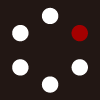
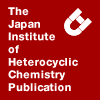
HETEROCYCLES
An International Journal for Reviews and Communications in Heterocyclic ChemistryWeb Edition ISSN: 1881-0942
Published online by The Japan Institute of Heterocyclic Chemistry
e-Journal
Full Text HTML
Received, 28th July, 2008, Accepted, 10th October, 2008, Published online, 14th October, 2008.
DOI: 10.3987/COM-08-S(F)64
■ Geranyl Derivatives of Salsolinol Show Increased Biological Activities
Kinuko Iwasa,* Suguru Okada, Yumi Nishiyama, Sousuke Takeuchi, Masataka Moriyasu, Chisato Tode, Makiko Sugiura, Atsuko Takeuchi, Harukuni Tokuda, Kazuyoshi Takeda, Yi-Nan Liu, Pei-Chi Wu, Kenneth F. Bastow, Toshiyuki Akiyama, and Kuo-Hsiung Lee
Kobe Pharmaceutical University, Motoyamakita, Higashinada, Kobe 658-8558, Japan
Abstract
Salsolinol and its N- and N,N-geranylated derivatives were tested for antimicrobial, cytotoxic, anti-oxidant, and anti-HIV activities, as well as inhibitory activity against Epstein-Barr virus early antigen (EBV-EA) activation induced by 12-O-tetradecanoylphorbol-13-acetate (TPA) in Raji cells. N,N-Geranylation increased potency in three different assays, antimicrobial, cytotoxic, and EBV-EA, while N-geranylation increased potency to a lesser extent in these same assays. N,N-Geranylated salsolinol was significantly active in all assays, except anti-HIV, and may be useful as a lead compound for developing potential chemotherapeutic agents.Salsolinol (1, 1-methyl-6, 7-dihydroxy-1, 2, 3, 4-tetrahydroisoquionoline), a dopamine (DA)-derived alkaloid, is one of the dopaminergic tetrahydroisoquinoline neurotoxins. It is present naturally in both rat1 and human2 brain, and is also found in certain foods, such as cheese and banana,3 and some alcoholic beverages, such as beer and wine.4 Salsolinol has several unique pharmacological activities.5 It has been reported that strong cancer preventive potential was found in flavonoids having a prenyl side-chain in the molecule, however, the inhibitory activities of compounds lacking a prenyl group were not fully estimated.6 From a pharmacological viewpoint, the addition of an isoprenoid chain frequently renders a molecule more effective than the parent compound.7 Thus, prenylated compounds represent a new frontier for the development of novel drugs, in particular, anti-microbial, anti-oxidant, anti-inflammatory, and anti-cancer agents. We have previously tested the antimicrobial, antimalarial, cytotoxic, anti-HIV, and anti-oxidant activities and inhibitory effects on EBV-EA induction of the isoquinoline alkaloids.8,9 Some of the tested isoquinolines showed significant activities in these assays. It was expected that addition of the prenyl group to the isoquinoline alkaloids would increase potency compared with the parent compounds in some assays. In this paper, we describe the synthesis of N-geranyl and N,N-geranyl salsolinol derivatives and compare their activities with those of salsolinol, N-methylsalsolinol, and N,N-dimethylsalsolinol in five assays, antimicrobial activity, cytotoxicity evaluation,10 inhibitory effects on EBV-EA induction,11 free radical scavenging activity, and anti-HIV activity.12
Geranylation of (±)-salsolinol (1) with geranyl bromide and NaH in N,N-dimethylformamide at RT for 4 h gave two geranylated products (2 and 3).
Compounds, 2 and 3 were separated by preparative HPLC using NH4OAc (0.05% TFA)-MeOH (0.05% TFA).
The molecular formula of compound 2 was determined to be C20H30NO2 by analysis of its HRSIMS
([M+1], m/z 316.2257), which indicated the presence of one geranyl group. The 1H NMR spectrum displayed two aromatic proton singlets at δ 6.62 and 6.57, a methine proton at δ 4.42 (q, J = 6.5 Hz), two methylene protons [δ 3.60 (1H, m) and 3.38 (1H, m), δ3.00 (1H, m) and 2.93 (1H, m)], and a methyl proton signal [δ 1.60 (3H, d, J = 6.5 Hz)], characteristic of an isoquinoline moiety, and three methyl proton signals at δ 1.70 (6H, s) and 1.64 (3H, s), two olefinic protons at δ 5.35 (t, J = 7.5 Hz) and 5.10 (m), and three methylene protons [δ 3.78 (1H, dd, J = 13.5, 7.5), δ 3.84 (1H, dd, J = 13.5, 7.5), and δ 2.19 (4H, m)], assignable to a geranyl group. An olefinic proton at δ 5.35 showed COSY coupling to the non-equivalent methylene protons at δ 3.78 and 3.84, which led to the assignment of H-2’ (δ 5.35) and H2-1’ (δ 3.78 and 3.84). The following COSY correlations were also observed: a methyl proton at δ 1.60 with a methine proton at δ 4.42, a methyl proton at δ 1.64 with an olefinic proton at δ 5.10 and two methyl protons at δ 1.70 with two olefinic protons at δ 5.10 and 5.35. One of the methyl signals at δ 1.70 should be assigned to the 3’-Me, and the olefinic proton signal at δ 5.10 was assigned as H-6’. The NOE correlations between an aromatic proton at δ 6.57 and both H-1 and 1-Me indicated that this aromatic proton was assigned as H-8. The aromatic proton at δ6.62, which correlated with the methylene protons at δ 2.93 and 3.00 (H2-4) was assigned as H-5. NOE correlations were also observed between the methine proton at δ 4.42 (1-H) and the methylene protons at δ 3.78 and δ 3.84 (H-1’), indicating a geranyl group was attached to nitrogen (N-2). Based on the above evidence, compound 2 was postulated to be 2-geranylated salsolinol. Moreover, the 13C NMR spectrum of 2 supported this assignment, with signals corresponding to nineteen carbons (one carbon was overlapped), including two protonated olefinic carbons (δ 124.62, 114.05), two protonated aromatic carbons (δ 115.97, 114.41), six quaternary carbons (δ 149.40, 147.04, 146.32, 133.22, 124.62, 121.96), five methylene carbons (δ 51.70, 44.69, 40.76, 26.98, 23.47), a methine carbon (δ 58.29), and four methyl carbons (δ 25.95, 21.38, 17.80, 16.71). The carbon signals at δ 44.69 (C-3) and 58.29 (C-1) displayed HMBC correlations with the proton signal at δ 3.78 (H-1’), confirming N-geranylation, which was suggested by the NOE correlation between H-1 and H-1’. Assignments of 1H and 13C signals of 2, obtained by 1D and 2D (1H-1H COSY, NOESY, HSQC, and HMBC) spectroscopic data, are listed in Table 1. On the basis of these data, the structure of 2 was established to be 2-geranylated salsolinol. The geometry of the disubstituted olefinic bond (between 2’ and 3’) was determined to be E on the basis of the NOE correlation between H-2’ and H-4’. NOE correlations were observed between H-3 (δ 3.60) and H-4 (δ 2.93) and between H-3 (δ 3.38) and H2-4 (δ 3.00 and 2.93), indicating axial-orientation of H-3 at δ 3.60. The NOE correlation between 1-Me and Hax-3 indicated axial-orientation of the methyl group at C-1. Configuration of the N-geranyl group is considered to be equatorial based on the NOE correlation between axial 1-Me and H-1’.
The molecular formula of compound 3 was determined to be C30H46NO2 by analysis of its HRSIMS (M+, m/z 452.3527), which indicated the presence of two geranyl groups. The 1H NMR spectrum showed two aromatic protons (δ 6.57, 6.64), two methylene protons [δ 3.67 (1H, m), 3.52 (1H, m), 3.03 (1H, m), 3.02 (1H, m)], a methine proton [δ 4.52 (1H, q, J = 6.5 Hz)], a methyl group [δ 1.65 (3H, d, J = 6.5 Hz)], indicating an isoquinoline moiety, and six methyl protons (δ 1.681 and 1.68 and δ 1.633 and 1.627, δ 1.81 and 1.52), four olefinic protons (δ 5.54 and 5.42, δ5.12 and 4.99), and six methylene protons [δ 2.25 (4H, m) and 2.20 (4H, m), δ 4.00 (1H, dd, J = 14.0, 7.5 Hz) and 3.89 (1H, dd, J = 14.0, 7.0 Hz), δ 3.98 (1H, dd, J = 14.0, 8.0 Hz) and 3.83 (1H, dd, J = 14.0, 6.5 Hz)], suggesting two sets of signals due to two geranyl groups. The 13C NMR spectrum displayed signals corresponding to twenty eight carbons (two carbons were overlapped), including a methine carbon [δ 65.33 (C-1)] and three deshielded methylene carbons (δ 57.56, 55.34, and 51.45), which suggested they were adjacent to the quaternary nitrogen. The methylene protons at δ 4.00 and 3.89 (H-1”) showed HMBC correlations with two carbons at δ 51.45 (C-3) and 55.34 (C-1’), and one of them (δ 4.00) also showed correlation with the carbon at δ 65.33 (C-1). Furthermore, HMBC correlations were observed from the methylene protons at δ 3.83 and/or 3.98 (H-1’) to the carbons at δ 57.56 (C-1”) and 65.33 (C-1). It was established that both geranyl groups were attached to nitrogen on the basis of these HMBC correlations, as well as NOESY correlations between the methine proton at δ 4.52 (H-1) and the olefinic protons (δ 5.42 and 5.54, H-2’ and H-2”) in the geranyl moiety. From assignments of 1H and 13C signals of 3 (Table 2) obtained by the analyses of the 1H-1H COSY, NOESY, HSQC, and HMBC spectra, compound 3 was determined to be 2, 2-digeranylated salsolinol. The geometries of the disubstituted olefinic bonds (between 2’ and 3’ and between 2” and 3’’) were determined to be E on the basis of the NOE correlations between H-2’ and H-4’ and H-2” and H-4”, respectively. The axial-orientation of the methyl group at C-1 was suggested by the NOE correlations between the methyl proton at δ 1.65 and axial proton (δ 3.67) of H2-3 (δ 3.67 and 3.52). Correlations were observed between this methyl signal and the methylene proton at δ 3.89 and 4.00 (H-1’’) and between H2-3 (axial and equatorial protons) and H-1’’ at δ 4.00, indicating equatorial orientation of the geranyl group (C-1’’).
(±)-N-Methylsalsolinol (4) was prepared by acid-catalyzed ether cleavage of (±)-carnegine.9 (±)-N,N-Dimethylsalsolinol (5) was obtained by N-methylation of 4. The molecular formula of compound 4 was determined to be C12H18NO2 by analysis of its HRSIMS (M+, m/z 208.1350).
The structures of 4 and 5 were determined by analysis of their 1H and 13C NMR and 2D NMR data (Tables 3 and 4).
Salsolinol (1), 2-geranylated salsolinol (2), 2, 2-digeranylated salsolinol (3), N-methylsalsolinol (4), and 2, 2-dimethylsalsolinol (5) were tested against Staphylococcus aureus (Gram-positive) and Escherichia coli (Gram-negative) by the liquid dilution method. The minimum inhibitory concentrations (MIC) are presented in Table 5.
N-Geranylation increased activity compared with salsolinol (1), N-methylsalsolinol (4), and N,N-dimethylsalsolinol (5), which were inactive. Notably, N,N-digeranylated salsolinol (3) displayed significant activity (7.8 μg/mL) against S. aureus. Thus, from these data, N-quaternization by N-geranylation, not simply N-alkylation, appears to be important for enhanced antimicrobial activity.
Salsolinol (1), as well as its N-geranylated (2 and 3), and N-methylated derivatives (4 and 5) were assayed for in vitro cytotoxicity against five human tumor cell lines, including lung carcinoma (A-549), prostate carcinoma (DU145), epidermoid carcinoma of the nasopharynx (KB), a drug-resistant KB-subline (KBvin), and human promyelocytic leukemia (HL-60). The cytotoxicity data are given as an ED50 value for each cell line, the concentration of compound that caused a 50% reduction in absorbance at 562 nm relative to untreated cells using SRB10 and MTT13 assays (HL60 is a non-adherent cell line; therefore, the SRB assay could not be used with it), and are shown in Table 6. The parent salsolinol (1) was inactive in all cell lines in both SRB and MTT assays.
N-Geranylsalsolinol (2) showed either weak or no activity against all cell lines in SRB and MTT assays, respectively, and N-methylsalsolinol (4) and N,N-dimethylsalsolinol (5) showed no activity in SRB assays. In comparison, N,N-digeranylsalsolinol (3) showed increased activity against DU-145, KB, and HL-60 cell lines. Compound 3 exhibited the highest potency (1.2 μg/mL) against the HL-60 cell line, and also displayed high activity (0.77 µg/mL) against this cell line in a WST-8 assay.14 Thus, N-geranylation, particularly digeranylation, increased cytotoxicity activity, while N-methylation, either mono or di, had no effect. The increase in cytotoxicity, as seen in 3 compared with 1, 2, 4 and 5, might be due to an increase in lipophilicity caused by additional geranyl moieties. Because KBvin cells were resistant to 3, the compound is likely a substrate for the p-glycoprotein drug efflux pump.
The Epstein-Barr virus early antigen (EBV-EA) activation assay is considered to be an effective indicator for the evaluation of anti-tumor-promoting activity.11 The inhibitory effects of 1-5 on EBV-EA activation induced by 12-O-tetradecanoylphorbol-13-acetate (TPA) in Raji cells were examined as a primary screening of anti-tumor-promoting activity. The inhibitory effects of the test compounds on TPA-induced EBV-EA activation, their effects on the viability of Raji cells, and the 50% inhibitory concentration (IC50) values are shown in Table 7. All compounds displayed stronger inhibition (IC50 296-384) than that of the reference compound, β-carotene (IC50 400), which has been studied extensively in cancer chemoprevention using animal models.15 The inhibitory activity was more increased by geranylation than methylation on nitrogen. N-Geranylation than N-methylation appears to be important for enhanced activity. 2, 2-Geranylated salsolinol (3) displayed the strongest inhibition, and its activity was higher than that of ginsenoside-Rg1, which is known as a strong anti-tumor-promoter.16 Thus, these compounds, especially N-geranylated derivatives appear to be useful leads for further development of potential cancer chemopreventive agents.
Several human illnesses, such as cancer, diabetes, atherosclerosis, etc., can be linked to the damaging action of reactive free radicals.17 The ability of compounds 1–3 to scavenge 1,1-diphenyl-2-picrylhydrazyl (DPPH) free radicals was examined, and the results are presented in Table 8. To evaluate the free radical scavenging activity of the compounds, the concentration required to scavenge DPPH free radicals by 50% (SC50) was determined.
The antioxidant α-tocopherol was used as the reference compound. All three compounds displayed similar potency and were more active than α-tocopherol. The phenolic hydroxyl groups on the aromatic rings may strongly influence the activity, without an affect from the geranyl group.
The isoquinoline alkaloids (1-3) were tested against HIV-1 replication in H9 lymphocytes to evaluate their anti-HIV activity. However, none of them displayed anti-HIV activity (Table 9).
In summary, among the tested biological activities of the simple isoquinolines 1-5, N,N-geranylation strongly increased potency in three assays, antimicrobial and cytotoxic activities and inhibitory effects on EBV-EA induction, while N-geranylation increased the same activities to a lesser extent. However, N, N- and N-methylation did not increase antimicrobial and cytotoxic activities. These N-geranylated isoquinolines also have free radical scavenging activity. These findings indicated that the N-geranyl group plays an important role in mediating these biological activities. In these present studies, we have identified new biologically active N-geranylated isoquinolines, which may be considered as lead structures for developing potential chemotherapeutic agents. Further experiments are ongoing to produce C- and/or O-geranyl derivatives.
#This paper is dedicated to Professor Emeritus Keiichiro Fukumoto on the occasion of his 75th birthday.
References
1. B. Sjoquist and E. Magnusson, J. Chromatogr., 1980, 183, 17.
2. B. Sjoquist, A. Eriksson, and B. Winblad, Prog. Clinical Biolog. Res., 1982, 90, 57.
3. R. M. Riggin, M. J. McCarty, and P. T. Kissinger, J.Agric. Food Chem., 1976, 24, 1891.
4. M. Duncan and G. Smythe, Lancet i (8277), 1982, 904.
5. R. Heikkila, G. Cohen, and D. Dembiec, J. Pharmacol. Exp. Ther., 1971, 179, 250; A. Giovine, M. Renis, and A. Bertolino, Pharmacology, 1976, 14, 86; CrossRef C. Weiner and M. Collins Biochemical Pharmacology, 1978, 27, 2699. CrossRef
6. M. Itoigawa, C. Ito, M. Ju-ichi, T. Nobukuni, E. Ichiishi, H. Tokuda, H. Nishino, and H. Furukawa, Cancer Lett., 2002, 176, 25. CrossRef
7. B. Botta, A. Vitali, P. Menendez, D. Misiti, and M. G. Delle, Curr. Med. Chem., 2005, 12, 717.
8. K. Iwasa, M. Moriyasu, Y. Tachibana, H.-S. Kim, Y. Wataya, W. Wiegrebe, K. F. Bastow, L. M. Cosentino, M. Kozuka, and K. H. Lee, Bioorg. Med. Chem., 2001, 9, 2871. CrossRef
9. W. H. Cui, K. Iwasa, H. Tokuda, A. Kashihara, Y. Mitani, T. Hasegawa,Y. Nishiyama, M. Moriyasu, H. Nishino, M. Hanaoka, C. Mukai, and K. Takeda, Phytochemistry, 2006, 67, 70. CrossRef
10. L. V. Rubinstein, R. H. Shoemaker, K. D. Paull, R. M. Simo, R. S. Tosini, P. Skehan, P. A.Scudiero, A. Monks, and M. R. Boyd, J. Natl. Cancer Inst., 1990, 82, 1113. CrossRef
11. Y. Ito, S. Yanase, J. Fujita, T. Harayama, T. Takashima, and H. Imanaka, Cancer Lett., 1981, 13, 29. CrossRef
12. Y. Kashiwada, F. Hashimoto, L. M. Cosentino, C. H. Chen, P. E. Garrett, and K. H. Lee, J. Med. Chem., 1996, 39, 1016.
13. T. Mosmann, J. Immunol. Methods, 1983, 16, 55. CrossRef
14. M. Ishiyama, Y. Miyazono, K. Sasamoto, Y. Ohkura, and K. Ueno, Taranta, 1997, 44, 1299.
15. A. Murakami, H. Ohigashi, and K. Koshimizu, Biosci. Biotechnol. Biochem., 1996, 60, 1.
16. K. Yasukawa, M. Takido, T. Matsumoto, M. Takeuchi, and S. Nakagawa, Oncology, 1991, 48, 72.
17. T. Konoshima, M. Takasaki, M. Kozuka, T. Nagao, H. Okabe, N. Irino, T. Nakasumi, H. Tokuda, and H. Nishino, Biol. Pharm. Bull., 1995, 18, 284.