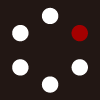
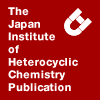
HETEROCYCLES
An International Journal for Reviews and Communications in Heterocyclic ChemistryWeb Edition ISSN: 1881-0942
Published online by The Japan Institute of Heterocyclic Chemistry
e-Journal
Full Text HTML
Received, 31st July, 2008, Accepted, 5th September, 2008, Published online, 9th September, 2008.
DOI: 10.3987/COM-08-S(F)82
■ Reaction of 9-Germaphenanthrene with Dimethyl Acetylenedicarboxylate: Unexpected Formation of a 1,2-Oxagermolane Derivative
Yoshiyuki Mizuhata, Koji Inamura, and Norihiro Tokitoh*
Institute for Chemical Research, Kyoto University, Uji, Kyoto 611-0011, Japan
Abstract
The reaction of 9-germaphenanthrene with dimethyl acetylenedicarboxylate (DMAD) in benzene afforded a 1:2 adduct containing 1,2-oxagermolane and cyclopentadiene units, the structure and generation mechanism of which are discussed based on X-ray structural analysis and theoretical calculations.The chemistry of “heavy aromatic compound,” [4n+2]π-electron ring systems containing a heavier group 14 element (Si, Ge, Sn, Pb),1-3 has attracted attention from the viewpoint of comparison with the parent aromatic hydrocarbons, which play very important roles in organic chemistry. Although heavy aromatic compounds have been known to be highly reactive and undergo ready dimerization or oligomerization under ambient conditions, we have succeeded in the synthesis of the first stable neutral sila-, germa-, and stanna-aromatic compounds,2 heavier analogues of benzene (Si,4 Ge5), naphthalene (Si,6,7 Ge,8 Sn9), anthracene (Si,10 Ge11), and phenanthrene [Si,12 Ge11 (Sn:13 unstable)], by taking advantage of kinetic stabilization using efficient steric protection groups, 2,4,6-tris[bis(trimethylsilyl)methyl]phenyl (Tbt) and 2,6-bis[bis(trimethylsilyl)methyl]-4-[tris(trimethylsilyl)methyl]phenyl (Bbt). Although these compounds were found to have sufficient aromatic characters judging from their structures and spectroscopic properties, they still possess high reactivity as a metallene (>M=C<, M = Si, Ge, Sn, Pb). Actually, they react with protic reagents (water, alcohol), multiple-bond species (olefin, alkyne, ketone, nitrile oxide), and elemental chalcogens (S8, Se) to afford the corresponding 1,2- or 1,4-adducts. As for a parent phenanthrene, on the other hand, it is well known that the aromaticity of the central ring is lower than those of the other rings and that the bond between 9 and 10 positions has a considerable double-bond character. Therefore, the investigation of the reactivity of heavy phenanthrene systems is important as a mediator between the typical metallenes (>M=C<) and heavy aromatic compounds. During the course of our studies on heavy aromatic compounds, we have found the unique reactivity of Tbt-substituted 9-germaphenanthrene 111 with dimethyl acetylenedicarboxylate (DMAD) giving 1:2 adduct 2. In this paper, we describe the structure of the product 2 and the reaction mechanism in detail.
The reaction of 9-germaphenanthrene 1 with DMAD in benzene afforded the 1:2 adduct 2 in 40% yield. There was no evidence for the generation of [2+2]-adduct 3 in contrast to the reactions of the Tbt-substituted sila- or germa-benzenes with phenylacetylene giving the corresponding [4+2]-adducts in which the acetylene worked as dienophile.4,5 Although many examples of cycloaddition reaction together with two DMAD molecules were reported, there are a few ones giving cyclopentadiene skeleton.14,15
The structure of 2 was supported by the 1H and 13C NMR and mass spectra16 and finally determined by X-ray crystallographic analysis.17 The whole and core structures of 2 were shown in Figure 1 together with the observed bond lengths of 2 and the calculated ones for the model compound 2’ bearing a methyl substituent instead of a Tbt group of 2. The fragment consisted of two DMAD molecules was found to add in a syn-fashion toward the 9-germaphenanthrene ring. The Ge1–C9 bond in 2 [2.0134(16) å] is one of the longest Ge–C single bonds ever-known and markedly differs in length from those of the bonds Ge1–C13 and Ge1–C26, which lie in the normal range for Ge–C single bonds. Although the elongation of Ge–C9 bond was supported by the theoretical calculations, the observed and calculated values for Ge–C9 were quite different from each other. This result indicated that the main factor of the elongation was the skeletal strain, which depends on the substituent sizes. The calculated values except for Ge1–C9 were in good agreement with the observed ones.
The plausible mechanism for the reaction of 1 with DMAD is shown in Scheme 2. The initial step can be explained by the 1,3-dipolar addition of 1 with zwitterion 4, which was suggested as a reactive intermediate in thermal tetramerization of DMAD itself.18 Regioselectivity was resulted from the polarity of the germene moiety (Geδ+=Cδ–). The addition of another DMAD molecule to the remaining carbene center in the intermediate 5 followed by the ring opening rearrangement may result in the formation of 2. Similar reaction pathway has already been postulated in the reaction of the strained anthracene having a short methylene linker between 1 and 4 positions with DMAD.15
In order to estimate the reaction pathway, theoretical calculations for model compounds 1’-3’, 5’, 6’ bearing a methyl substituent instead of a Tbt group of 1-3, 5, 6 were performed. The energies of the optimized structures calculated at B3LYP/6-31G(d) level were summarized in Figure 2. As for the initial step, the energy of [2+3]-adduct 5’ was higher than that of the [2+2]-adduct 3’. However, the generations of 6’ and 2’ by the addition of DMAD to 5’ were highly exothermic. These results imply the route via 5’ might be more favorable than that giving 3’, although the reaction pathways should be discussed on the basis of the energies of transition states in a strict sense.
In summary, we have found the unique reactivity of 9-germaphenanthrene with DMAD. Systematic investigation on the reactivity of other heavy aromatic compounds, especially 9-silaphenanthrene, with DMAD is currently in progress.
ACKNOWLEDGEMENTS
This work was partially supported by Grants-in-Aid for Creative Scientific Research (No. 17GS0207) and the Global COE program B09 (International Center for Integrated Research and Advanced Education in Material Science) from Ministry of Education, Culture, Sports, Science and Technology, Japan.
# Dedicated to Professor Emeritus Keiichiro Fukumoto on the occasion of his 75th birthday.
References
1. M. Haaf, T. A. Schmedake, and R. West, Acc. Chem. Res., 2000, 33, 704; CrossRef V. Y. Lee, A. Sekiguchi, M. Ichinohe, and N. Fukaya, J. Organomet. Chem., 2000, 611, 228; CrossRef A. Sekiguchi and V. Y. Lee, Chem. Rev., 2003, 103, 1429; CrossRef V. Y. Lee and A. Sekiguchi, Angew. Chem. Int. Ed., 2007, 46, 6596. CrossRef
2. N. Tokitoh, Acc. Chem. Res., 2004, 37, 86. CrossRef
3. A. Sekiguchi, M. Tsukamoto, and M. Ichinohe, Science, 1997, 275, 60; CrossRef S. B. Choi, P. Boudjouk, and P. R. Wei, J. Am. Chem. Soc., 1998, 120, 5814; CrossRef T. Nishinaga, Y. Izukawa, and K. Komatsu, J. Am. Chem. Soc., 2000, 122, 9312; CrossRef M. Saito, R. Haga, and M. Yoshioka, Chem. Commun., 2002, 1002; CrossRef M. Saito, R. Haga, and M. Yoshioka, Chem. Lett., 2003, 32, 912; CrossRef M. Saito, R. Haga, M. Yoshioka, K. Ishimura, and S. Nagase, Angew. Chem. Int. Ed., 2005, 44, 6553; CrossRef M. Ichinohe, M. Igarashi, K. Sanuki, and A. Sekiguchi, J. Am. Chem. Soc., 2005, 127, 9978; CrossRef V. Y. Lee, R. Kato, M. Ichinohe, and A. Sekiguchi, J. Am. Chem. Soc., 2005, 127, 13142; CrossRef S. Ishida, T. Nishinaga, R. West, and K. Komatsu, Chem. Commun., 2005, 778; CrossRef M. Driess, S. Yao, M. Brym, and V.-C. Wullen, Angew. Chem. Int. Ed., 2006, 45, 6730; CrossRef R. Kinjo, M. Ichinohe, A. Sekiguchi, N. Takagi, M. Sumimoto, and S. Nagase, J. Am. Chem. Soc., 2007, 129, 7766; CrossRef M. Igarashi, M. Ichinohe, and A. Sekiguchi, J. Am. Chem. Soc., 2007, 129, 12660. CrossRef
4. K. Wakita, N. Tokitoh, R. Okazaki, and S. Nagase, Angew. Chem. Int. Ed., 2000, 39, 634; CrossRef K. Wakita, N. Tokitoh, R. Okazaki, N. Takagi, and S. Nagase, J. Am. Chem. Soc., 2000, 122, 5648; CrossRef A. Shinohara, N. Takeda, T. Sasamori, T. Matsumoto, and N. Tokitoh, Organometallics, 2005, 24, 6141; CrossRef N. Tokitoh, K. Wakita, T. Matsumoto, T. Sasamori, R. Okazaki, N. Takagi, M. Kimura, and S. Nagase, J. Chin. Chem. Soc., 2008, 55, 487.
5. N. Nakata, N. Takeda, and N. Tokitoh, J. Am. Chem. Soc., 2002, 124, 6914; CrossRef N. Nakata, N. Takeda, and N. Tokitoh, J. Organomet. Chem., 2003, 672, 66; CrossRef N. Nakata, N. Takeda, and N. Tokitoh, Angew. Chem. Int. Ed., 2003, 42, 115. CrossRef
6. N. Tokitoh, K. Wakita, R. Okazaki, S. Nagase, P. v. R. Schleyer, and H. Jiao, J. Am. Chem. Soc., 1997, 119, 6951; CrossRef K. Wakita, N. Tokitoh, R. Okazaki, S. Nagase, P. v. R. Schleyer, and H. Jiao, J. Am. Chem. Soc., 1999, 121, 11336; CrossRef K. Wakita, N. Tokitoh, and R. Okazaki, Bull. Chem. Soc. Jpn., 2000, 73, 2157. CrossRef
7. N. Takeda, A. Shinohara, and N. Tokitoh, Organometallics, 2002, 21, 4024; CrossRef A. Shinohara, N. Takeda, T. Sasamori, and N. Tokitoh, Bull. Chem. Soc. Jpn., 2005, 78, 977. CrossRef
8. N. Nakata, N. Takeda, and N. Tokitoh, Organometallics, 2001, 20, 5507; CrossRef N. Nakata, N. Takeda, and N. Tokitoh, Chem. Lett., 2002, 818; CrossRef N. Nakata, N. Takeda, and N. Tokitoh, Organometallics, 2003, 22, 481. CrossRef
9. Y. Mizuhata, T. Sasamori, N. Takeda, and N. Tokitoh, J. Am. Chem. Soc., 2006, 128, 1050; CrossRef Y. Mizuhata, T. Sasamori, N. Nagahora, Y. Watanabe, Y. Furukawa, and N. Tokitoh, Dalton Trans., 2008, 4409. CrossRef
10. N. Takeda, A. Shinohara, and N. Tokitoh, Organometallics, 2002, 21, 256; CrossRef A. Shinohara, N. Takeda, and N. Tokitoh, J. Am. Chem. Soc., 2003, 125, 10804. CrossRef
11. T. Sasamori, K. Inamura, W. Hoshino, N. Nakata, Y. Mizuhata, Y. Watanabe, Y. Furukawa, and N. Tokitoh, Organometallics, 2006, 25, 3533. CrossRef
12. N. Tokitoh, A. Shinohara, T. Matsumoto, T. Sasamori, N. Takeda, and Y. Furukawa, Organometallics, 2007, 26, 4048. CrossRef
13. Y. Mizuhata, N. Takeda, T. Sasamori, and N. Tokitoh, Chem. Lett., 2005, 128, 1088. CrossRef
14. R. W. Hoffmann, J. Gehlhaus, G. Steinbach, and H. J. Lindner, Chem. Ber., 1973, 106, 1758. CrossRef
15. Y. Tobe, T. Takahashi, K. Kobiro, and K. Kakiuchi, Tetrahedron Lett., 1991, 32, 359; CrossRef Y. Tobe, A. Takemura, M. Jimbo, T. Takahashi, K. Kobiro, and K. Kakiuchi, J. Am. Chem. Soc., 1992, 114, 3479. CrossRef
16. Chemical data for 2: yellow crystals, mp 194 °C (decomp.); 1H NMR (300 MHz, CDCl3, 298 K) δ -0.19 (s, 9H), -0.14 (s, 9H), -0.12 (s, 18H), 0.02 (s, 18H), 1.32 (s, 1H), 2.28 (s, 1H), 2.52 (s, 1H), 3.00 (s, 3H), 3.08 (s, 3H), 3.69 (s, 3H), 3.73 (s, 3H), 4.07 (s, 1H), 6.33 (s, 1H), 6.39 (s, 1H), 7.21-7.55 (m, 8H); 13C NMR (75 MHz, CDCl3, 298 K) δ 0.09 (q), 0.34 (q), 0.51 (q), 0.66 (q), 0.73 (q), 0.80 (q), 27.85 (d), 28.62 (d), 30.87 (d), 38.72 (d), 49.87 (q), 51.57 (q), 51.67 (q), 52.27 (q), 112.98 (s), 122.57 (d), 125.49 (s), 125.73 (s), 127.31 (d), 127.76 (d), 127.96 (d), 128.00 (d ◊ 2), 129.74 (d), 130.74 (d), 132.42 (s), 132.73 (d), 133.49 (s), 133.85 (d), 136.72 (s), 137.03 (s), 141.58 (s), 144.63 (s), 146.88 (s), 152.19 (s), 152.26 (s), 159.54 (s), 161.97 (s), 162.40 (s), 164.74 (s). High-resolution MS (FAB) m/z Calcd for C52H80O874GeSi6 1074.3680 ([M]+). Found 1074.3701 ([M]+).
17. X-Ray crystallographic data for [2·1.5benzene] (C61H89GeO8Si6): M = 1191.45, T = 103(2) K, triclinic, P-1 (no. 2), a = 11.38400(10) Å, b = 12.7361(2) Å, c = 25.5537(4) Å, α = 94.3756(8)º, β = 95.1247(8)º, g = 115.7578(11)º, V = 3296.03(8) Å3, Z = 2, Dcalc = 1.201 g cm-3, μ = 0.622 mm-1, 2θmax = 50.0, 28126 measured reflections, 11563 independent reflections (Rint = 0.0238), 738 refined parameters, GOF = 1.104, R1 = 0.0319 and wR2 = 0.0799 [I>2σ(I)], R1 = 0.0362 and wR2 = 0.0827 [for all data], largest diff. peak and hole 0.377 and -0.558 e.Å-3. Crystallographic data (excluding structure factors) for the structure reported in this paper have been deposited with Cambridge Crystallographic Data Centre as supplementary publication no. CCDC 696804. Copies of the data can be obtained free of charge on applications to CCDC, 12 Union Road, Cambridge CB21EZ. UK (fax: (+44)1223-336-033; E-mail: deposite@ccdc.cam.ac.uk). The intensity data were collected on a Rigaku/MSC Mercury CCD diffractometer. The structure was solved by direct method (SHELXS-97) and refined by full-matrix least-squares procedures on F2 for all reflections (SHELXL-97).
18. E. Winterfeldt and G. Giesler, Angew. Chem., Int. Ed. Engl., 1966, 5, 579; CrossRef E. LeGoff and R. B. LaCount, Tetrahedron Lett., 1967, 8, 2333 CrossRef