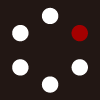
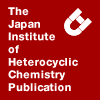
HETEROCYCLES
An International Journal for Reviews and Communications in Heterocyclic ChemistryWeb Edition ISSN: 1881-0942
Published online by The Japan Institute of Heterocyclic Chemistry
e-Journal
Full Text HTML
Received, 29th July, 2008, Accepted, 26th September, 2008, Published online, 29th September, 2008.
DOI: 10.3987/COM-08-S(F)85
■ Selective Lithiation of 4- and 5-Halophthalans
Daniel García, Francisco Foubelo,* and Miguel Yus*
Department of Organic Chemistry, Faculty of Sciences and Institute of Organic Synthesis (ISO), University of Alicante, P.O. Box 99, 03080 Alicante, Spain
Abstract
The reaction of 4- and 5-halophthalans 5 with lithium and a catalytic amount of DTBB at -78 °C leads to the formation of the corresponding functionalized organolithium intermediates 6 and 11, which by reaction with carbonyl compounds give, after hydrolysis, the expected substituted phthalans 8 and 13, respectively. When after reaction with the carbonyl compound the system is allowed to react at 0 °C, a second lithiation occur: A reductive opening of the heterocycle takes place with some regioselectivity leading to new organolithium intermediates 9 and 14/15 that by reaction with electrophiles lead, after hydrolysis, to polyfunctionalized molecules 10 and 16/17, respectively.INTRODUCTION
Functionalized organolithium compounds1 can be prepared following the same methodologies used for simple organolithium compounds. They are also accessible through the reductive ring opening of appropriate oxygen-, nitrogen- and sulfur-containing heterocycles,2 such as strained heterocycles (three and four membered-rings) and heterocycles with activated bonds. In addition, suitable precursors are compounds bearing allylic3 and benzylic4 carbon-heteroatom bonds, and cyclic aryl ethers5 and thioethers.6 Among the lithiation reagents, the use of an excess of lithium in the presence of a catalytic amount of an arene7 [naphthalene and 4,4’-di-tert-butylbiphenyl (DTBB)],8 has shown to be very effective even at low temperatures for this purpose. More recently, polymer supported naphthalene, biphenyl9 and also polyphenylene10 have been used as electron transfer reagents in these processes.11 As an example, phthalan (1; R = R’ = H) can be easily opened with the above mentioned methodology to give the expected lithiated intermediate 2a/2b (R = R’ = H)12 through a favoured reductive benzylic cleavage (Scheme 1). When the heterocyclic ring isn phthalan bears substituents (1; R = Me, n-Bu, Ph; R’ = H), the opening takes place giving the most stable intermediate 2a (R = Ph; R’ = H) or 2b (R = Me, n-Bu; R’ = H) (Scheme 1).13 In the case of non-symmetrically substituted phthalans possessing different groups attached to the aromatic ring (1; R = H; R’ = OMe, Ph), the reductive opening leading to intermediates 2 occurs with high regioselectivity and it can be explained taking into account the electron density deduced by semiempirical PM3 calculations of either the anion or the radical-anion intermediate responsible of the reductive cleavage (Scheme 1).14
Continuing with our recent studies on the selective lithiation of substituted phthalans,14 we report here the selective (chemo- and/or regioselective) lithiation of 4- and 5-halophthalans.
RESULTS AND DISCUSSION
Substituted phthalans 5 were prepared starting from the corresponding commercially available phthalic anhydrides 3 through a three-step process: first reduction with LAH in the presence of ZnCl2 to give the corresponding diol 4, followed by Swern oxidation and final cyclization of the resulting dialdehyde by treatment with Et3SiH in the presence of Me3SiOTf. The expected substituted phthalans 5 were obtained, in general, in good overall yields (Scheme 2).
The reaction of 4-chlorophthalan (5a) with an excess of lithium powder (1:10 molar ratio) and a catalytic amount of DTBB (1:0.13 molar ratio; 7.0 mol %) in THF at -78 ºC for 2 h, led to a solution of the intermediate 6, which by reaction with different carbonyl compounds as electrophiles [R1R2CO: PhCHO, (CH2)5CO] at -78 ºC gave, after hydrolysis with water at the same temperature, the corresponding alcohol 8 (Scheme 3, Table 1, entries 1 and 2).
Lithiation of 5-fluorophthalan (5c) and 5-bromophthalan (5d) under the same reaction conditions as for 4-chlorophthalan (5a) in Scheme 3 led to organolithium intermediate 11 which upon reaction with different carbonyl compounds as electrophiles [R1R2CO: t-BuCHO, Et2CO, (CH2)5CO] at -78 ºC gave, after hydrolysis with water at the same temperature, the corresponding substituted phthalan 13 (Scheme 4, Table 1, entries 3-5). In these processes, a chemoselective lithiation occurred: Carbon-halogen bonds are more reactive towards the lithiation reagent than the benzylic carbon-oxygen bond, so halogen/lithium exchange took place exclusively instead of the reductive opening of the heterocycle. The presence of a carbon-lithium bond in intermediates 6 and 11 prevents them for suffering a further reduction (reductive opening in this case) even if the process is performed at 0 ºC. Yields were considerably higher in the case of 5-halophthalans 5c,d, due probably to the higher stability of the organolithium intermediate 11 compared to 6.
From a synthetic point of view, an interesting variant of the monolithiation of the halophthalans 5 results when the second electrophile is introduced in the molecule. It happens when the alcoholates 7 [derived from 4-chlorophthalan (5a), Scheme 3] and 12 [derived from 5-bromophthalan (5d), Scheme 4] were stirred at 0 ºC for 1 h in the presence of the excess of lithium still present in the reaction medium. Under these reaction
conditions the reductive cleavage of the heterocycle took place and after addition of a new equivalent of the carbonyl compound as electrophile at -78 ºC, followed by hydrolysis with water, the triols 10 (Scheme 3) and 16/17 (Scheme 4) were obtained, respectively. The structure of these compounds was elucidated using NOESY, HSQC and HMBC experiments. The solid triol 17a [R = Ph(CH)2] gave crystals suitable for single crystal X-ray analysis and the obtained structure (Chart 1)15-18 was in total agreement with the NMR experiments. Apparently, in the case of alcoholate 7 [R1R2 = (CH2)5] a selective reductive cleavage took place leading to intermediate 9 exclusively (Scheme 3, Table 2, entry 1). However, in the case of alcoholates 12 derived from 5-bromophthalan (5d), a mixture of the two possible organolithium intermediates 14 and 15 were obtained, so leading to a mixture of triols 16 and 17 after reaction with the electrophile (Scheme 4, Table 2, entries 2 and 3). Yields were low in all cases.
The lithiation of 4-methylphthalan (5b) and 5-methylphthalan (5e) was also studied in order to rationalize the experimental results of the reductive opening of alcoholates 7 and 12, concerning the regiochemistry of the process. Lithiation of 4-methylphthalan (5b) under the same reaction conditions as previously described for other phthalan derivatives, followed by hydrolysis with water, gave a mixture of regioisomeric alcohols 20 and 21 in a 3:2 ratio. That means that the two possible reductive openings of 5b took place leading to dianionic intermediates 18 and 19 (Scheme 5). Surprisinly, 2,4-dimethylbenzyl alcohol (23a) was the only reaction product in the case of starting from 5-methylphthalan (5e) and using water as electrophile. In the case of using 3-pentanone as electrophile, diol 23b was isolated in 31% yield, 2,4-dimethylbenzyl alcohol (23a) being the major reaction product. So, under these reaction conditions reductive cleavage in 5e leading to intermediate 22 took place exclusively. Since the regiochemistry of the reductive opening of 4- and 5-methyl substituted phthalans 5b and 5e was different to that of alcoholates 7 and 12, it seems that the lithium alkoxide unit plays an important role in this process.
From the results described in this paper we can conclude that it is possible to lithiate selectively 4- and 5-halophthalans 5 using an excess of lithium in the presence of a catalytic amount of DTBB, halogen/lithium exchange taking place first. A second lithiation occurs only after the reaction of the resulting organolithium intermediates 6 and 11 with a carbonyl compound as electrophile. The new organolithium intermediates result from the reductive opening of the heterocycle that happens with some regioselectivity. The addition of a carbonyl compound as electrophile yields triols 10, 16 and 17.
EXPERIMENTAL
All reactions were performed in oven dried glassware under argon. All chemicals were commercially available (Acros, Aldrich). TLC was performed on Merck silica gel 60 F254, using aluminum plates and visualized with phosphomolybdic acid (PMA) stain. Chromatographic purification was performed by flash chromatography using Merck silica gel 60 (0.040-0.063 mm) and hexane/EtOAc as eluent. IR spectra were measured (film) with a Nicolet Impact 510 P-FT Spectrometer. Melting points were recorded on an OptiMelt (Stanford Research Systems) apparatus using open glass capillaries and reported without corrections. NMR spectra were recorded with a Bruker AC-400 using CDCl3 as the solvent and TMS as internal standard. HRMS (EI) were recorded on a Finnigan MAT 95S.
Preparation of diols 4. General procedure.
To a suspension of LiAlH4 (10 mmol, 380 mg) and ZnCl2 (3 mmol, 408 mg) in THF (20 mL) under Argon was slowly added a solution of the corresponding phthalic anhydride 3 (5 mmol) in THF (2 mL) at 0 ºC. The resulting reaction mixture was strirred at 20 ºC for 6 h. After that it was hydrolyzed with water (10 mL) at 0 ºC, acidified with 2M HCl (20 mL), extrated with EtOAc (3 × 20 mL), dried over anhydrous MgSO4 and evaporated (15 Torr). The residue was purified by column chromatography (silica gel, hexane/EtOAc) to give products 4 in almost quantitative yield. Physical and spectroscopic data as well as literature references for known compounds follow.
3-Chloro-2-(hydroxymethyl)benzyl alcohol (4a): White solid; mp 69-70 ºC (pentane/CH2Cl2); Rf = 0.29 (hexane/EtOAc: 1/1); IR ν (KBr) 3425-3455 (OH), 3057, 2971, 2892 cm-1; 1H NMR (400 MHz, CDCl3) δ 4.61 (2H, br s, 2×OH), 4.77 (4H, s, 2×CH2OH), 7.14-7.16 (2H, m, ArH), 7.26-7.27 (1H, m, ArH); 13C NMR (100 MHz, CDCl3) δ 59.1, 63.9 (CH2), 128.3, 129.4, 129.5, 135.0, 136.1, 141.6 (ArC); LRMS (EI) m/z 156 (M+-H2O, 32%), 155 (41), 154 (98), 153 (100), 127 (12), 125 (34), 91 (44), 90 (10), 89 (27), 77 (28), 63 (10), 51 (11).
2-(Hydroxymethyl)-3-methylbenzyl alcohol (4b): Colorless oil; Rf = 0.21 (hexane/EtOAc: 1/1); IR ν (film) 3380-3295 (OH), 3069, 2965, 2897 cm-1; 1H NMR (400 MHz, CDCl3) δ 2.33 (3H, s, CH3), 4.11 (2H, br s, 2×OH), 4.48 (2H, s, CH2OH), 4.53 (2H, s, CH2OH), 7.04-7.11 (3H, m, ArH); 13C NMR (100 MHz, CDCl3) δ 19.3 (CH3), 58.4, 65.8 (CH2), 127.4, 128.0, 130.5, 137.2, 137.5, 139.8 (ArC); LRMS (EI) m/z 152 (M+, 1%), 135 (10), 134 (100), 133 (82), 132 (16), 119 (12), 106 (37), 105 (88), 103 (29), 91 (73), 79 (24), 78 (18), 77 (43), 65 (11), 51 (18).
4-Fluoro-2-(hydroxymethyl)benzyl alcohol (4c): Colorless oil; Rf = 0.20 (hexane/EtOAC: 1/1); IR ν (film) 3405-3335 (OH), 2947, 2887 cm-1; 1H NMR (400 MHz, CDCl3) δ 4.43 (2H, s, CH2OH), 4.46 (2H, s, CH2OH), 4.70 (2H, br s, 2×OH), 6.86-6.97 (2H, m, ArH), 7.13-7.18 (1H, m, ArH); 13C NMR (100 MHz, CDCl3) δ 62.2, 62.3 (CH2), 114.2, 114.4, 115.4, 115.7, 130.1, 134.3, 141.5, 141.6, 160.7, 163.9 (ArC); LRMS (EI) m/z 138 (M+-H2O, 100%), 137 (98), 110 (13), 109 (81), 107 (11), 97 (15), 95 (10), 83 (16), 77 (11).
4-Bromo-2-(hydroxymethyl)benzyl alcohol (4d):19 White solid; mp 69-70 ºC (pentane/CH2Cl2); Rf = 0.20 (hexane/EtOAc: 1/1); IR ν (KBr) 3320-3235 (OH), 2941, 2882 cm-1; 1H NMR (400 MHz, CDCl3) δ 4.22 (2H, br s, 2×OH), 4.62 (2H, s, CH2OH), 4.66 (2H, s, CH2OH), 7.29-7.32 (1H, m, ArH), 7.39-7.43 (1H, m, ArH), 7.59 (1H, s, ArH); 13C NMR (100 MHz, CDCl3) δ 61.9, 62.1 (CH2), 122.3, 130.1, 131.2, 131.3, 139.1, 142.8 (ArC); LRMS (EI) m/z 218 (M+, 2%), 216 (2), 200 (94), 199 (89), 198 (100), 197 (82), 171 (28), 169 (29), 92 (13), 91 (69), 90 (35), 89 (45), 79 (10), 78 (24), 77 (41), 65 (17), 63 (24), 51 (22), 50 (12).
2-(Hydroxymethyl)-4-methylbenzyl alcohol (4e):20 Colorless oil; Rf = 0.25 (hexane/EtOAc: 1/1); IR ν (film) 3355-3280 (OH), 3014, 2917, 2880 cm-1; 1H NMR (400 MHz, CDCl3) δ 2.29 (3H, s, CH3), 4.16 (2H, br s, 2×OH), 4.48 (4H, s, 2×CH2OH), 7.03-7.06 (2H, m, ArH), 7.12 (1H, d, J = 7.7 Hz, ArH); 13C NMR (100 MHz, CDCl3) δ 21.0 (CH3), 63.2, 63.5 (CH2), 128.8, 129.6, 130.3, 136.2, 138.1, 139.1 (ArC); LRMS (EI) m/z 152 (M+, 1%), 135 (10), 134 (100), 133 (97), 106 (11), 105 (49), 103 (13), 93 (11), 91 (53), 79 (17), 78 (11), 77 (37), 65 (11), 51 (11).
Preparation of phthalans 5. General procedure.
To a solution of oxalyl chloride (11.5 mmol, 1.450 g, 1.0 mL) in CH2Cl2 (45 mL) was added dimethylsulfoxide (25 mmol, 1.750 g, 1.8 mL) at -65 ºC. After 5 min at this temperature, a solution of the corresponding diol 4 (5 mmol) in CH2Cl2 (45 mL) was added and stirring continued for 15 min prior to the addition Et3N (52 mmol, 5.252 g, 7.2 mL). After that, the cold bath was removed and stirring was manteined for 2 h at 20 ºC. The reaction mixture was hydrolyzed with 2M HCl (50 mL) and extrated with CH2Cl2 (3 × 40 mL). The organic layers were washed with water (3 × 50 mL), dried over anhydrous MgSO4 and evaporated (15 Torr). The resulting dialdehyde was used without purification in the next step of the reaction. To a solution of the corresponding dialdehyde (4.50 mmol) in dry CH2Cl2 (15 mL) was successively added Et3SiH (20 mmol, 2.32 g, 2.58 mL) and Me3SiOTf (0.134 mml, 30 mg, 0.025 mL) at 0 ºC. The reaction mixture was stirred at the same temperature for 4 h and for 2 h at 20 ºC. After that the solvent was evaporated (15 Torr) and the resulting residue was purified by column chromatography (silica gel, hexane/EtOAc) to give products 5. Overall yields are given in Scheme 2. Physical, and spectroscopic data as well as literature references for known compounds follow.
4-Chlorophthalan (5a): Yellow oil; Rf = 0.41 (hexane/EtOAc: 10/1); IR ν (film) 3155, 2917, 2855, 1456 cm-1; 1H NMR (400 MHz, CDCl3) δ 5.12 (2H, s, CH2O), 5.16 (2H, s, CH2O), 7.10 (1H, t, J = 4.1 Hz, ArH), 7.21 (2H, d, J = 3.85 Hz, ArH); 13C NMR (100 MHz, CDCl3) δ 73.3, 74.5 (CH2), 119.3, 127.4, 128.0, 129.2, 137.8, 141.3 (ArC); LRMS (EI) m/z 156 (M+, 17%), 155 (20), 154 (52), 153 (48), 127 (26), 126 (28), 125 (75), 99 (10), 92 (10), 91 (100), 90 (18), 89 (44), 63 (24), 62 (11); HRMS (EI) calcd for C8H7ClO 154.0185, found 154.0200.
4-Methylphthalan (5b):21 Yellow oil; Rf = 0.36 (hexane/EtOAc: 10/1); IR ν (film) 3027, 2916, 2855 cm-1; 1H NMR (400 MHz, CDCl3) δ 2.30 (3H, s, CH3), 5.07 (2H, s, CH2O), 5.12 (2H, s, CH2O), 7.02-7.05 (2H, m, ArH), 7.10-7.19 (1H, m, ArH); 13C NMR (100 MHz, CDCl3) δ 18.8 (CH3), 73.1, 74.1 (CH2), 118.2, 127.6, 128.0, 130.3, 138.1, 138.7 (ArC); LRMS (EI) m/z 134 (M+, 63%), 133 (43), 119 (16), 106 (56), 105 (100), 103 (29), 91 (71), 79 (25), 78 (15), 77 (38), 63 (11), 51 (20).
5-Fluorophthalan (5c): Yellowish oil; Rf = 0.32 (hexane/EtOAc: 10/1); IR ν (film) 3024, 1262 cm-1; 1H NMR (400 MHz, CDCl3) δ 5.06 (4H, s, CH2O), 6.90-6.97 (2H, m, ArH), 7.13-7.17 (1H, m, ArH); 13C NMR (100 MHz, CDCl3) δ 73.1, 73.4 (CH2), 108.1, 108.5, 114.3, 114.6, 122.1, 122.2, 134.5, 141.4, 141.5, 161.1, 164.3 (ArC); LRMS (EI) m/z 138 (M+, 43%), 137 (49), 110 (57), 109 (100), 107 (19), 83 (27), 57 (10).
5-Bromophthalan (5d): White solid; mp 41-42 ºC (pentane/CH2Cl2); Rf = 0.30 (hexane/EtOAc: 10/1); IR ν (KBr) 3074, 1051 cm-1; 1H NMR (400 MHz, CDCl3) δ 5.09 (2H, s, CH2O), 5.20 (2H, s, CH2O), 7.15 (2H, d, J = 6.3 Hz, ArH), 7.35 (1H, dd, J = 6.3, 2.3 Hz, ArH); 13C NMR (100 MHz, CDCl3) δ 74.7 (CH2), 115.9, 119.7, 129.2, 130.2, 139.8, 141.0 (ArC); LRMS (EI) m/z 200 (M+, 35%), 199 (31), 198 (36), 197 (29), 172 (14), 171 (37), 170 (15), 169 (38), 118 (10), 91 (100), 89 (42), 88 (41), 63 (24), 62 (11).
5-Methylphthalan (5e):22 Colorless oil; Rf = 0.39 (hexane/EtOAc: 10/1); IR ν (film) 3014, 2953, 2910, 2850 cm-1; 1H NMR (400 MHz, CDCl3) δ 2.31 (3H, s, CH3), 5.00 (4H, s, 2×CH2O), 6.96 (1H, s, ArH), 6.99-7.06 (2H, m, ArH); 13C NMR (100 MHz, CDCl3) δ 21.0 (CH3), 73.2 (CH2), 120.4, 121.3, 127.9, 136.0, 136.7, 139.2 (ArC); LRMS (EI) m/z 134 (M+, 65%), 133 (52), 119 (10), 106 (51), 105 (100), 103 (25), 91 (52), 79 (21), 78 (11), 77 (31), 63 (10), 51 (14).
Monolithiation of phthalans 5. Preparation of compounds 8, 13, 20, 21 and 23. General procedure.
To a blue suspension of lithium powder (72 mg, 10.4 mmol) and a catalytic amount of DTBB (34 mg, 0.13 mmol) in dry THF (3 mL) under argon was added dropwise a solution of the corresponding phthalan 5 (1 mmol) in THF (0.5 mL) at -78 ºC, and the resulting mixture was stirred for 2 h at the same temperature. Then the corresponding carbonyl compound was added dropwise (1.1 mmol) at -78 ºC and, after 20 min the reaction mixture was hydrolyzed with water (4 mL), extracted with EtOAc (3 × 15 mL), dried over anhydrous MgSO4 and evaporated (15 Torr). The residue was purified by column chromatography (silica gel, hexane/ethyl acetate) to yield pure products 8, 13, 20, 21 and 23. Yields are given in Table 1 and Scheme 4. Physical, analytical and spectroscopic data as well as literature references for known compounds follow.
4-(1-Hydroxy1-phenylmethyl)phthalan (8a): Colorless oil; Rf = 0.11 (hexane/EtOAc: 5/1); IR ν (film) 3420-3355 (OH), 3069, 3032, 2928, 2855 cm-1; 1H NMR (400 MHz, CDCl3) δ 2.60 (1H, br s, OH), 4.91 (1H, d, J = 12.9 Hz, CHHO), 4.96 (1H, d, J = 12.9 Hz, CHHO), 5.02 (2H, s, CH2O), 7.13 (1H, d, J = 7.3 Hz, ArH), 5.74 (1H, s, CHOH), 7.24-7.33 (7H, m, ArH); 13C NMR (100 MHz, CDCl3) δ 72.8, 73.3 (CH2), 75.0 (CH), 120.2, 125.1, 126.8, 127.8, 128.0, 128.7, 136.8, 137.6, 139.9, 142.5 (ArC); LRMS (EI) m/z 226 (M+, 2%), 209 (17), 208 (100), 207 (72), 180 (19), 179 (23), 165 (34), 152 (11), 146 (17), 132 (11), 119 (36), 104 (19), 91 (38), 89 (18), 79 (10), 77 (30), 65 (12), 63 (12), 51 (10); HRMS (EI) calcd for C15H12O [M+-H2O] 208.0883, found 208.0841.
4-(1-Hydroxycyclohexyl)phthalan (8b): Colorless oil; Rf = 0.14 (hexane/EtOAc: 5/1); IR ν (film) 3430-3365 (OH), 2918, 2849, 1037 cm-1; 1H NMR (400 MHz, CDCl3) δ 1.68-1.80 (10H, m, 5×CH2), 2.45 (1H, br s, OH), 5.06 (2H, s, CH2O), 5.35 (2H, s, CH2O), 7.12 (1H, d, J = 6.1 Hz, ArH), 7.20-7.25 (2H, m, ArH); 13C NMR (100 MHz, CDCl3) δ 20.3, 24.3, 25.6, 30.8, 35.7, 72.8, 74.5 (CH2), 75.8 (CH), 119.5, 123.8, 127.6, 127.7, 130.5, 140.6 (ArC); LRMS (EI) m/z 218 (M+, 7%), 201 (15), 200 (100), 199 (14), 185 (10), 171 (18), 167 (10), 159 (30), 157 (21), 147 (10), 145 (29), 144 (10), 143 (12), 141 (13), 119 (13), 115 (28), 104 (10), 91 (21); HRMS (EI) calcd for C14H16O [M+-H2O] 200.1201, found 200.1233.
5-(1-Hydroxycyclohexyl)phthalan (13a): Yellow oil; Rf = 0.16 (hexane/EtOAc: 5/1); IR ν (film) 3475-3395(OH), 3069, 2933, 2855, 1035 cm-1; 1H NMR (400 MHz, CDCl3) δ 1.70-1.85 (10H, m, 5×CH2), 2.63 (1H, br s, OH), 5.10 (4H, br s, CH2O), 7.21 (1H, d, J = 8.6 Hz, ArH), 7.40-7.42 (2H, m, ArH); 13C NMR (100 MHz, CDCl3) δ 22.3, 25.6, 39.2, 73.3, 73.5 (CH2), 73.7 (CH), 117.3, 120.8, 124.0, 137.6, 139.3, 149.2 (ArC); LRMS (EI) m/z 218 (M+, 28%), 188 (10), 175 (22), 162 (22), 147 (19), 146 (15), 145 (100), 133 (11), 119 (10), 105 (10), 104 (14), 91 (20), 55 (15); HRMS (EI) calcd for C14H18O2 218.1307, found 218.1305.
5-(1-Hydroxy-2,2-dimethylpropyl)phthalan (13b):23 Colorless oil; Rf = 0.34 (hexane/EtOAc: 2/1); IR ν (film) 3460-3385 (OH), 3020 (ArH), 2953, 2892, 2867 cm-1; 1H NMR (400 MHz, CDCl3) δ 0.92 [9H, s, C(CH3)3], 2.55 (1H, br s, OH), 4.40 (1H, s, CHOH), 5.08 (4H, br s, CH2O), 7.13-7.26 (3H, m, ArH); 13C NMR (100 MHz, CDCl3) δ 26.0 (CH3), 35.1 (C), 73.5, 73.6 (CH2), 82.4 (CH), 120.0, 120.1, 127.1, 138.1, 138.6, 141.8 (ArC); LRMS (EI) m/z 206 (M+, 6%), 150 (15), 149 (100), 93 (18), 91 (34); HRMS (EI) calcd for C13H18O2 206.1307, found 206.1292.
5-(1-Ethyl-1-hydroxypropyl)phthalan (13c): Colorless oil; Rf = 0.19 (hexane/EtOAc: 5/1); IR ν (film) 3465-3390 (OH), 2967. 2940, 2855 cm-1; 1H NMR (400 MHz, CDCl3) δ 0.77 (6H, t, J = 7.4 Hz, 2×CH3), 1.79-1.91 (4H, m, 2×CH2CH3), 5.10 (4H, br s, CH2O), 7.19 (1H, d, J = 7.95 Hz, ArH), 7.25-7.29 (2H, m, ArH); 13C NMR (100 MHz, CDCl3) δ 8.0 (CH3), 35.3, 73.5, 73.7 (CH2), 77.5 (C), 118.2, 120.5, 124.8, 137.1, 139.1, 145.5 (ArC); LRMS (EI) m/z 206 (M+, 1%), 178 (13), 177 (100), 175, 147 (11), 119 (10), 91 (11), 57 (50); HRMS (EI) calcd for C13H18O2 206.1307, found 206.1300.
2,3-Dimethylbenzyl alcohol (20):24 1H NMR (400 MHz, CDCl3) δ 1.75 (1H, br s, OH), 2.24 (3H, s, CH3), 2.28 (3H, s, CH3), 4.66 (2H, s, CH2OH), 7.07-7.18 (3H, m, ArH); 13C NMR (100 MHz, CDCl3) δ 20.4 (CH3), 64.2 (CH2OH), 125.6, 125.8, 129.7, 135.0, 137.5, 138.1 (ArC); LRMS (EI) m/z 136 (M+, 35%), 134 (37), 133 (36), 121 (21), 119 (21), 118 (100), 117 (47), 115 (10), 107 (14), 105 (52), 103 (23), 93 (30), 91 (73), 79 (19), 78 (12), 77 (44), 65 (13), 51 (15).
2,6-Dimethylbenzyl alcohol (21):25 1H NMR (400 MHz, CDCl3) δ 1.75 (1H, br s, OH), 2.41 (6H, s, 2×CH3), 4.70 (2H, s, CH2OH), 7.01-7.19 (3H, m, ArH); 13C NMR (100 MHz, CDCl3) δ 19.5 (CH3), 59.4 (CH2OH), 128.1, 128.5, 136.6, 137.3 (ArC); LRMS (EI) m/z 136 (M+, 35%), 134 (37), 133 (36), 121 (21), 119 (21), 118 (100), 117 (47), 115 (10), 107 (14), 105 (52), 103 (23), 93 (30), 91 (74), 79 (19), 78 (12), 77 (45), 65 (13), 51 (16).
2,4-Dimethylbenzyl alcohol (23a): Yellow oil; Rf = 0.27 (hexane/EtOAc: 6/1); IR ν (film) 3395-3320 (OH), 3006, 2959, 2917, 2849 cm-1; 1H NMR (400 MHz, CDCl3) δ 1.85 (1H, br s, OH), 2.30 (6H, br s, 2×CH3), 4.60 (2H, s, CH2OH), 6.99 (2H, br s, ArH), 7.18-7.23 (1H, m, ArH); 13C NMR (100 MHz, CDCl3) δ 18.7, 21.1 (CH3), 63.4 (CH2), 126.7, 127.9, 131.3, 135.8, 136.2, 137.6 (ArC); LRMS (EI) m/z 136 (M+, 52%), 135 (10), 134 (29), 133 (41), 121 (47), 119 (24), 118 (100), 117 (54), 115 (19), 107 (22), 105 (45), 103 (25), 93 (33), 91 (79), 79 (17), 78 (11), 77 (39), 65 (14), 51 (15).
3-[(2-Hydroxymethyl-5-methyl)phenyl]pentan-3-ol (23b): Yellow oil; Rf = 0.32 (hexane/EtOAc: 2/1); IR ν (film) 3340-3285 (OH), 2963, 2929, 2879 cm-1; 1H NMR (400 MHz, CDCl3) δ 0.94 (6H, t, J = 7.4 Hz, CH3), 1.52-1.60 (4H, m, CH2CH3), 2.33 (3H, s, ArCH3), 2.82 (2H, s, ArCH2), 4.53 (2H, s, CH2OH), 6.94 (1H, s, ArH), 7.02 (1H, d, J = 7.55 Hz, ArH), 7.21 (1H, d, J = 7.55 Hz, ArH); 13C NMR (100 MHz, CDCl3) δ 8.2, 21.3 (CH3), 30.9, 40.6, 63.1 (CH2), 74.3 (C), 127.7, 130.8, 132.8, 136.1, 137.3, 137.7 (ArC); LRMS (EI) m/z 204 [(M+-H2O) 2%], 186 (20), 184 (21), 175 (61), 171 (12), 169 (33), 157 (55), 153 (11), 143 (20), 142 (24), 141 (20), 128 (15), 119 (29), 118 (100), 117 (22), 115 (18), 91 (14), 57 (23); HRMS (EI) calcd for C14H20O [M+-H2O] 204.1514, found 204.1494.
Double lithiation of phthalans 5. Preparation of compounds 10, 16 and 17. General procedure.
To a blue suspension of lithium powder (72 mg, 10.4 mmol) and a catalytic amount of DTBB (34 mg, 0.13 mmol) in dry THF (3 mL) under argon was added dropwise a solution of the corresponding phthalan 5 (1 mmol) in THF (0.5 mL) at -78 ºC, and the resulting mixture was stirred for 2 h at the same temperature. Then, the corresponding carbonyl compound was added dropwise (1.1 mmol) at -78 ºC and stirring was continued for 20 min. After that, the reaction mixture was allowed to rise to 0 ºC and stirring was continued for 1 h at this temperature. Then, the reaction mixture was cooled down to -78 ºC and the carbonyl compound (1.1 mmol) was added dropwise. Finally it was hydrolyzed with water (4 mL), extracted with EtOAc (3 × 15 mL), dried over anhydrous MgSO4 and evaporated (15 Torr). The residue was purified by column chromatography (silica gel, hexane/ethyl acetate) to yield pure products 10, 16 and 17. Yields are given in Table 2. Physical, analytical and spectroscopic data follow.
1-[2-(1-Hydroxycyclohexyl)-6-(hydroxymethyl)benzyl]cyclohexanol (10): White solid; mp 206-207 ºC (pentane/CH2Cl2); Rf = 0.21 (hexane/EtOAc: 2/1); IR ν (KBr) 3420-3350 (OH), 3063, 3020, 2928, 2853, 1034 cm-1; 1H NMR (400 MHz, CDCl3) δ 1.26-1.37 (4H, m, 2×CH2), 1.38-1.93 (14H, m, 7×CH2), 2.01-2.05 (2H, m, CH2), 2.61 (2H, s, ArCH2), 4.72 (2H, s, CH2OH), 7.18 (1H, t, J = 7.7 Hz, ArH), 7.32 (1H, d, J = 7.0 Hz, ArH), 7.72 (1H, d, J = 8.0 Hz, ArH); 13C NMR (100 MHz, CDCl3) δ 16.7, 22.3, 25.6, 37.9, 64.3 (CH2), 74.4, 77.4 (CH), 125.2, 125.6, 126.7, 135.4, 140.9, 146.5 (ArC); LRMS (EI) m/z 220 {M+-[(CH2)5COH], 9%}, 202 (24), 159 (12), 150 (13), 149 (100), 147 (46), 143 (11), 129 (15), 128 (13), 114 (13), 91 (14), 77 (11), 55 (14); HRMS (EI) calcd for C14H20O2 [M+-(CH2)5COH] 220.1463, found 220.1457.
3-[3-(2-Ethyl-2-hydroxybutyl)-4-(hydroxymethyl)phenyl]pentan-3-ol (16a): White solid; mp 90-91 ºC (pentane/CH2Cl2); Rf = 0.37 (hexane/EtOAc: 1/1); IR ν (KBr) 3375-3300 (OH), 2964, 2937, 2878 cm-1; 1H NMR (400 MHz, CDCl3) δ 0.76 (6H, t, J = 7.4 Hz, 2×CH2CH3), 0.94 (6H, t, J = 7.5 Hz, 2×CH2CH3), 1.43-1.62 (4H, m, 2×CH2CH3), 1.73-1.89 (4H, m, 2×CH2CH3), 2.88 (2H, s, ArCH2), 4.57 (2H, s, CH2OH), 7.15 (1H, d, J = 1.7 Hz, ArH), 7.21 (1H, dd, J = 8.4, 1.9 Hz, ArH), 7.30 (1H, d, J = 1.9 Hz, ArH); 13C NMR (100 MHz, CDCl3) δ 7.9, 8.2 (CH3), 31.1, 35.0, 41.1, 63.1 (CH2), 75.0, 77.3 (C), 124.2, 129.5, 130.5, 135.7, 138.5, 145.3 (ArC); LRMS (EI) m/z 247 [(M+-Et-H2O), 20%], 191 (15), 190 (85), 179 (12), 161 (57), 159 (12), 134 (15), 133 (34), 105 (10), 91 (11), 87 (16), 57 (100); HRMS (EI) calcd for C16H23O2 [M+-Et-H2O] 247.1693, found 247.1702.
3-[4-(2-Ethyl-2-hydroxybutyl)-3-(hydroxymethyl)phenyl]pentan-3-ol (17a): White solid; mp 119-120 ºC (pentane/CH2Cl2); Rf = 0.27 (hexane/EtOAc: 1/1); IR ν (KBr) 3390-3310 (OH), 3027, 2966, 2938, 2879 cm-1; 1H NMR (400 MHz, CDCl3) δ 0.76 (6H, t, J = 7.4 Hz, 2×CH3), 0.95 (6H, t, J = 7.4 Hz, 2×CH3), 1.50-1.64 (4H, m, 2×CH2CH3), 1.79-1.86 (4H, m, 2×CH2CH3), 2.86 (2H, s, ArCH2), 4.60 (2H, s, CH2OH), 7.11 (1H, d, J = 8.05 Hz, ArH), 7.24-7.26 (1H, m, ArH), 7.35-7.37 (1H, m, ArH); 13C NMR (100 MHz, CDCl3) δ 8.1, 8.2 (CH3), 31.1, 34.8, 40.3, 63.9 (CH2), 75.0, 77.4 (C), 125.0, 128.0, 131.9, 134.0, 140.2, 144.8 (ArC); LRMS (EI) m/z 247 [(M+-Et-H2O), 29%], 191 (15), 190 (100), 179 (24), 172 (11), 162 (12), 161 (84), 148 (10), 134 (18), 133 (41), 105 (14), 91 (13), 87 (22), 57 (98); HRMS (EI) calcd for C16H23O2 [M+-Et-H2O] 247.1693, found 247.1689.
1-[5-(1-Hydroxycyclohexyl)-2-(hydroxymethyl)benzyl]cyclohexanol (16b): White solid; mp 166-167 ºC (pentane/CH2Cl2); Rf = 0.27 (hexane/EtOAc: 1/1); IR ν (KBr) 3390-3315 (OH), 2931, 2854, 1022 cm-1; 1H NMR (400 MHz, CDCl3) δ 1.31-1.57 (10H, m, 5×CH2), 1.71-1.85 (10H, m, 5×CH2), 2.88 (2H, s, ArCH2), 4.64 (2H, s, CH2OH), 7.29-7.34 (3H, m, ArH); 13C NMR (100 MHz, CDCl3) δ 23.2, 26.7, 27.0, 38.7, 39.7, 63.5 (CH2), 72.4, 73.8 (CO), 123.9, 129.8, 130.2, 136.9, 139.4, 150.0 (ArC); LRMS (EI) m/z 282 [(M+-2H2O), 22%], 264 (10), 202 (19), 185 (18), 184 (100), 155 (11), 141 (20), 129 (10), 128 (11); HRMS (EI) calcd for C20H26O [M+-2H2O] 282.1984, found 282.1951.
ACKNOWLEDGEMENTS
This work was generously supported by the Spanish Ministerio de Educación y Ciencia (MEC; grants no. Consolider Ingenio 2010-CSD2007-00006 and CTQ-2007-65218). D. G. thanks the University of Alicante for a predoctoral fellowship. We also thank MEDALCHEMY S.L. for a gift of chemicals.
This paper is dedicated to Professor Emeritus Keiichiro Fukumoto on the occasion of his 75th birthday.
References
1. For reviews, see: (a) C. Nájera and M. Yus, Trends Org. Chem., 1991, 2, 155; (b) C. Nájera and M. Yus, Org. Prep. Proced. Int., 1995, 27, 383; (c) C. Nájera and M. Yus, Recent Res. Dev. Org. Chem., 1997, 1, 67; (d) C. Nájera and M. Yus, Curr. Org. Chem., 2003, 7, 867; CrossRef (e) C. Nájera, J. M. Sansano, and M. Yus, Tetrahedron, 2003, 59, 9255; CrossRef (f) R. Chinchilla, C. Nájera, and M. Yus, Chem. Rev., 2004, 104, 2667; CrossRef (g) R. Chinchilla, C. Nájera, and M. Yus, Tetrahedron, 2005, 61, 3139; CrossRef (h) M. Yus and F. Foubelo, Functionalized Organometallics, P. Knochel (Ed.), Wiley-VCH, Weinheim, 2005, Vol. 1, Chapter 2; (i) C. Nájera and M. Yus, The Chemistry of Organolithium Compounds, Z. Rappoport and I. Marek (Eds.), J. Wiley & Sons, Chichester, 2006, Vol. 2, Chapter 3; (j) R. Chinchilla, C. NÁjera, and M. Yus, Arkivoc, 2007, x, 152.
2. For reviews, see: (a) M. Yus and F. Foubelo, Rev. Heteroatom Chem., 1997, 17, 73; (b) M. Yus and F. Foubelo, Targets in Heterocyclic Systems, 2002, 6, 136; (c) M. Yus, Pure Appl. Chem. 2003, 75, 1453; CrossRef (d) M. Yus and F. Foubelo, Adv. Heterocycl. Chem., 2006, 91, 135. CrossRef
3. (a) S. F. Sabes, R. A. Urbanek, and C. J. Forsyth, J. Am. Chem. Soc., 1998, 120, 2534; CrossRef (b) F. Alonso, E. Lorenzo, and M. Yus, Tetrahedron Lett. 1997, 38, 2187; CrossRef (c) F. Alonso, E. Lorenzo, and M. Yus, Tetrahedron Lett., 1998, 39, 3303; CrossRef (d) E. Lorenzo, F. Alonso, and M. Yus, Tetrahedron, 2000, 56, 1745; CrossRef (e) E. Lorenzo, F. Alonso, and M. Yus, Tetrahedron Lett., 2000, 41, 1661; CrossRef (f) F. Alonso, L. R. Falvello, P. E. Fanwick, E. Lorenzo, and M. Yus, Synthesis, 2000, 949; CrossRef (g) F. Alonso, J. Meléndez, and M. Yus, Helv. Chim. Acta, 2002, 85, 3262; CrossRef (h) M. Yus, B. Maciá, and C. Gómez, Tetrahedron, 2003, 59, 5183; CrossRef (i) F. Alonso, E. Lorenzo, J. Meléndez, and M. Yus, Tetrahedron, 2003, 59, 5199. CrossRef
4. (a) U. Azzena, S. Demartis, M. G. Fiori, G. Melloni, and L. Pisano, Tetrahedron Lett., 1995, 36, 5641; (b) J. Almena, F. Foubelo, and M. Yus, J. Org. Chem., 1996, 61, 1859.; CrossRef (c) J. Almena, F. Foubelo, and M. Yus, Tetrahedron, 1996, 52, 8545; CrossRef (d) For a review, see: U. Azzena, Trends Org. Chem., 1997, 6, 55; (e) J. Almena, F. Foubelo, and M. Yus, Tetrahedron, 1997, 53, 5563; CrossRef (f) U. Azzena, S. Carta, G. Melloni, and A. Sechi, Tetrahedron, 1997, 53, 16205. CrossRef
5. (a) A. Maercker, Angew. Chem. Int. Ed. Engl., 1987, 26, 972; CrossRef (b) M. C. R. L. R. Lazana, M. L. T. M. B. Franco, and B. J. Herold, J. Am. Chem. Soc., 1989, 111, 8640; CrossRef (c) F. Casado, L. Pisano, M. Farriol, I. Gallardo, J. Marquet, and G. Melloni, J. Org. Chem., 2000, 65, 322; CrossRef (d) U. Azzena, T. Denurra, G. Melloni, and A. M. Piroddi, J. Org. Chem., 1990, 55, 5386; CrossRef (e) U. Azzena, T. Denurra, G. Melloni, E. Fenude, and G. Rassu, J. Org. Chem., 1992, 57, 1444; CrossRef (f) A. Bachki, F. Foubelo, and M. Yus, Tetrahedron Lett., 1998, 39, 7759; CrossRef (g) F. Foubelo and M. Yus, Tetrahedron Lett., 1999, 40, 743; CrossRef (h) F. Foubelo, S. A. Saleh, and M. Yus, J. Org. Chem., 2000, 65, 3478; CrossRef (i) J. V. FerrÁndez, F. Foubelo, and M. Yus, Eur. J. Org. Chem., 2001, 3223; (j) M. Yus, F. Foubelo, J. V. FerrÁndez, and A. Bachki, Tetrahedron, 2002, 58, 4907. CrossRef
6. (a) C. G. Screttas and M. Micha-Screttas, J. Org. Chem., 1978, 43, 1064; CrossRef (b) C. G. Screttas, and M. Micha-Screttas, J. Org. Chem., 1979, 44, 713; CrossRef (c) T. Cohen and M. Bhupathy, Acc. Chem. Res., 1989, 22, 152; CrossRef (d) F. Foubelo, A. Gutiérrez, and M. Yus, Tetrahedron Lett., 1997, 38, 4837; CrossRef (e) F. Foubelo, A. Gutiérrez, and M. Yus, Synthesis, 1999, 503; CrossRef (f) F. Foubelo, A. Gutiérrez, and M. Yus, Tetrahedron Lett., 1999, 40, 8173; CrossRef (g) F. Foubelo, A. Gutiérrez, and M. Yus, Tetrahedron Lett., 1999, 40, 8177; CrossRef (h) F. Foubelo and M. Yus, Tetrahedron Lett., 2000, 41, 5335; CrossRef (i) A. Gutiérrez, F. Foubelo, and M. Yus, Tetrahedron, 2001, 57, 4411; CrossRef (j) M. Yus, F. Foubelo, and J. V. Ferrández, Chem. Lett., 2002, 726; CrossRef (k) M. Yus, F. Foubelo, and J. V. Ferrández, Tetrahedron Lett., 2002, 43, 7205; CrossRef (l) M. Yus, F. Foubelo, and J. V. Ferrández, Tetrahedron, 2003, 59, 2083. CrossRef
7. For the first account on this reaction, see: M. Yus and D. J. Ramón, J. Chem. Soc., Chem. Commun., 1991, 398. CrossRef
8. For reviews, see: (a) M. Yus, Chem. Soc. Rev., 1996, 25, 155; CrossRef (b) D. J. Ramón and M . Yus, Eur. J. Org. Chem., 2000, 225; (c) M. Yus, Synlett, 2001, 1197; CrossRef (d) M. Yus, The Chemistry of Organolithium Compounds, Z. Rapppoport and I. Marek (Eds.), J. Wiley Sons, Chichester, 2004, Vol. 1, Part 1, Chapter 11.
9. (a) C. Gómez, S. Ruiz, and M. Yus, Tetrahedron Lett., 1998, 39, 1397; CrossRef (b) C. Gómez, S. Ruiz, and M. Yus, Tetrahedron, 1999, 55, 7017. CrossRef
10. M. Yus, C. Gómez, and P. Candela, Tetrahedron, 2002, 58, 6207. CrossRef
11. For studies on the mechanism of this reaction, see: (a) M. Yus, R. P. Herrera, and A. Guijarro, Tetrahedron Lett., 2001, 42, 3455; CrossRef (b) M. Yus, R. P. Herrera, and A. Guijarro, Chem. Eur. J., 2002, 8, 2574; CrossRef (c) R. P. Herrera, A. Guijarro, and M. Yus, Tetrahedron Lett., 2003, 44, 1309; CrossRef (d) R. P. Herrera, A. Guijarro, and M. Yus, Tetrahedron Lett., 2003, 44, 1313. CrossRef
12. J. Almena, F. Foubelo, and M. Yus, Tetrahedron, 1995, 51, 3351. CrossRef
13. U. Azzena, S. Demartis, and G. Melloni, J. Org. Chem., 1996, 61, 4913. CrossRef
14. (a) F. Foubelo, D. García, B. Moreno, and M. Yus, Tetrahedron Lett., 2007, 48, 3379; CrossRef (b) D. García, F. Foubelo, and M. Yus, Tetrahedron, 2008, 64, 4275. CrossRef
15. Crystal data (excluding structure factors) deposited at the Cambridge Crystallographic Data Centre as supplementary publication number CCDC 696182: C18H30O3, M = 294.42; orthorhombic, a = 15.3894(8) Å, b = 10.1191(5) Å, c = 22.8872(12) Å; V = 3564.2(3) Å3; space group Pca2(1); Z = 8; Dc = 1.097 Mg m-3; λ = 0.71073 Å; μ = 0.073 mm-1; F(000) = 1296; T = 23±1 °C. Data collection was performed on a Bruker Smart CCD diffractometer, based on three ω-scan runs (starting = -34°) at values φ =0°, 120°, 240° with the detector at 2θ =-32°. For each of these runs, 606 frames were collected at 0.3° intervals and 10 s per frame. An additional run at φ =0° of 100 frames was collected to improve redundancy. The diffraction frames were integrated using the program SAINT16 and the integrated intensities were corrected for Lorentz-polarization effects with SADABS.17 The structure was solved by direct methods18 and refined to all 3247 unique Fo2 by full matrix least squares.18 All the hydrogen atoms were placed at idealized positions and refined as rigid atoms. Final wR2=0.1194 for all data and 393 parameters; R1=0.0436 for 2493 Fo>4σ(Fo).
16. SAINT Version 6.02A: Area-Detector Integration Software; Siemens Industrial Automation, Inc.: Madison, WI, 1995.
17. G. M. Sheldrick, SADABS: Area-Detector Absorption Correction, GÖttingen University, 1996.
18. G. M. Sheldrick, Acta Cryst., 2008, A64, 112.
19. G. H. Posner, Z. Li, M. C. White, V. Vinader, K. Takeuchi, S. E. Guggino, P. Dolan, and T. W. Kensler, J. Med. Chem., 1995, 38, 4529. CrossRef
20. N. Eghbali, D. S. Bohle, and D. N. Harpp, J. Org. Chem., 2006, 71, 6659. CrossRef
21. R. H. Grubbs and T. A. Pancoast, J. Am. Chem. Soc., 1977, 99, 2382. CrossRef
22. V. Singh, S. Q. Alam, and D. G. Praveena, Tetrahedron, 2002, 58, 9729. CrossRef
23. M. Bar-Zeev and S. Gothilf, J. Med. Entomol., 1973, 10, 71.
24. E. Baciocchi and C. Rol, J. Org. Chem., 1977, 42, 3682. CrossRef
25. V. A. Soloshonok, X. Tang, and V. Hruby, Tetrahedron, 2001, 57, 6375 CrossRef