planned, isatin (1a) and an equimolar amount (unless necessitated otherwise) of each of ethyl cyanoacetate (2b), cyanoacetamide (2c), diethyl malonate (2d), hydantoin (2e), methyl acetoacetate (2f), barbituric acid (2g), dimedone (2h), thiazolidine-2,4-dione (2i) and indane-1,3-dione (2j), uniformly adsorbed on commercially procured neutral alumina, was kept at rt. Subsequently, N-methylisatin (1b) was treated with 2b and 5-nitroisatin (1c) separately with 2a and 2b in a similar manner. Except for 2i and 2j, which failed to react at rt, at elevated temperature (60-70 °C) and even under MWI (20% power, 10 min), the reactions were complete in 5-35 min (except for 2f, which required nearly an hour). We believe, both 2i and 2j are very weak active methylene compounds, and that is why they did not react with 1a. In support of our belief, we like to state that both 2i and 2j had earlier been reported to undergo condensations with ketones only under strong conditions, 2i furnishing the Knoevenagel condensates under basic conditions2 but 2j giving rise to aldol products under acidic or neutral conditions.31 The products from the reactions of 1a with 2b-e, of 1b with 2b and of 1c with 2a and 2b were identified as the corresponding Knoevenagel condensates (3ab-ae, bb, ca, cb), isolated in 78-100% yields (Scheme 1, Table 1). The two new products, 3ca and 3cb, were characterised by detailed spectroscopic and elemental analyses (vide experimental). The rest of the products, since known, were identified by comparing their melting points with those reported in the literature and supportive 1H NMR spectroscopic data.
The product from 1a and 2f recorded a molecular formula of C13H11NO4 (HR FAB-MS), which corresponded to the molecular formula of the expected Knoevenagel condensate (3af). Junek et al.19 had earlier ascertained the ratio of the E- and the Z-isomers of the Knoevenagel condensates of isatin and its N-methyl and N-(2,6-dichlorophenyl) derivatives (with malononitrile and ethyl cyanoacetate) by a combined 13C NMR analysis and by measuring the NOEs of H-4 as a result of the double irradiation of the CH2 group of the ethyl ester moiety. It was demonstrated therein that the carbon signals for C-5 and, more importantly, for C-7 appear at δ 135-138 and δ 110-111, respectively for both the E- and the Z-isomers. The absence of any CH signal in these ranges in the 13C NMR spectrum of the product ruled out its oxindole structure. The only alternative was the 2-hydroxyindolenine tautomeric structure 4 (Scheme 2, Table 1). All the observed 1H and 13C NMR spectroscopic data of this product could be explained in terms of this revised structure.
For additional structural support, 4 was tried to be converted to its acetate by reaction with acetic anhydride and pyridine. But the sole product, C12H9NO4 (HR FAB-MS), lacked the expected IR band for the α,β-unsaturated ester carbonyl and the ester methyl signals in its 1H and 13C NMR spectra. All other NMR signals of this product were noticeably similar to those of 4. The targeted acetyl derivative thus turned out to be the corresponding hydrolysed product, i.e. the carboxylic acid 5 (Scheme 2).
Here a base catalysed hydrolysis of the ester group in 4 occurred as pyridine was present in the reaction medium. This fact was additionally supported by two separate experiments. When 4 was dissolved in minimum amount of pyridine and in acetic anhydride separately and kept overnight at rt, 5 was formed as the only product (TLC) in the first case, whereas, no reaction took place in the latter case. The only snag in the characterisation of both 4 and 5 is that none of these compounds recorded the 1H NMR signals expected for the hydroxyl groups. In our opinion, DMSO-d6, used as the NMR solvent, caused deuterium exchange of the hydroxyl protons of the lactim as well as the carboxylic acid groups. The exact reason is not known but this type of lack of hydroxyl signals is a common observation in 1H NMR spectra when they are recorded in DMSO-d6. However, we are unable to provide any literature reference.
Since the reaction of 1a with 2f required nearly an hour, the reaction was carried out twice more - once by heating at 60-70 °C in an oven and separately under MWI (20% power). These experiments required 25 min and 12 min, respectively for completion to furnish the same product 4 in 75% and 72% yields (as against in 79% yield at rt). This outcome suggested that, in general, the carrying out of the reactions at rt is a better choice in terms of yield although reactions would require longer time periods.
With barbituric acid (2g), a little more than two equivalents of it was required for the complete consumption of isatin. The product analysed for C16H11N5O7, which suggested that it involved one isatin and two barbituric acid moieties with the exclusion of a molecule of water. Thus, it was not the expected Knoevenagel condensate (3ag), which had earlier been prepared from the same two substrates by carrying out the reaction in a mixture of glacial acetic acid and acetic anhydride.21, 22
The molecular formula further suggested that it should be the product of Michael addition at the initially formed Knoevenagel condensate (3ag). A search through the literature revealed that this compound, 3,3-bis(2´-barbituryl)oxindole (6) had also been prepared earlier from the same substrates by carrying out the reaction in refluxing methanol for a few hours.32 Indeed, these workers ascertained the correct structure of this compound as well as of its tetra-N-methyl derivative (prepared from isatin and N,N´-dimethylbarbituric acid in a similar manner) by 1H and 13C NMR spectroscopic analyses and also by a single crystal X-ray analysis of the latter. Strangely, this report32 did not mention the melting points of 6 and its tetra-N-methyl derivative, which prevented a comparison with our product.
We could, however, locate an early piece of work,25 in which 6 was prepared in 67% yield from the same substrates by heating for a few minutes in aqueous hydrochloric acid. The melting point of the product obtained by us agreed well with that reported for 6, thereby confirming the identity of the two. Our experiment with 2g and its outcome have been shown in Scheme 3. In view of the formation of 6 as the only product, irrespective of the mole ratio of the two substrates, as observed by us and also by others,32 it can be inferred that the initially formed Knoevenagel condensate (3ag) is too reactive a substrate for Michael addition to survive isolation. Our previous work33 on the facile (5 min) formation of 3,3-bis(3´-indolyl)oxindoles from a clay-mediated reaction of isatins with indoles at rt strengthens this view of ours.
The product from dimedone (2h) and isatin recorded a molecular formula of C24H27NO5 (HR EI-MS), which pointed to the inclusion of two dimedonyl moieties into the product in the same way as in the reaction of isatin with barbituric acid. It was subjected to detailed spectroscopic analyses (vide experimental). The product, bearing a tert-hydroxyl group, recorded signals for the lactam NH (δ 8.2, s) and the lactam carbonyl carbon (δ 181.8) along with a conspicuous CH signal at δ 110.7 (characteristic of CH-7 in oxindoles) in addition to three other aromatic CH signals, which demonstrated that the product indeed possessed a 3,3-disubstituted oxindole structure. Its 13C NMR spectrum also recorded two keto carbonyl carbons at δ 202.7 and 195.6, the Cα (δ 112.3) and the Cβ (δ 169.3) signals of a −O–Cβ=Cα–CO− moiety and a dioxygenated carbon (δ 101.2). These data clearly demonstrated that an active methylene proton of one dimedone moiety of the initially formed 3,3-bis(2′-dimedonyl)oxindole (3ah) underwent hemi-ketalisation, via its enolic hydroxyl, with the carbonyl group of the second dimedone moiety, thereby pointing to the spiro[octahydroxanthene-9,3′-oxindole] structure 7 for the product (Scheme 4, Table 1).
Only one point remained to be checked. Since the recyclability of any catalyst increases its utility, the reusability of neutral alumina was checked for three more cycles, taking the reaction of 1a with 2b at rt as the model reaction. From the second to fourth cycles, 3ab was isolated as the sole product in 95% (20 min), 83% (20 min) and 76% (30 min) yields, respectively. Clearly, the catalyst is recyclable with equal efficacy only for the second cycle for the substrate 2b. Although not tested for other substrates, the catalyst is likely to be similarly effective at least for the second cycle for them as well.
To conclude, the present work of ours demonstrated the efficacy of neutral alumina at rt to bring about the Knoevenagel condensation of isatin with many, but not all, active methylene compounds. The formation of a tandem Knoevenagel condensation-Michael addition product and a spiro compound constitutes additional dividends. The positive aspects of this method are that the method is mild and efficient, commercially available neutral alumina is inexpensive and the isolation of the products involves a mere leaching, all of which render the present piece of work a significant contribution.
EXPERIMENTAL
Melting points are uncorrected and were determined on a Toshniwal apparatus. IR spectra were recorded on a Nicolet Impact-400 FT-IR and Perkin Elmer RX1 FT-IR spectrophotometer. 1H (500 MHz) and 13C (125 MHz) NMR spectra (PND, DEPT-135, HMQC and HMBC) on a BRUKER DRX-500 NMR spectrometer, the LR EI-MS on a JEOL JMS-AX505HA and the HR EI/FAB-MS on a JEOL JMS-700 MStation mass spectrometer. Individual 1H and 13C NMR spectral assignments of 7 were ascertained from its HMQC and HMBC spectra. The five new compounds (3ca, cb, 4, 5, 7) were subjected to elemental analysis in a Perkin Elmer 2400 Series II C, H, N Analyser and the results were found to be in good agreement with the calculated values. The thin layer chromatographies (TLCs), both analytical and preparative, were carried out on silica gel G (Merck, India) plates. Aluminium oxide, active, neutral (SQ), bearing product no. 21285 was procured from Qualigens Fine Chemicals, India. Dry and distilled MeOH was used. PE refers to petroleum ether, bp 60-80 ºC. N-methylisatin and 5-nitroisatin were prepared by reported methods.34, 35
General experimental procedure. A mixture of an isatin (1a-c) (1.0 mmol) and an active methylene compound (1.0 mmol for 2a-f; 2.2 mmol for 2g, h) was dissolved in MeOH (0.5-1 mL), adsorbed uniformly on neutral alumina (1 g) and left in a hood at rt when the solvent slowly evaporated off. The progress of the reaction was monitered every 5 min (TLC) right from the point of adsorption. When the reactants were fully consumed, alumina was leached with 5% MeOH-EtOAc. The solvent was then distilled off, and the resulting residue was purified either by crystallisation from PE-EtOAc (for 3ab-ac, bb, ca, cb), MeOH-C6H6 (for 3ad), MeOH-EtOAc (for 4-6) or by preparative TLC, followed by crystallisation from MeOH-EtOAc (for 3ae) or PE-EtOAc (for 7).
Preparation of 5. Acetic anhydride (2.0 mmol) was added dropwise with stirring to an ice-cold solution of 4 (1.0 mmol) in pyridine (1 mL) and kept overnight at rt. When the reactants were consumed (TLC), the reaction mixture was diluted with benzene, and the resulting precipitate was filtered, washed with benzene till free from the smell of pyridine and purified by recrystallisation from MeOH-EtOAc to furnish 5.
Methyl 2-(2′-hydroxyindolenin-3′-ylidene)-3-oxobutyrate (4): mp 270-271 °C; IR (Nujol): 3428, 1720, 1702, 983, 768 cm-1; 1H NMR (DMSO-d6): δ 8.38 (1H, d, J=8.5 Hz), 7.85 (1H, d, J=8.5 Hz), 7.68 (1H, t, J=7.5 Hz), 7.47 (1H, t, J=7.5 Hz), 3.77 (3H, s), 2.57 (3H, s); 13C NMR: δ 170.0, 169.1, 155.3, 149.9, 148.3, 123.8, 123.6 (all C), 130.6, 128.9, 128.7, 126.3 (all CH), 52.9 (OCH3), 24.2 (COCH3); EI-MS: m/z (%) 227 (M–OH, 84), 213 (M–MeOH, 57), 169 (100); LR FAB-MS (+): m/z 246 (M+H)+, 268 (M+Na)+; HRMS (FAB+): calcd for C13H12NO4, 246.0766 (M+H)+; found 246.0743. Anal. Calcd for C13H11NO4: C, 63.67; H, 4.49; N, 5.71. Found: C, 63.73; H, 4.48; N, 5.73.
2-(2′-Hydroxyindolenin-3′-ylidene)-3-oxobutyric acid (5): mp 284-286 °C; IR (Nujol): 3429, 3224, 1653, 1613, 864, 777, 724 cm-1; 1H NMR (DMSO-d6): δ 8.05 (1H, d, J=8.5 Hz), 7.87 (1H, d, J=8.5 Hz), 7.67 (1H, t, J=7.5 Hz), 7.47 (1H, t, J=7.5 Hz), 2.65 (3H, s); 13C NMR: δ 171.3, 170.4, 156.2, 147.4, 144.5, 127.4, 123.2 (all C), 130.3, 128.8, 127.7, 126.6 (all CH), 24.8 (COCH3); EI-MS: m/z (%) 213 (M–H2O, 27), 169 (100), 141 (85); LR FAB-MS (+): m/z 232 (M+H)+, 254 (M+Na)+; HRMS (FAB+): calcd for C12H10NO4, 232.0610 (M+H)+; found: 232.0599; calcd for C12H9NO4+Na, 254.0430 (M+Na)+; found: 254.0438. Anal. Calcd for C12H9NO4: C, 62.33; H, 3.89; N, 6.06. Found: C, 62.25; H, 3.88; N, 6.09.
4a-Hydroxy-3,3,6,6-tetramethyl-1,8-dioxospiro[1,2,3,4,4a,5,6,7,8,9a-decahydroxanthene-9,3′-indolin-2′-one] (7): mp 232-234 °C; IR (KBr): 3330, 3105, 1723, 1693, 1649, 949, 904, 854, 793, 753 cm-1; 1H NMR (CDCl3): δ 8.85 (1H, d, J=1.5 Hz, 4a-OH), 8.25 (1H, s, NH), 7.16 (1H, dt, J1=7.5 Hz, J2=0.5 Hz, H-6'), 6.91 (1H, dt, J1=7.5 Hz, J2=0.5 Hz, H-5'), 6.86 (1H, d, J=7.5 Hz, H-7'), 6.81 (1H, d, J =7.5 Hz, H-4'), 3.52 (1H, s, H-9a), 2.45 (1H, d, J=18 Hz, H-5), 2.35 (1H, dd, J1=18 Hz, J2=1.5 Hz, H-5), 2.31 (1H, dd, J1=14 Hz, J2=2 Hz, H-4), 2.27 (1H, d, J=14 Hz, H-2), 2.20 (1H, d, J=16.5 Hz, H-7), 2.15 (1H, dd, J1=14 Hz, J2=2 Hz, H-2), 2.14 (1H, dd, J1=14 Hz, J2=2 Hz, H-4), 2.08 (1H, dd, J1=16.5 Hz, J2=1.5 Hz, H-7), 1.13 and 1.12 (3H each, s, 2×CH3-3), 1.06 and 1.03 (3H each, s, 2×CH3-6); 13C NMR: δ 202.7 (C-1), 195.6 (C-8), 181.8 (C-2'), 169.3 (C-10a), 143.6 (C-7'a), 132.8 (C-3'a), 128.8 (CH-6'), 122.8 (CH-5'), 120.9 (CH-4'), 112.3 (C-8a), 110.7 (CH-7'), 101.2 (C-4a), 60.9 (CH-9a), 55.2 (CH2-2), 51.1 (CH2-7), 48.4 (CH2-4), 47.0 (C-9/3'), 43.6 (CH2-5), 34.0 (C-3), 33.4 (CH3-3), 31.8 (C-6), 29.7 (CH3-6), 27.3 (CH3-3), 27.1 (CH3-6); EI-MS: m/z (%) 409 (M+, 33), 392 (29), 391 (100), 308 (26), 307 (84), 270 (45), 269 (58), 241 (35), 226 (84), 198 (29), 84 (23), 83 (66); HRMS (EI): calcd for C24H27NO5, 409.1889; found 409.1896. Anal. Calcd for C24H27NO5: C, 70.41; H, 6.60; N, 3.42. Found: C, 70.53; H, 6.57; N, 3.40.
2-(5′-Nitroindolin-2′-one-3′-ylidene)malononitrile (3ca): mp 260-261 °C; IR (Nujol): 3197, 2243, 1741, 1613, 1520, 1336, 1082, 943, 844, 724 cm-1; 1H NMR (DMSO-d6): δ 11.92 (1H, s), 8.63 (1H, d, J=2 Hz), 8.43 (1H, dd, J1=9 Hz, J2=2 Hz), 7.12 (1H, d, J=9 Hz); 13C NMR: δ 164.8, 152.0, 150.1, 143.2, 119.6, 113.3, 111.7, 84.3 (all C), 133.5, 121.5, 112.7 (all CH); EI-MS: m/z (%) 240 (M+, 100), 210 (46), 194 (12), 166 (24), 139 (31); HRMS (EI): calcd for C11H4N4O3, 240.0283; found 240.0283. Anal. Calcd for C11H4N4O3: C, 55.00; H, 1.66; N, 23.33. Found: C, 55.09; H, 1.67; N, 23.31.
Ethyl 2-(5′-nitroindolin-2′-one-3′-ylidene)cyanoacetate (3cb): mp 230-231 °C; IR (Nujol): 3350, 2223, 1752, 1714, 1619, 1599, 1519, 1345, 1082, 864, 751 cm-1; 1H NMR (DMSO-d6): δ 11.77 (1H, s), 9.12 (1H, d, J=2.5 Hz), 8.32 (1H, dd, J1=9 Hz, J2=2.5 Hz), 7.02 (1H, d, J=9 Hz), 4.42 (2H, q, J=7 Hz), 1.35 (3H, t, J=7 Hz); 13C NMR: δ 166.3, 161.8, 151.9, 145.0, 142.8, 119.7, 114.5, 108.2 (all C), 132.1, 126.1, 111.8 (all CH), 64.3 (CH2), 14.5 (CH3); EI-MS: m/z (%) 287 (M+, 100), 259 (11), 242 (50), 215 (61), 214 (15), 185 (20); HRMS (EI): calcd for C13H9N3O5, 287.0542; found 287.0533. Anal. Calcd for C13H9N3O5: C, 54.35; H, 3.13; N, 14.63. Found: C, 54.23; H, 3.15; N, 14.60.
ACKNOWLEDGEMENTS
The authors express their sincere thanks to the Director, Bose Institute for providing laboratory facilities, the C.S.I.R., Govt. of India for providing fellowship (R. M.), Mr. B. Majumder, NMR Facilities and Mr. P. Dey, Microanalytical Laboratory, both of Bose institute, for recording the spectra.
References
1. E. Knoevenagel, Chem. Ber., 1894, 27, 2345. CrossRef
2. G. Jones, ‘Organic Reactions,’ Vol. 15, ed. by A. C. Cope, John Wiley and Sons, NewYork, 1967, pp. 204-599; L. F. Tietze, U. Beifuss, ‘Comprehensive Organic Synthesis,’ Vol. 2, ed. by B. M. Trost, Pergamon Press, Oxford, 1991, pp. 341-394. CrossRef
3. A. T. Khan, T. Parvin, and L. H. Chowdhury, Tetrahedron, 2007, 63, 5593. CrossRef
4. Y. M. Ren and C. Cai, Synth. Commun., 2007, 37, 2209; CrossRef G. Bartoli, R. Beleggia, S. Giuli, A. Giuliani, E. Marcantoni, M. Massaccesi, and M. Paoletti, Tetrahedron Lett., 2006, 47, 6501; CrossRef C. Xu, J. K. Bartley, D. I. Enache, D. W. Knight, and G. J. Hutchings, Synthesis, 2005, 3468; CrossRef S. X. Wang, J.-T. Li, W.-Z. Yang, Y.-H. Yin, and Z.-H. Xie, Synth. Commun., 2004, 34, 829; CrossRef Y. Lu, Z. Ren, W. Cao, W. Tong, and M. Gao, Synth. Commun., 2004, 34, 2047; CrossRef D. Fildes, V. Caignaert, D. Villemin, and P.-A. Jaffrés, Green Chem., 2001, 3, 52; CrossRef H. Moison, F. Texier-Boullet, and A. Foucaud, Tetrahedron, 1987, 43, 537. CrossRef
5. T. Saito, H. Goto, K. Honda, and T. Fujii, Tetrahedron Lett., 1992, 33, 7535. CrossRef
6. D. C. Forbes, A. M. Law, and D. W. Morrison, Tetrahedron Lett., 2006, 47, 1699; CrossRef N. B. Darvatkar, A. R. Deorukhkar, S. V. Bhilare, and M. M. Salunkhe, Synth. Commun., 2006, 36, 3043; CrossRef Y. Hu, J. Chen, Z.-G. Le, and Q.-G. Zheng, Synth. Commun., 2005, 35, 739; CrossRef I. R. Harjani, S. J. Nara, and M. M. Salunkhe, Tetrahedron Lett., 2002, 43, 1127. CrossRef
7. M. Gupta and B. P. Wakloo, ARKIVOC, 2007, 94; S. Balalaie, M. Bararjanian, S. Hekmat, and P. Salehi, Synth. Commun., 2006, 36, 2549; CrossRef H. A. Oskooie, M. M. Heravi, F. Derikvand, M. Khorasani, and F. F. Bamoharram, Synth. Commun., 2006, 36, 2819; CrossRef M. L. Deb and P. J. Bhuyan, Tetrahedron Lett., 2005, 46, 6453; CrossRef Z. Ren, W. Cao, W. Tong, and X. Jing, Synth. Commun., 2002, 32, 1947; CrossRef F. Bigi, S. Carloni, L. Ferrari, R. Maggi, A. Mazzacani, and G. Sartori, Tetrahedron Lett., 2001, 42, 5203; CrossRef F. Bigi, M. L. Conforti, R. Maggi, A. Picciano, and G. Sartori, Green Chem., 2000, 2, 101. CrossRef
8. A. I. Khodair and J. Nielsen, Heterocycles, 2002, 57, 1017; CrossRef Y. Peng, G. Song, and X. Qian, J. Chem. Res. (S), 2001, 188; CrossRef J.-Tai Li, T.-S. Li, L.-J. Li, and X. Cheng, Ultrasonics Sonochemistry, 1999, 6, 199; P. de la Cruz, E. D.-Barra, A. Loupy, and F. Langa, Tetrahedron Lett., 1996, 37, 1113. CrossRef
9. L. Yue, W. Du, Y.-K. Liu, and Y.-C. Chen, Tetrahedron Lett., 2008, 49, 3881; CrossRef R. M. Shaker, A. F. Mahmoud, and F. F. Abdel-Latif, J. Chin. Chem. Soc., 2005, 52, 563; M. N. Elinson, S. K. Feducovich, Z. A. Starikova, A. N. Vereshchagin, S. V. Gorbunov, and G. I. Nikishin, Tetrahedron Lett., 2005, 46, 6389; CrossRef F. Al-Omran, A.-Z Elassar, and A. A. El-Khair, Tetrahedron, 2001, 57, 10163; CrossRef B. Pita, E. Sotelo, M. SuÁrez, E. Raviña, E. Ochoa, Y. Verdecia, H. Novoa, N. Blaton, C. de Ranter, and O. M. Peeters, Tetrahedron, 2000, 56, 2473; CrossRef H. Al-Awadhi, F. Al-Omran, M. H. Elnagi, L. Infantes, C. Foces-Foces, N. Jagerovic, and J. Elguero, Tetrahedron, 1995, 46, 12745; CrossRef S. Chatterjee, Science, 1967, 157, 314; CrossRef S. Chatterjee, J. Chem. Soc.(B), 1969, 725. CrossRef
10. F. F. AbdelLatif, R. M. Shaker, and N. S. AbdelAziz, Heterocycl. Commun., 1997, 3, 245; CrossRef J. M. Quintela, C. Peinador, and M. J. Moreira, Tetrahedron, 1995, 51, 5901; CrossRef F. F. AbdelLatif, Bull. Soc. Chim. Fr., 1990, 127, 129; F. Freeman, Chem. Rev., 1980, 80, 329; CrossRef A. J. Fatiadi, Synthesis, 1978, 165. CrossRef
11. M Chakrabarty, R. Mukherjee, A. Mukherji, S. Arima, and Y. Harigaya, Heterocycles, 2006, 68, 1659. CrossRef
12. M. Chakrabarty, R. Mukherjee, S. Karmakar, and Y. Harigaya, Monatsh. Chem., 2007, 138, 1279. CrossRef
13. M. Chakrabarty, N. Ghosh, R. Basak, and Y. Harigaya, Tetrahedron Lett., 2002, 43, 4705.
14. M. Chakrabarty, S. Sarkar, A. Linden, and B. K. Stein, Synth. Commun., 2004, 34, 1801. CrossRef
15. M. Chakrabarty, N. Ghosh, and Y. Harigaya, Tetrahedron Lett., 2004, 45, 4955. CrossRef
16. J. F. M. da Silva, S. J. Garden, and A. C. Pinto, J. Braz. Chem. Soc., 2001, 12, 273; CrossRef F. D. Popp, ‘Advances in Heterocyclic Chemistry,’ Vol. 18, ed. by A. R. Katritzky, Academic Press, Inc., London, 1975, pp. 2-58.
17. C. L. Woodard, Z. Li, A. K. Kathcart, J. Terrell, L. Gerena, M. Lopez-Sanchez, D. E. Kyle, A. K. Bhattacharjee, D. A. Nichols, W. Ellis, S. T. Prigge, J. A. Geyer, and N. C. Waters, J. Med. Chem., 2003, 46, 3877 and references (28-30) cited therein. CrossRef
18. L. Capuano, V. Diehl, and W. Ebner, Chem. Ber., 1972, 105, 3407. CrossRef
19. H. Junek, R. Dworezak, H. Sterk, and W. Fabian, Liebigs Ann. Chem., 1989, 1065. CrossRef
20. S. J. Garden, C. R. W. Guimarães, M. B. Corréa, C. A. F. de Oliveira, A. da Cunha Pinto, and R. B. de Alencastro, J. Org. Chem., 2003, 68, 8815. CrossRef
21. M. El-Badawi, E. S. I. Ibrahim, H. Farag, and M. M. Abbasi, J. Chin. Chem. Soc., 1991, 38, 277.
22. H. Schäfer, Arch. Pharm., 1970, 303, 183. CrossRef
23. G. Jones and W. J. Rae, Tetrahedron, 1966, 22, 3021. CrossRef
24. J. Harley-Mason and R. F. J. Ingleby, J. Chem. Soc., 1958, 3639. CrossRef
25. F. E. King, T. J. King, and G. B. Thompson, J. Chem. Soc., 1948, 552. CrossRef
26. E. Zrike and H. G. Lindwall, J. Am. Chem. Soc., 1936, 58, 49. CrossRef
27. H. G. Lindwall and A. J. Hill, J. Am. Chem. Soc., 1935, 57, 735. CrossRef
28. A. J. Hill and H. R. Henze, J. Am. Chem. Soc., 1924, 46, 2806. CrossRef
29. A. Dandia, H. Taneja, R. Gupta, and S. Paul, Synth. Commun., 1999, 29, 2323. CrossRef
30. K. Tanaka, ‘Solvent-free Organic Synthesis’, Wiley-VCH, Weinheim, 2003. CrossRef
31. S. Das, A. Pramanik, R. Fröhlich, and A. Patra, Tetrahedron, 2004, 60, 10197; CrossRef A. Schönberg and E. Singer, Chem. Ber., 1970, 103, 3871. CrossRef
32. B. S. Jursic and E. D. Stevens, Tetrahedron Lett., 2002, 43, 5681. CrossRef
33. M. Chakrabarty, S. Sarkar, and Y. Harigaya, J. Chem. Res., 2005, 540.
34. S. J. Garden, J. C. Torres, L. E. da Silva, and A. C. Pinto, Synth. Commun., 1998, 28, 1679. CrossRef
35. H. O. Calvery, C. R. Noller, and R. Adams, J. Am. Chem. Soc., 1925, 47, 3058 CrossRef