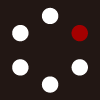
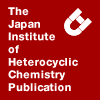
HETEROCYCLES
An International Journal for Reviews and Communications in Heterocyclic ChemistryWeb Edition ISSN: 1881-0942
Published online by The Japan Institute of Heterocyclic Chemistry
e-Journal
Full Text HTML
Received, 28th July, 2008, Accepted, 30th September, 2008, Published online, 2nd October, 2008.
DOI: 10.3987/COM-08-S(F)71
■ Nucleophilic Substitution Reaction in Indole Chemistry: 1-Methoxy-6-nitroindole-3-carbaldehyde as a Versatile Building Block for 2,3,6-Trisubstituted Indoles
Koji Yamada, Fumio Yamada, Takei Shiraishi, Saori Tomioka, and Masanori Somei*
Faculty of Pharmaceutical Sciences, Graduate School of Natural Science and Technology, Kanazawa University, Kakuma, Kanazawa, Ishikawa 920-1192, Japan
Abstract
1-Methoxy-6-nitroindole-3-carbaldehyde is proved to be a versatile electrophile and reacts regioselectively at the 2-position with various types of nucleophiles providing 2,3,6-trisubstituted indole derivatives. The reaction is applicable for the preparation of a novel pyrimido[1,2-a]indole derivative.Indole is one of the electron rich hetero-aromatics. In the indole chemistry, therefore, electrophilic substitution reaction has been well studied2 (as shown in general formula in Figure 1, A) and been applied to explain the biosyntheses of various types of indole alkaloids.2
It is evident, however, that some of natural products are difficult to produce by the electrophilic substitution reaction. Some examples are moroidin (1, Figure 2),3a phalloidin (2),3b goniomitine (3),3c and so on.3d They have either a C—N, a C—S, or a C—C bond at the 2 position of indole nucleus. Their syntheses require N+, S+, and C+ synthons, respectively. Except for S+ and C+, the N+ synthon is rarely available chemical species. If by chance a nucleophilic substitution reaction could be applied, their syntheses would become easy because the readily available N– synthon would be employed. In the cases of 2 and 3, the required S– and C– synthons have ample synthetic equivalents as well. Based on these ideas, we have thus far developed the unprecedented2,4 nucleophilic substitution reaction5 (as shown in general formula in Figure 1, B) simply by introducing a hydroxy or its modified group onto the nitrogen, N(1),5 of indole substrates.
In this paper, we wish to report that 1-methoxy-6-nitroindole-3-carbaldehyde (4) is an excellent substrate for achieving nucleophilic substitution reactions with variety of nucleophiles. Consequently, various types of 2,3,6-trisubstituted indole derivatives become readily available, providing useful building blocks for the syntheses of 1, 2, and 3. Preparation of a novel pyrimido[1,2-a]indole derivative is also reported.
According to our synthetic method,6 we prepared 1-methoxy-6-nitroindole (6) from indoline (5) in 70% overall yield in 3 steps as shown in Scheme 1. Subsequent Vilsmeier-Haack reaction of 6 with POCl3 and N,N-dimethylformamide (DMF) provided 1-methoxy-6-nitroindole-3-carbaldehyde (4) in 94% yield.
Thus, 4 is now readily available from 5 in 4 steps in 66% overall yield.
With 4 in hand, we examined its nucleophilic substitution reaction with nitrogen-centered nucleophiles. Using NaH as a base in DMF, piperidine was allowed to react with 4 at room temperature culminating in the formation of 7a in 92% yield (Table 1).
Under similar reaction conditions, pyrrole and indole provided 7b and 7c in 98 and 96% yields, respectively. Imidazole and benzimidazole also reacted with 4 providing 7d and 7e in the respective yields of 97 and 87%. Based on these successful results, we attempted the synthesis of 8, a core structure of 1. As expected, the reaction of Nα-Boc-L-histidine with 4 in DMF by the action of NaH as a base resulted in the formation of the desired Nα-Boc(3-formyl-6-nitroindol-2-yl)-L-histidine (8) in 94% yield.
We next examined NaSMe as a representative of sulfur-centered nucleophile. It reacted smoothly with 4 in DMF to afford 9 in 98% yield. In the next model experiment directed toward the phalloidin synthesis, N-acetyl-L-cysteine reacted successfully with 4 producing the expected N-acetyl-S-(3-formyl-6-nitroindol-2-yl)-L-cysteine (10) in 73% yield by the action of NaH in DMF.
As for a carbon nucleophile, we chose dimethyl malonate at first. In the presence of KOtBu in DMF at room temperature, it reacted with 4 to give 11 in 92% yield. Next, in order to prepare a suitable synthetic intermediate for 3, 3-acetylpyridine was allowed to react with 4 by the action of KH in THF. The desired 12 was successfully isolated in 92% yield.
On the other hand, we examined the reaction of 6 with p-chlorophenoxyacetonitrile (13) in the presence of KOtBu in DMF at 0 °C and isolated vicarious7 product 14 in 67% yield (Scheme 2). It should be noted that, under similar reaction conditions, attempts to convert 4 to 15 by the reaction with 13 resulted in the formation of a novel 4-amino-3-p-chlorophenoxy-2-p-chlorophenoxymethyl-7-nitropyrimido[1,2-a]indole-10-carbaldehyde (16) in 71% yield.
The structure of pyrimido[1,2-a]indole skeleton was determined as follows. First, 16 was converted to 10-methyl compound 17 in 93% yield by the treatment with Et3SiH in refluxing TFA. Subsequent reaction of 17 with Ac2O-pyridine at room temperature afforded 89% yield of 18. The structure of 18 was determined by X-ray single crystallographic analysis and the results are shown in ORTEP drawing in Figure 4.
To clear the reaction mechanism for the formation of 16, 13 was treated with KOtBu in DMF at 0 °C in the absence of 4 resulting in the formation of a 41% yield of 3-amino-2-butenonitrile 19 together with the recovery of 13. Therefore, we can propose the following possible mechanism as shown in Scheme 3.
By the reaction of KOtBu and 13, rapid formation of 19 occurs and it is converted to the corresponding N anion species 20. Subsequent nucleophilic attack at the 2-position of 4 together with the liberation of the methoxy group produces an intermediate 21. Then KOtBu abstracts the proton at the 2-position of 21. The resultant anion of indole nitrogen attacks the cyano group on the side chain to afford 22. Subsequent prototropy of the imine group completes the formation of 16.
In conclusion, we have demonstrated that 4 is an excellent electrophile and it reacts regioselectively at the 2-position with nitrogen, sulfur, and carbon centered nucleophiles. Since the 6-nitro group can be transformed into variety of functional groups, this methodology could be applied to the synthesis of various types of 2,3,6-trisubstituted indole derivatives and natural products. The formation of 16 suggests that if we treat 4 with nucleophiles having 3-amino-2-butenonitrile like synthon, the construction of new heterocycles would become possible.
EXPERIMENTAL
Melting points were determined on a Yanagimoto micro melting point apparatus and are uncorrected. Infrared (IR) spectra were recorded with a Shimadzu IR-420 and proton nuclear magnetic resonance (1H-NMR) spectra with a JEOL GSX-500 spectrometer with tetramethylsilane as an internal standard. Mass spectra (MS) were recorded on a JEOL JMS-SX102A or JEOL JMS-AX5 instruments. Optical rotations were determined on a Horiba SEPA-300 spectrometer. Column chromatography was performed on silica gel (SiO2, 100-200 mesh, from Kanto Chemical Co., Inc.) throughout the present study.
1-Methoxy-6-nitroindole-3-carbaldehyde (4) from 1-methoxy-6-nitroindole6 (6) — A mixture of POCl3 (0.97 mL, 10.6 mmol) and anhydrous DMF (5.84 mL, 75.1 mmol) was stirred at rt for 15 min. To the resulting mixture, a solution of 6 (1.00 g, 5.23 mmol) in anhydrous DMF (15 mL) was added and the mixture was stirred at rt for 7 h. After addition of H2O, the whole was made alkaline with saturated aqueous NaHCO3 and extracted with EtOAc. The extract was washed with brine, dried over Na2SO4, and evaporated under reduced pressure to leave a solid, which was column-chromatographed on SiO2 with EtOAc-hexane (1:2, v/v) to give 4 (1.08 g, 94 %). 4: mp 180-182 °C (yellow prisms, recrystallized from CHCl3-Hexane). IR (KBr): 1664, 1653, 1508, 1342 cm-1. 1H-NMR (CDCl3) δ: 4.28 (3H, s), 8.14 (1H, s), 8.22 (1H, dd, J = 8.8, 2.0 Hz), 8.43 (1H, d, J = 8.8 Hz), 8.45 (1H, d, J = 2.0 Hz), 10.02 (1H, s). Anal. Calcd for C10H8N2O4: C, 54.55; H, 3.66; N, 12.72. Found: C, 54.32; H, 3.61; N, 12.54.
6-Nitro-2-(piperidin-1-yl)indole-3-carbaldehyde (7a) from 4 — General procedure: A solution of piperidine (82.4 mg, 0.96 mmol) in anhydrous DMF (1 mL) was added to NaH (60% suspension in paraffin oil, 48.4 mg, 1.21 mmol) under ice cooling. The mixture was stirred at 0 °C for 30 min and then a solution of 4 (51.3 mg, 0.23 mmol) in anhydrous DMF (2 mL) was added. The reaction mixture was stirred at rt for 2.5 h. After addition of H2O, the whole was made acidic with saturated aqueous NH4Cl, and extracted with EtOAc. The extract was washed with brine, dried over Na2SO4, and evaporated under reduced pressure to leave a solid, which was column-chromatographed on SiO2 with CHCl3-MeOH (98:2, v/v) to give 7a (58.2 mg, 92%). 7a: mp >300 °C (yellow powder, recrystallized from acetone). IR (KBr): 1606, 1577, 1500, 1487, 1387, 1300 cm-1. 1H-NMR (DMSO-d6, 90 °C) δ: 1.68-1.75 (6H, m), 3.63-3.69 (4H, m), 7.91 (1H, dd, J = 8.5, 2.1 Hz), 7.95 (1H, d, J = 2.1 Hz), 8.00 (1H, d, J = 8.5 Hz), 10.00 (1H, s), 11.25 (1H, br s, disappeared on addition of D2O). MS m/z: 273 (M+). Anal. Calcd for C14H15N3O3·1/4H2O: C, 60.53; H, 5.66; N, 15.13. Found: C, 60.56; H, 5.56; N, 15.01.
6-Nitro-2-(pyrrol-1-yl)indole-3-carbaldehyde (7b) from 4 — In the general procedure, pyrrole (63.1 mg, 0.94 mmol), NaH (28.7 mg, 0.72 mmol), and 4 (50.0 mg, 0.23 mmol) were used. The reaction time was 3.5 h. After the work-up and column-chromatography with CHCl3-MeOH (98:2, v/v), 7b (56.6 mg, 98%) was obtained. 7b: mp >300 °C (yellow powder, recrystallized from acetone). IR (KBr): 1630, 1620, 1566, 1510, 1487, 1336 cm-1. 1H-NMR (DMSO-d6) δ: 6.50 (2H, t, J = 2.2 Hz), 7.55 (2H, t, J = 2.2 Hz), 8.14 (1H, dd, J = 8.8, 2.1 Hz), 8.26 (1H, d, J = 2.1 Hz), 8.31 (1H, d, J = 8.8 Hz), 10.06 (1H, s), 13.23 (1H, br s, disappeared on addition of D2O). MS m/z: 255 (M+). Anal. Calcd for C13H9N3O3·1/4H2O: C, 60.12; H, 3.49; N, 16.18. Found: C, 60.15; H, 3.63; N, 15.91.
2-(Indol-1-yl)-6-nitroindole-3-carbaldehyde (7c) from 4 — In the general procedure, indole (86.1 mg, 0.74 mmol), NaH (43.0 mg, 1.10 mmol), and 4 (51.6 mg, 0.24 mmol) were used. The reaction time was 2 h. After the work-up and column-chromatography with CHCl3, 7c (68.7 mg, 96%) was obtained. 7c: mp 291-293 °C (yellow powder, recrystallized from EtOAc). IR (KBr): 1630, 1618, 1558, 1508, 1483, 1383, 1327 cm-1. 1H-NMR (DMSO-d6) δ: 6.93 (1H, d, J = 3.4 Hz), 7.28 (1H, t, J = 7.8 Hz), 7.35 (1H, t, J = 7.8 Hz), 7.70 (1H, d, J = 7.8 Hz), 7.76 (1H, d, J = 7.8 Hz), 7.97 (1H, d, J = 3.4 Hz), 8.20 (1H, dd, J = 8.9, 2.5 Hz), 8.35 (1H, d, J = 2.5 Hz), 8.36 (1H, d, J = 8.9 Hz), 9.92 (1H, s), 13.49 (1H, br s, disappeared on addition of D2O). MS m/z: 305 (M+). Anal. Calcd for C17H11N3O3·1/4H2O: C, 65.91; H, 3.74; N, 13.56. Found: C, 66.11; H, 3.76; N, 13.29.
2-(Imidazol-1-yl)-6-nitroindole-3-carbaldehyde (7d) from 4 — In the general procedure, imidazole (53.2 mg, 0.77 mmol), NaH (39.8 mg, 1.00 mmol), and 4 (51.5 mg, 0.23 mmol) were used. The reaction time was 1.5 h. After the work-up and column-chromatography with CHCl3-MeOH (98:2, v/v), 7d (54.9 mg, 97%) was obtained. 7d: mp 268-272 °C (yellow powder, recrystallized from acetone). IR (KBr): 1660, 1510, 1331 cm-1. 1H-NMR (DMSO-d6) δ: 7.30 (1H, s), 7.94 (1H, s), 8.17 (1H, dd, J = 8.8, 2.2 Hz), 8.34 (1H, d, J = 2.2 Hz), 8.35 (1H, d, J = 8.8 Hz), 8.48 (1H, br s), 9.99 (1H, s). MS m/z: 256 (M+). Anal. Calcd for C12H8N4O3·1/4H2O: C, 55.28; H, 3.29; N, 21.49. Found: C, 55.53; H, 3.20; N, 21.31.
2-(Benzimidazol-1-yl)-6-nitroindole-3-carbaldehyde (7e) from 4 — In the general procedure, benzimidazole (86.1 mg, 0.71 mmol), NaH (28.3 mg, 0.71 mmol), and 4 (50.0 mg, 0.23 mmol) were used. The reaction time was 3.5 h. After the work-up and column-chromatography with EtOAc, 7e (60.4 mg, 87%) was obtained. 7e: mp >300 °C (yellow powder, recrystallized from MeOH). IR (KBr): 1672, 1502, 1338, 1203 cm-1. 1H-NMR (DMSO-d6) δ: 7.41-7.48 (2H, m), 7.75 (1H, dd, J = 7.3, 1.6 Hz), 7.87 (1H, dd, J = 7.3, 1.6 Hz), 8.21 (1H, dd, J = 8.8, 2.0 Hz), 8.40 (1H, d, J = 8.8 Hz), 8.41 (1H, d, J = 2.0 Hz), 8.87 (1H, s), 9.93 (1H, s), 13.65 (1H, br s, disappeared on addition of D2O). MS m/z: 306 (M+). Anal. Calcd for C16H10N4O3·1/2H2O: C, 60.95; H, 3.56; N, 17.77. Found: C, 61.02; H, 3.37; N, 17.49.
Nα-Boc-1-(3-formyl-6-nitroindol-2-yl)-L-histidine (8) from 4 — In the general procedure, Nα-Boc-L-histidine (208.7 mg, 0.82 mmol), NaH (58.0 mg, 1.21 mmol), and 4 (58.0 mg, 0.26 mmol) were used. The reaction time was 2 h. After the work-up and column-chromatography successively with CHCl3–MeOH–AcOH (46:10:1, v/v) and CHCl3–MeOH (6:4, v/v), 8 (110.3 mg, 94%) was obtained. 8: mp 125-136 °C (decomp., yellow fine needles, recrystallized from EtOAc). IR (KBr): 1655, 1518, 1340 cm-1. 1H-NMR (DMSO-d6) δ: 1.35 (9H, s), 2.93 (1H, dd, J = 14.7, 8.8 Hz), 3.00 (1H, dd, J = 14.7, 4.8 Hz), 4.27 (1H, td, J = 8.8, 4.8 Hz, collapsed to dd, J = 8.8, 4.8 Hz on addition of D2O), 7.05 (1H, d, J = 8.8 Hz, disappeared on addition of D2O), 7.64 (1H, s), 8.13 (1H, dd, J = 8.8, 2.0 Hz), 8.30 (1H, d, J = 8.8 Hz), 8.30 (1H, d, J=2.0 Hz), 8.39 (1H, br s), 9.98 (1H, s). FAB-MS m/z: 444 (M++1). Anal. Calcd for C20H21N5O7·1/2H2O: C, 53.10; H, 4.90; N, 15.48. Found: C, 53.11; H, 4.75; N, 15.41. [α]D23 +24.26° (c = 0.101, MeOH).
2-Methylthio-6-nitroindole-3-carbaldehyde (9) from 4 — A solution of 4 (51.4 mg, 0.23 mmol) and NaSCH3 (15% in water, 1 mL, 2.14 mmol) in DMF (2 mL) was refluxed for 1 h with stirring. After addition of H2O, the whole was made acidic with 6% HCl. The resulted precipitates (9, 54.2 mg, 98%) were collected by filtration and washed with EtOAc. 9: mp >300 °C (yellow powder, recrystallized from acetone). IR (KBr): 1626, 1608, 1500, 1311, 1298 cm-1. 1H-NMR (DMSO-d6) δ: 2.76 (3H, s), 8.07 (1H, dd, J = 8.6, 2.0 Hz), 8.11 (1H, d, J = 8.6 Hz), 8.25 (1H, d, J = 2.0 Hz), 10.10 (1H, s). Anal. Calcd for C10H8N2O3S·1/4H2O: C, 49.89; H, 3.56; N, 11.64. Found: C, 49.70; H, 3.29; N, 11.59.
N-Acetyl-S-(3-formyl-6-nitroindol-2-yl)-L-cysteine (10) from 4 — A solution of N-acetyl-L-cysteine (150.8 mg, 0.92 mmol) in anhydrous THF (3 mL) was added to a suspension of NaH (60% suspension in paraffin oil, 74.5 mg, 1.68 mmol) in anhydrous THF (2 mL) under ice cooling with stirring. Stirring was continued at rt for 10 min. After evaporation of the solvent, the residue was dissolved in DMF (3 mL). Then a solution of 4 (58.0 mg, 0.26 mmol) in anhydrous DMF (2 mL) was added and the reaction mixture was stirred at rt for 30 min. After addition of H2O, the whole was made acidic with 6% HCl, and extracted with EtOAc. The extract was washed with brine, dried over Na2SO4, and evaporated under reduced pressure to leave a solid, which was column-chromatographed on SiO2 with CHCl3–MeOH–AcOH (90:7:3, v/v) to give 10 (117.6 mg, 73%). 10: mp 212-214 °C (decomp., yellow prisms, recrystallized from MeOH). IR (KBr): 1722, 1630, 1610, 1518, 1335 cm-1. 1H-NMR (DMSO-d6) δ: 1.74 (3H, s), 3.55 (1H, dd, J = 13.7, 8.3 Hz), 3.67 (1H, dd, J = 13.7, 4.9 Hz), 4.47 (1H, td, J = 8.3, 4.9 Hz, collapsed to dd, J=8.3, 4.9 Hz on addition of D2O), 8.09 (1H, dd, J = 8.8, 2.2 Hz), 8.18 (1H, d, J = 8.8 Hz), 8.25 (1H, d, J = 2.2 Hz), 8.42 (1H, br d, disappeared on addition of D2O), 10.09 (1H, s) 12.97 (1H, br s, disappeared on addition of D2O). Anal. Calcd for C14H13N3O6S: C, 47.86; H, 3.73; N, 11.96. Found: C, 47.75; H, 3.79; N, 11.71. [α]D27 –29.60° (c=0.101, MeOH).
2-(3-Formyl-6-nitroindol-2-yl)malonic acid dimethyl ester(11)from 4 — A mixture of KOtBu (52.2 mg, 0.47 mmol) and dimethyl malonate (61.5 mg, 0.47 mmol) in anhydrous DMF (2 mL) was stirred at rt for 10 min. To the resulting mixture, a solution of 4 (51.2 mg, 0.23 mmol) in anhydrous DMF (2 mL) was added with stirring. Stirring was continued at rt for 30 min. After addition of H2O, the whole was made acidic with 6% HCl under ice cooling and extracted with CHCl3–MeOH (95:5,v/v). The extract was washed with brine, dried over Na2SO4, and evaporated under reduced pressure to leave a solid, which was column-chromatographed on SiO2 with CHCl3-MeOH (99:1,v/v) to give 11 (68.6 mg, 92%). 11: mp >300 °C (yellow needles, recrystallized from acetone-hexane). IR (KBr): 1741, 1651, 1512, 1342 cm-1. 1H-NMR (DMSO-d6) δ: 3.78 (6H, s), 6.13 (1H, s), 8.11 (1H, dd, J = 8.8, 2.2 Hz), 8.30 (1H, d, J = 8.8Hz), 8.43 (1H, d, J = 2.2 Hz), 10.21 (1H, s), 12.91 (1H, br s, disappeared on addition of D2O). MS m/z: 320 (M+). Anal. Calcd for C14H12N2O7: C, 52.50; H, 3.78; N, 8.75. Found: C, 52.29; H, 3.79; N, 8.63.
3-[2-(3-Formyl-6-nitroindol-2-yl)acetyl]pyridine (12) from 4 — 3-Acetylpyridine (69.6 mg, 0.57 mmol) was added to a suspension of KH (35% suspension in paraffin oil, 77.5 mg, 0.68 mmol) in anhydrous THF (4 mL), and the mixture was stirred at 0 °C for 10 min. To the resulting mixture, a solution of 4 (100.8 mg, 0.46 mmol) in anhydrous THF (5 mL) was added and the mixture was stirred at rt for 1 h. After addition of H2O, the whole was extracted with EtOAc. The water layer was made neutral with 6% HCl, and extracted with EtOAc. The combined extract was washed with brine, dried over Na2SO4, and evaporated under reduced pressure to leave a solid, which was column-chromatographed on SiO2 with EtOAc-MeOH (99:1,v/v) to give 12 (129.3 mg, 92%). 12: mp >300 °C (orange amorphous solid, recrystallized from acetone-hexane). IR (KBr): 3114, 1701, 1639, 1585, 1504, 1468, 1338, 1298 cm-1. 1H-NMR (DMSO-d6, 60 °C) δ: 5.13 (2H, s), 7.61 (1H, dd, J = 7.8, 4.9 Hz), 8.07 (1H, br d, J = 8.8 Hz, collapsed to d on addition of D2O), 8.26 (1H, d, J = 8.8 Hz), 8.39 (1H, br s, collapsed to d on addition of D2O), 8.42 (1H, br d, J = 7.8 Hz, collapsed to ddd on addition of D2O), 8.84 (1H, br d, J = 4.9 Hz, collapsed to dd on addition of D2O), 9.27 (1H, s), 10.16 (1H, s), 12.47 (1H, br s, disappeared on addition of D2O). MS m/z: 309 (M+). Anal. Calcd for C16H11N3O4: C, 62.13; H, 3.59; N, 13.59. Found: C, 61.90; H, 3.56; N, 13.38.
7-Cyanomethyl-1-methoxy-6-nitroindole (14) from 6 — KOtBu (155.5 mg, 1.39 mmol) was added to a solution of 6 (52.8 mg, 0.28 mmol) and 13 (139.6 mg, 0.83 mmol) in DMF (1 mL) under ice cooling with stirring. Stirring was continued at 0 °C for 5 min. After addition of H2O, the whole was made acidic with 6% HCl, and extracted with EtOAc. The extract was washed with brine, dried over Na2SO4, and evaporated under reduced pressure to leave a residue, which was column-chromatographed on SiO2 with EtOAc-hexane (1:5, v/v) to give 14 (42.4 mg, 67%). 14: mp 148-150 °C (yellow needles, recrystallized from CHCl3-hexane). IR (KBr): 2256, 1514, 1493, 1335, 1317 cm-1. 1H-NMR (CDCl3) δ: 4.23 (3H, s), 4.55 (2H, s), 6.56 (1H, d, J = 3.4 Hz), 7.58 (1H, d, J = 3.4 Hz), 7.65 (1H, d, J = 8.8 Hz), 7.29 (1H, d, J = 8.8 Hz). Anal. Calcd for C11H9N3O3: C, 57.14; H, 3.92; N, 18.17. Found: C, 57.11; H, 3.86; N, 18.04.
4-Amino-3-p-chlorophenoxy-2-p-chlorophenoxymethyl-7-nitropyrimido[1,2-a]indole-10-carbaldehyde (16) from 4 and p-chlorophenoxyacetonitrile (13) — A mixture of KOtBu (156.3 mg, 1.39 mmol) and 13 (229.1 mg, 1.37 mmol) in anhydrous DMF (3 mL) was stirred at 0 °C for 30 min. To the resulting mixture, a solution of 4 (100.1 mg, 0.46 mmol) in anhydrous DMF (3 mL) was added with stirring. Stirring was continued at rt for 10 min. After addition of saturated aqueous NH4Cl, the whole was extracted with EtOAc. The extract was washed with brine, dried over Na2SO4, and evaporated under reduced pressure to leave a solid, which was column-chromatographed on SiO2 with acetone-hexane (1:3, v/v) to give 16 (169.5 mg, 71%). 16: mp 242-244 °C (yellow powder, recrystallized from acetone-hexane). IR (KBr): 1628, 1585, 1510, 1485, 1356, 1336 cm-1. 1H-NMR (DMSO-d6) δ: 5.02 (2H, s), 6.84 (2H, d, J = 9.0 Hz), 7.11 (2H, d, J = 9.0 Hz), 7.26 (2H, d, J = 9.0Hz), 7.34 (2H, d, J = 9.0 Hz), 8.44 (1H, dd, J = 8.8, 1.7 Hz), 8.49 (1H, d, J = 8.8 Hz), 8.60 (2H, br s, disappeared on addition of D2O), 9.33 (1H, br s), 10.32 (1H, s). Anal. Calcd for C25H16Cl2N4O5: C, 57.38; H, 3.08; N, 10.71. Found: C, 57.13; H, 3.10; N, 10.56.
4-Amino-3-p-chlorophenoxy-2-p-chlorophenoxymethyl-10-methyl-7-nitropyrimido[1,2-a]indole (17) from 16 — A mixture of 16 (113.1 mg, 0.22 mmol) and Et3SiH (0.11 mL, 0.69 mmol) in TFA (5 mL) was refluxed for 30 min with stirring. After evaporation of the solvent, the resulting solid was column-chromatographed on SiO2 with CHCl3 to give 17 (102.9 mg, 93%). 17: mp 240-241 °C (decomp., brown fine needles, recrystallized from EtOAc). IR (KBr): 1523, 1489, 1483, 1468, 1308, 1284, 1275, 1232 cm-1. 1H-NMR (CDCl3) δ: 2.61 (3H, s), 5.06 (2H, s), 5.53 (2H, br s, disappeared on addition of D2O), 6.71 (2H, d, J = 9.0 Hz), 6.90 (2H, d, J = 9.0 Hz), 7.16 (2H, d, J = 9.0 Hz), 7.26 (2H, d, J = 9.0 Hz), 7.81 (1H, d, J = 9.0 Hz), 8.34 (1H, dd, J = 9.0, 2.0 Hz), 8.97 (1H, d, J = 2.0 Hz). Anal. Calcd for C25H18Cl2N4O4: C, 58.95; H, 3.56; N, 11.00. Found: C, 58.88; H, 3.56; N, 10.90.
4-Diacetylamino-3-p-chlorophenoxy-2-p-chlorophenoxymethyl-10-methyl-7-nitropyrimido[1,2-a]indole (18) from 17 — Ac2O (1 mL) was added to a solution of 17 (49.0 mg, 0.10 mmol) in pyridine (2 mL) and stirred at rt for 1 h. After evaporation of the solvent, the resulting solid was column-chromatographed on SiO2 with CHCl3-hexane (2:1, v/v) to give 18 (50.7 mg, 89%). 18: mp 217-219 °C (decomp., orange prisms, recrystallized from EtOAc). IR (KBr): 1736, 1724, 1520, 1489, 1477, 1363, 1331, 1311, 1242, 1223, 1203 cm-1. 1H-NMR (CDCl3) δ: 2.38 (6H, s), 2.69 (3H, s), 5.07 (2H, s), 6.77 (2H, d, J = 9.0 Hz), 6.86 (2H, d, J = 9.0 Hz), 7.20 (2H, d, J = 9.0 Hz), 7.23 (2H, d, J = 9.0 Hz), 7.93 (1H, d, J = 9.0 Hz), 8.32 (1H, dd, J = 9.0, 2.0 Hz), 8.57 (1H, d, J = 2.0 Hz). Anal. Calcd for C29H22Cl2N4O6: C, 58.70; H, 3.74; N, 9.44. Found: C, 58.83; H, 3.69; N, 9.42.
(E)-3-Amino-2,4-di(p-chlorophenoxy)-2-butenonitrile (19) from 13 — A mixture of KOtBu (69.3 mg, 0.62 mmol) and 13 (102.3 mg, 0.61 mmol) in anhydrous DMF (2 mL) was stirred at 0 °C for 30 min. After addition of H2O, the whole was made acidic with 6% HCl, and extracted with EtOAc. The extract was washed with brine, dried over Na2SO4, and evaporated under reduced pressure to leave a residue, which was column-chromatographed on SiO2 with CHCl3-hexane (1:1, v/v) to give unreacted 14 (27.4 mg, 27%) and 19 (41.5 mg, 41%) in the order of elution. 19: mp 101-101.5 °C (colorless fine needles, recrystallized from CHCl3–hexane). IR (KBr): 3473, 3361, 2197, 1645, 1489, 1485, 1205, 827 cm-1. 1H-NMR (CDCl3) δ: 4.73 (2H, br s, disappeared on addition of D2O), 4.86 (2H, s), 6.92-6.95 (4H, m), 7.29 (2H, d, J = 9.0 Hz), 7.31 (2H, d, J = 9.0 Hz). Anal. Calcd for C16H12Cl2N2O2: C, 57.33; H, 3.61; N, 8.36. Found: C, 57.14; H, 3.61; N, 8.33. Two protons attached to the amino group appeared as a singlet proving that they are equivalent and 19 is an E isomer. If 19 is a Z isomer, the two protons should be non-equivalent because of hydrogen bonding to phenoxy oxygen.
X-Ray Crystallographic Analysis of 18 — The reflection data were collected at rt on a Rigaku AFC-5R diffractometer with graphite monochromated CuKα radiation (λ=1.54178 Å). The structures were solved by direct methods using MITHRIL and refined by the full-matrix least squares. The non-hydrogen atoms were refined anisotropically. All calculations were performed using the teXsan crystallographic package.
# Dedicated to the 75th birthday of Professor Emeritus Keiichiro Fukumoto
† Sumika Technoservice Corporation. Present address: 2-1-4 Takatsukasa, Takarazuka-shi, Hyogo, 665-0051, Japan. ‡ Professor Emeritus of Kanazawa University. Present address: 2-40-3 Sodani, Hakusan-shi, Ishikawa, 920-2101, Japan.
References
1. a) This report is part 134 of a series entitled “The Chemistry of Indoles” and a full report of the previous communication: K. Yamada, F. Yamada, T. Shiraishi, S. Tomioka, and M. Somei, Heterocycles, 2002, 58, 53; CrossRef b) Part 133: K. Yoshino, F. Yamada, and M. Somei, Heterocycles, 2008, 76, in press. CrossRef
2. R. J. Sundberg, “Indoles”, Academic Press, 1996; R. T. Brown, J. A. Joule, and P. G. Sammes, “Comprehensive Organic Chemistry”, Vol. 4, Pergamon Press, 1979, pp. 411-492; R. J. Sundberg, “The Chemistry of Indoles”, Academic Press, 1970.
3. a) S. D. Kahn, P. M. Booth, J. P. Waltho, and D. H. Williams, J. Org. Chem., 2000, 65, 8406; CrossRef idem., ibid., 1989, 54, 1901; CrossRef T.-W. C. Leung, D. H. Williams, J. C. J. Barna, and S. Foti, Tetrahedron, 1986, 42, 3333; CrossRef b) L. Falcigno, S. Costantini, G. D‘Auria, B. M. Bruno, S. Zobeley, G. Zanotti, and L. Paolillo, Chem. Eur. J., 2001, 7, 4665 and references cited therein; CrossRef F. Lynen and U. Wieland, Liebigs Ann. Chem., 1938, 548, 1938; c) L. Randriambola, J.C. Quirion, C. Kan-Fan, and H.P. Husson, Tetrahedron Lett., 1987, 28, 2123; CrossRef d) J. Kobayashi, H. Suzuki, K. Shimbo, K. Takeya, and H. Morita, J. Org. Chem., 2001, 66, 6626; ; CrossRef M. Mure and K. Tanizawa, Biosci. Biotech. Biochem., 1997, 61, 410 and references cited therein.
4. Nucleophilic substitution reactions were suggested in the following literatures: P. H. H. Hermkens, R. Plate, C. G. Kruse, H. W. Scheeren, and H. C. J. Ottenheijm, J. Org. Chem., 1992, 57, 3881; CrossRef J. Becher, P. L. Jfrgensen, K. Pluta, N. J. Krake, and B. F. Hansen, ibid., 1992, 57, 2127; CrossRef R. M. Acheson, “Advances in Heterocyclic Chemistry”, Vol. 51, Academic Press, 1990, pp. 105-175; T. Nagayoshi, S. Saeki, and M. Hamana, Chem. Pharm. Bull., 1984, 32, 3678; L. Dalton, G. L. Humphrey, M. M. Cooper, and J. A. Joule, J. Chem. Soc., Perkin Trans. 1, 1983, 2417; CrossRef M. G. Beal, W. R. Ashcroft, M. M. Cooper, and J. A. Joule, ibid., 1982, 435; CrossRef M. M. Cooper, G. J. Hignett, and J. A. Joule, ibid., 1981, 3008; CrossRef M. De. Rosa, L. Carbognani, and A. Febres, J. Org. Chem., 1981, 46, 2054; CrossRef D. V. C. Awang and A. Vincent, Can. J. Chem., 1980, 58, 1589; CrossRef M. De. Rosa and J. L. T. Alonsa, J. Org. Chem., 1978, 43, 2639; CrossRef R. M. Acheson, J. Chem. Soc., Perkin Trans. 1, 1978, 1117; CrossRef T. Hino, M. Endo, M. Tonozuka, Y. Hashimoto, and M. Nakagawa, Chem. Pharm. Bull., 1977, 25, 2350; P. G. Gassman, G. A. Campbell, and G. Mehta, Tetrahedron, 1972, 28, 2749; CrossRef J. Billet and S. G. Smith, Tetrahedron Lett., 1969, 4465; R. J. Sundberg, J. Org. Chem., 1965, 30, 3604. CrossRef
5. M. Somei, Yakugaku Zasshi, 2008, 128, 527; CrossRef M. Somei, Heterocycles, 2008, 75, 1021; CrossRef M. Somei, “Advances in Heterocyclic Chemistry”, Vol. 82, ed. by A. R. Katritzky, Elsevier Science, USA, 2002, pp. 101-155; M. Somei, Heterocycles, 1999, 50, 1157; CrossRef M. Somei, J. Synth. Org. Chem. Jpn., 1991, 49, 205; M. Somei and T. Kawasaki, Heterocycles, 1989, 29, 1251. CrossRef
6. a) K. Yamada, S. Tomioka, N. Tanizawa, and M. Somei, Heterocycles, 2004, 63, 1601; CrossRef b) K. Yamada, T. Kawasaki, Y. Fujita, and M. Somei, ibid., 2001, 55, 1151; CrossRef c) M. Somei and T. Kawasaki, ibid., 1989, 29, 1251. CrossRef
7. Vicarious reaction: M. Makosza and J. Winiarski, Acc. Chem. Res., 1987, 20, 282 CrossRef