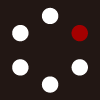
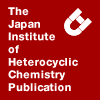
HETEROCYCLES
An International Journal for Reviews and Communications in Heterocyclic ChemistryWeb Edition ISSN: 1881-0942
Published online by The Japan Institute of Heterocyclic Chemistry
e-Journal
Full Text HTML
Received, 4th August, 2008, Accepted, 18th September, 2008, Published online, 22nd September, 2008.
DOI: 10.3987/COM-08-S(F)94
■ Benzimidazoline-Dimethoxypyrene. An Effective Promoter System for Photoinduced Electron Transfer Promoted Reductive Transformations of Organic Compounds
Eietsu Hasegawa,* Harumi Hirose, Kosuke Sasaki, Shinya Takizawa, Takayuki Seida, and Naoki Chiba
Department of Chemistry, Faculty of Sciences, Niigata University, Ikarasi 2-8050, Niigata 950-2181, Japan
Abstract
2-(p-Methoxyphenyl)-1,3-dimethylbenzimidazoline (ADMBI) and 2-(o-hydroxyphenyl)-1,3-dimethylbenzimidazoline (HPDMBI) are used as reducing reagents in 1,8-dimethoxypyrene (1,8-DMP) sensitized, photoinduced electron transfer (PET) reactions. This system was effectively used for PET induced, reductive transformations of various organic substrates, including α,β-epoxy ketones, the olefin tethered 2-bromomethyl-1-tetralone, and o-allyloxy-iodobenzene, as well as for the deprotection reactions of dodecyl-2-benzoylbenzoate and N-sulfonylindole. The results of studies show that 1,8-DMP is a more effective sensitizer than the previously used 9-methylcarbazole for deprotection of N-methyl-4-picolinium ester.INTRODUCTION
About two decades ago, 1,3-dimethyl-2-phenylbenzimidazoline (DMPBI) was shown to be a hydride-transfer reagent for reductive transformations of organic compounds.1 Subsequently, it was demonstrated that DMPBI promotes radical reactions, in which it acts as an effective hydrogen atom-donor.2 These earlier observations suggested that DMPBI and its derivatives behave in a manner similar to biologically relevant dihydropyridines (i.e. NADH). This has been confirmed by the results of further later investigations in this area.3
In recent years, we have investigated photoinduced electron transfer (PET) reactions of carbonyl compounds and halides.4-7 In this effort, a substance possessing both electron-donating and hydrogen atom-donating abilities was required to serve as an effective reducing reagent. An evaluation of its properties suggested that DMPBI would meet these requirements. About ten years ago, we first applied DMPBI in PET promoted transformations of aromatic epoxy ketones to hydroxy ketones.8 We found that the use of DMPBI led to processes that took place in much higher product yields than those that employed previously reported PET conditions.4,9 In the reaction investigated, DMPBI serves as a two-electron- and one-proton-donor, therefore, addition of an appropriate proton-donor (ROH) is required to complete the reaction (Type 1 in Scheme 1). A second break-through occurred when 2-(o-hydroxyphenyl)-1,3-dimethylbenzimidazoline (HPDMBI) was employed in the above types of PET reactions. In this case, no additional proton-donor is required because HPDMBI acts as a two-electron- and two-proton-donor in which its phenolic group serves as a second proton source (Type 2 in Scheme 1).10 Since the time of these early studies, we developed a variety of PET promoted reactions, in which several benzimidazolines (DMBI), including DMPBI and HPDMBI, were used to achieve reductive transformation of carbonyl compounds and halides.11-16
For promoting PET reactions, photosensitization methods have been employed frequently owing to several advantageous features that do not exist with direct irradiation induced PET.17 By using photosensitization, the photosensitizer is selectively excited by the light that is not absorbed by substrates. As a result, undesired photoreactions emanating from the excited states of the substrates do not take place. Dimethylamino- or methoxy-substituted arenes have been used as sensitizers for PET promoted reductive transformations of a variety of organic compounds.18-20 Recently, we demonstrated that both 1,6-bis-dimethylaminopyrene (BDMAP) and 1,6-dimethoxypyrene (1,6-DMP) are effective sensitizers in cooperation with benzimidazolines (DMBIs) for PET reduction reactions of carbonyl compounds.16 In the photosensitization cycle followed in these processes (Scheme 2), the sensitizer first absorbs light to generate its singlet excited state, from which a single electron is transferred to a carbonyl substrate to generate the carbonyl radical anion (ketyl radical). The simultaneously formed radical cation of sensitizer is subsequently reduced by DMBI to regenerate its ground state. Finally, the formed radical cation of DMBI reacts with ketyl radicals to give reduction products.
RESULTS AND DISCUSSION
Below, we describe results arising from an investigation of PET sensitization reactions of organic substrates in which 1,8-dimethoxypyrene (1,8-DMP)21 is used for the first time as an electron donor sensitizer together with 2-(p-methoxyphenyl)-1,3-dimethylbenzimidazoline (ADMBI) and 2-(o-hydroxyphenyl)-1,3-dimethylbenzimidazoline (HPDMBI). In earlier efforts, we have demonstrated that the conversion of epoxy-ketones to the corresponding hydroxy-ketones is a useful process to evaluate new electron transfer conditions.4,5,7,8,10,12,13,16 Thus, the PET sensitization method that relies on the use of 1,8-DMP and DMBIs was first probed employing the ring opening reaction of epoxy-ketone 1a (Table 1).
As can be seen by viewing the data in Table 1, 1,8-DMP was found to be similarly effective to 1,6-DMP and BDMAP for reductive ring opening PET reactions of 1a with ADMBI and HPDMBI in DMF (entries 1 and 2).16 Notably, addition of Mg(ClO4)2 resulted in a higher conversion of 1a and an increased yield of 2a (compare entry 3 to entry 2). Solvent property is one of the key factors in governing PET reactions in solution.17 As data in Table 1 show, reaction of 1a takes place irregardless of the polarity of the solvent (entries 4~7).22 It should be noted that the relatively less polar solvent benzotrifluoride (BTF) can also be used in this process. Although BTF is suggested to be a more environmentally benign solvent than benzene and methylene chloride,23 it has not been previously used for PET reactions.24 Another notable observation is that the pale yellow solution of 1,8-DMP in BTF and methylene chloride became brown on irradiation while a significant color change is not observed when 1a and HPDMBI are present.
The expected reaction pathway followed in these reactions is outlined in Scheme 2. Irradiation selectively generates the excited state of 1,8-DMP (1,8-DMP*) whose reducing ability is similar to those of 1,6-DMP and BDMAP.25 Single electron transfer (SET) from 1,8-DMP* to 1a occurs to produce the radical cation of 1,8-DMP (1,8-DMP•+) and the radical anion of 1a (1a•–). Mg(ClO4)2 could suppress the back electron transfer from 1a•– to 1,8-DMP•+ through an ion-pair exchange effect, and therefore the quantum efficiency of the reaction increases.26 1,8-DMP•+ returns to its ground state by SET from DMBI. If SET with 1a is absent, 1,8-DMP* undergoes energy wasting decay and, in some cases decomposition occurs as is observed in BTF and methylene chloride solutions lacking 1a.
We next examined reactions of 1a in which the DMBIs are replaced by other electron donors, including ascorbic acid (AA)19,27 and 3,5-bis(ethoxycarbonyl)-2,6-dimethylpyridine (Hantzch dihydropyridine, HDHP)10,28 which have been previously used for PET reactions. As the results presented in Table 2 demonstrate, by using AA, Et3N and HDHP reaction does not take place and 1a is recovered almost quantitatively (entries 1-3). On the other hand, Me2NPh promotes reaction of 1a to some extent (entry 4) and, as already described, both HPDMBI and ADMBI are effective. These observations can be understood by considering the thermodynamic/kinetic feasibility of SET from these donors to 1,8-DMP•+. Looking at the calculated free energy changes (ΔG) given in Table 2, it is seen that endoergonic SET is expected for AA, Et3N and HDHP whose oxidation potentials are greater than that of 1,8-DMP. In contrast SET between 1,8-DMP•+ and Me2NPh, HPDMBI or ADMBI, whose oxidation potentials are lower than that of 1,8-DMP, should be exoergonic.
On the basis of above observations, photosensitization system consisting of 1,8-DMP and DMBIs is expected to be ideally suited for PET reduction reactions of a variety of substances. To probe the viability of this proposal, we examined two representative dehalogenation reactions of the olefin tethered 2-bromomethyl-1-tetralone 314 and o-allyloxy iodobenzene 512 which produce the corresponding products 4 and 6 through an 5-exo radical cyclization pathway29 (Scheme 3 and Table 3).
PET spirocyclization reaction of 3 to give 4, sensitized by 1,8-DMP and ADMBI proceeds both in DMF and BTF in yields that are comparable to those promoted by using 1,6-DMP and BDMAP (entries 1 and 2).16 In the case of BTF, precipitation of imidazolium bromide interfered with light absorption by 1,8-DMP. Addition of water to the BTF solution solved this problem to some extent, and the conversion of 3 and the yield of 4 increased slightly (entry 3). The 1,8-DMP and ADMBI based photosensitization method was also effective in inducing the dehalogenation/cyclization reaction of olefin tethered aromatic iodide 5 in DMF and BTF although the yields of 6 were relatively low (entries 4 and 5). Notably, when ADMBI is replaced by the hydrogen atom donor 1,4-cyclohexadiene (CHD, 53 equiv), only a trace amount of 6 is detected.
In some cases, above the DMBIs act as effective reducing reagents for PET reactions carried out in the absence of 1,8-DMP. This technique serves as a practical alternative particularly for reactions of substrates which absorb Pyrex™-glass filtered light of which ordinary photoreaction vessels are made. For example, irradiation (λ > 280 nm) of 1,3-diphenyl-2-propene-1-one oxide 1b (chalcone epoxide, 0.40 mmol) with HPDMBI (1.2 equiv) in THF or BTF (4.0 mL) for 1 h leads to production of the desired hydroxy-ketone 2b (81% yield at 84% conv of 1b in THF; 91% yield at 81% conv of 1b in BTF). Similarly, when 3 (0.50 mmol) was irradiated with ADMBI (1.2 equiv) in MeCN or BTF (5.0 mL) for 4 h, 4 was obtained in moderate to good yields (60% in MeCN; 74% at 98% conv of 3 in BTF). Also, irradiation of 5 (0.20 mmol) with ADMBI (1.2 equiv) in BTF (4.0 mL) for 4 h gave 6 in a 35% yield at 73% conversion of 5.
Photodeprotection reaction is recognized as a useful technique in photolithography as well as chemical biology.30 As described above, BTF was found to be a tolerable solvent for PET reactions. Then, we decided to apply 1,8-DMP sensitized PET method in various solvents including BTF to three representative deprotection reactions.
Nitrobenzyl and phenacyl groups have been used previously for photoremovable protecting groups for alcohols and amines, especially in peptide synthesis.31 About ten years ago, Porter, et al. reported that benzoylesters of alcohols are removed efficiently by using photochemical methods. For example, irradiation (λ > 280 nm) of dodecyl 2-benzoyl benzoate 7 with cyclohexyl amine (10 equiv) in 1:1 benzene and MeCN leads to generation of dodecanol 8 and the lactone 9 (95% each).32 When 1,8-DMP
sensitized method using DMBI was applied to the deprotection reaction of 7, the desired dodecanol 8 is obtained in excellent yields (> 90%) (Table 4). While 9 is also obtained in reasonable yields in DMF, only small amount of this substance is detected when BTF is used as solvent. This finding is difficult to explain. A plausible mechanism for the deprotection reaction is shown in Scheme 4. Ketyl radical (7•–) accepts two protons and a single electron from DMBI•+ and AcOH to produce an intermediate diarylcarbinol which then undergoes lactonization to produce 8 and 9.
Desulfonylation reactions of N-sulfonamides generally take place under relatively vigorous conditions.33 Recently, Padwa, et al. reported a PET based mild desulfonylation method using Et3N and nBu3SnH. This method was applied to the desulfonylation reaction of N-sulfonylindole 10, which produces the deprotected product 11 (96%).34 Accordingly, sulfonyl substitution at the benzene-ring occurs in the absence of nBu3SnH. We believed that this methodology, using an environmentally undesired organotin reagent and high energy short wavelength light (254 nm), could be advantageously modified by substituting the PET conditions we have developed (Table 5). Indeed, desulfonylation of the N-sulfonylindole 10 takes place smoothly and in excellent yield by employing PET reaction with 1,8-DMP and HPDMBI in MeCN (entry 1). Although a longer irradiation time is required when reaction is carried out in BTF, the yield of 11 remains high. The expected pathway followed in this process is shown in Scheme 5. Radical anion 10•–, formed by SET between 1,8-DMP* and 10 (Ered1/2 = -0.95 V vs
SCE), undergoes desulfonylation to give indole anion and sulfonyl radical which accept a respective proton and hydrogen atom from HPDMBI•+ to produce 11 and PhSO2H.
The final process we have explored is the deprotection of N-methylpicolinium carboxylate 12 that was first reported by Falvey and co-workers.35 In Falvey’s work, a stoichiometric amount of 9-methylcarbazol (9-MC) was used as a sensitizer and an excess amount of CHD was employed as a hydrogen atom and proton donor. We reexamined this process using both 1,8-DMP and 9-MC with CHD in MeCN (Table 6). Using stoichiometric amounts of these sensitizers, no significant differences in conversion and product
yields are detected (entries 1 and 2). However, when the quantities of the sensitizers are decreased to catalytic levels and irradiation takes place with longer wavelength light, significant differences appear between the 9-MC and 1,8-DMP promoted reactions (entries 3 and 4).
The large differences observed between PET reaction of 12 sensitized by 1,8-DMP versus 9-MC appears to be due to differences in their UV-VIS absorption profiles. Namely, end-absorption of 9-MC is around 360 nm while that of 1,8-DMP extends to around 400 nm in MeCN while oxidizaing abilities of their excited states are comparable.25,36 Another notable observation is that the results of cyclic voltammometric studies show that 9-MC displays irreversible redox behavior while 1,8-DMP behaves reversibly. This finding suggests that the radical cation formed from 9-MC is less stable than that derived from 1,8-DMP. A plausible mechanistic route for PET deprotection of 12 is outlined in Scheme 6. Accordingly,35 the radical 15, generated from SET between 1,8-DMP* and 12 (Ered1/2 = -0.95 V vs SCE), undergoes fragmentation to give diphenylacetate and the picolinium radical, which then abstract a respective proton and hydrogen atom to form 13 and 14, repectively.
The interesting issues in this process are, 1) what process intervenes to return 1,8-DMP•+ to its neutral form to participate in the catalytic cycle, and 2) what species donates a proton to the acetate. Unlike DMBIs, CHD does not have a sufficiently strong electron donating ability (+1.74 V vs SCE)37 to transfer single electron to 1,8-DMP•+. A more plausible candidate for this role is the CHD radical that is generated by hydrogen atom transfer between CHD and the picolinium radical (Scheme 7).38 SET between the CHD radical and 1,8-DMP•+ generates the CHD cation that should be acidic enough to protonate acetate anion and produce benzene.
CONCLUSION
Electron transfer is a fundamental process that produces charged radicals that serve as intermediates in many chemical and biological reduction and oxidation (redox) reactions.39,40 While the use of redox reagents as well as electrochemical procedures are common ways to ion radical species, reactive species in organic chemistry, PET represents a mild, practical alternative.17 Above, we have demonstrated that DMBI and 1,8-DMP is a useful promoter system for promoting PET reductive transformations of a variety of organic compounds. Among characteristic features of the PET method based on this combination are that 1) two types of DMBIs can be chosen as reducing agents in PET promoted reductions, 2) 1,8-DMP is an effective photosensitizer that absorbs incident light near the visible region, thus avoiding unwanted photoreactions of substrates, 3) DMBIs are compatible with 1,8-DMP sensitized PET reaction conditions while DMBIs can be also used in the absence of sensitizers, 4) 1,8-DMP is not only effective with DMBIs but also with CHD, and 5) BTF, proposed as an environmentally benign solvent, can be employed in these PET reaction conditions.
EXPERIMENTAL
General procedures.
NMR spectra were recorded in CDCl3 with Me4Si as an internal standard at 200 MHz and 270 MHz for 1H NMR, and 50 MHz and 68 MHz for 13C NMR. Uncorrected melting points are reported. Absorption and fluorescence spectra were measured in MeCN. Oxidation and reduction potentials in MeCN were measured with cyclic voltammetry according to the previously reported procedure.16 Photoreactions were conducted in a Pyrex test tube (1.5 cm diameter) immersed in a water bath at room temperature with a 500 W Xe lamp as a light source. Column chromatography was performed with silica gel (Wakogel C-200). Preparative TLC was performed on 20 cm x 20 cm plates coated with silica gel (Wakogel B-5F). Anhydrous DMF was purchased and used for the photoreactions. MeCN was distilled over P2O5 and subsequently with K2CO3. THF was distilled over sodium–benzophenone under N2. Other reagents and solvents were purchased and used without further purification. ADMBI and HPDMBI were prepared by the previously reported methods.15 1,8-DMP was similarly prepared to 1,6-DMP16 by the slight modification of the literature procedure41 (see below). 9-MC42 and HDHP43 were prepared according the to literature reported procedures. Substrates (1a,44 1b,4 3,16 5,12 7,32 10,34 and 1235) and photoproducts (2a,44 2b,4 4,16 6,12 8,32 9,32 11,34 13,35 and 1435) are known compounds.
Preparation and property of 1,8-DMP.
Procedure to obtain the mixture of 1,6-pyrenedione and 1,8-pyrenedione was previously reported.16 A typical procedure is given as follows. To the crude solid containing a mixture of 1,6-pyrenedione and 1,8-pyrenedione (13 mmol), and n-Bu4NBr (419 mg, 1.3 mmol) in aqueous THF (THF + H2O = 58 mL + 20 mL) was added Na2S2O4 (13.6 g, 78 mmol) in H2O (40 mL). The resulting mixture was stirred at room temperature for 1 h. Then, KOH (16.8 g, 299 mmol) in H2O (40 mL) was added, and Me2SO4 (25.8 mL, 273 mmol) was slowly added in an ice–water bath. After the resulting mixture was stirred at room temperature for 22 h, addition of H2O was followed by extraction with CH2Cl2. The extract was washed with H2O and dried over anhydrous MgSO4, and then concentrated in vacuo. Silica-gel column chromatography of the residue (CH2Cl2 / nC6H14 = 1 / 2) gave a mixture of 1,6-dimethoxypyrene and 1,8-dimethoxypyrene (724 mg, 2.76 mmol, 21%). This mixture was subjected to fractional crystallization three times from C6H5Cl, and then 1,6-DMP (239 mg, 0.91 mmol, 7%) was obtained as yellow needles: mp 246.5–247.0 °C (lit.,41 244–245 °C). Then, 1,8-DMP was concentrated in the filtrate, from which 1,8-DMP (142 mg, 0.54 mmol, 4%) was obtained as yellow needles: mp 208.3-210.0 °C (lit.,45 201-202 °C). 1,6-DMP: IR (KBr) 2950, 1518, 1030 cm-1; 1H-NMR (270 MHz) δ 4.17 (s, 6H), 7.54 (d, 2H, J = 8.37 Hz), 7.94 (d, 2H, J = 9.18 Hz), 8.06 (d, 2H, J = 8.37 Hz), 8.26 (d, 2H, J = 9.18 Hz); 1,8-DMP: IR (KBr) 2950, 1588, 1020 cm-1; 1H-NMR (270 MHz) δ 4.16 (s, 6H), 7.51 (d, 2H, J = 8.51 Hz), 7.77 (s, 2H), 8.03 (d, 2H, J = 8.51 Hz), 8.41 (s, 2H); 13C-NMR (68 MHz) δ 56.1 (q), 108.0 (d), 120.1 (d), 120.8 (s), 124.5 (d), 124.7 (d), 125.6 (s), 125.9 (s), 152.9 (s).
Solubilities of 1,6-DMP and 1,8-DMP in several solvents were qualitatively checked by adding DMP (1 mmol) into a solvent (1.0 mL). Both 1,6-DMP and 1,8-DMP were insoluble in MeOH. While 1,6-DMP was not completely dissolved in MeCN and BTF, 1,8-DMP was soluble in these solvents. Both 1,6-DMP and 1,8-DMP were soluble in DMF, CH2Cl2, and THF.
General procedure of PET reactions.
A solution of a substrate (1, 3, 5, 7, 10: 0.20–0.50 mmol) and DMBI (0.24–0.60 mmol) with or without 1,8-DMP (1.0–2.5 x 10-2 mmol) in a solvent (DMF, MeCN, CH2Cl2, THF, BTF 2.0–5.0 mL), in the presence or absence of AcOH (1.40 mmol) or Mg(ClO4)2 (0.24 mmol), was purged with N2 for 5-10 min prior to irradiation. In the case of 12, a solution of 12 (0.02 mmol), CHD (1.0 mL, 10.6 mmol) and 9-MC or 1,8-DMP (0.02 mmol or 0.001 mmol) in MeCN (4.0 mL) was purged with N2 for 10 min before irradiation. These solutions were irradiated with the light using cut-off glass-filters (λ > 320 nm, 340 nm, and 360 nm for sensitization reactions) or not using a filter (λ > 280 nm through Pyrex) for an appropriate irradiation time. Photolysates obtained from the reactions of 1a, 5, 7 in DMF and 5 in BTF were subjected to the extraction with Et2O for 1a and EtOAc for 5, 7. The extracts were washed with water and satd. aqueous NaCl, and dried over anhydrous MgSO4 and then concentrated. Photolysates from other reactions in MeCN, CH2Cl2, THF, and BTF were directly concentrated. The resulting residues were analyzed with 1H-NMR using triphenylmethane for 1a, 3, 7, 1,4-dimethoxybenzene for 5, and 1,3,5-trimethoxybenze for 12 as internal standards. For reactions of 1b and 10, the residues were subjected to silica-gel column or TLC separation using appropriate solvents (column: CH2Cl2 for 1b, TLC: EtOAc / n-C6H14 = 1 / 3 for 10) to give the starting materials and the products. Photoproducts were identified by analyses with their NMR and IR spectra.
ACKNOWLEDGEMENTS
We are grateful to Professor Patrick S. Mariano (University of New Mexico, USA) for his valuable comments and editing. This work was partly supported by the grant from the Uchida Energy Science Promotion Foundation.
This paper is dedicated to Professor Emeritus Keiichiro Fukumoto on the occasion of his 75th birthday.
References
1. H, Chikashita and K. Itoh, Bull. Chem. Soc. Jpn., 1986, 59, 1747; CrossRef H. Chikashita, H. Ide, and K. Itoh, J. Org. Chem., 1986, 51, 5400. CrossRef
2. J. J. Chen and D. D. Tanner, J. Org. Chem., 1988, 53, 3897; CrossRef D. D. Tanner and J. J. Chen, J. Org. Chem., 1989, 54, 3842; CrossRef D. D. Tanner, J. J. Chen, L. Chen, and C. Luelo, J. Am. Chem. Soc., 1991, 113, 8074; CrossRef D. D. Tanner and J. J. Chen, J. Org. Chem., 1992, 57, 662; CrossRef D. D. Tanner, J. J. Chen, C. Luelo, and P. M. Peters, J. Am. Chem. Soc., 1992, 114, 713. CrossRef
3. I-S. H. Lee, E. H. Jeoung, and M. M. Kreevoy, J. Am. Chem. Soc., 1997, 119, 2722; CrossRef I-S. H. Lee and E. H. Jeoung, J. Org. Chem., 1998, 63, 7275; CrossRef I-S. H. Lee, E. H. Jeoung, and M. M. Kreevoy, J. Am. Chem. Soc., 2001, 123, 7492; CrossRef X-Q. Zhu, M-T. Zhang, A. Yu, C-H. Wang, and J-P. Cheng, J. Am. Chem. Soc., 2008, 130, 2501. CrossRef
4. E. Hasegawa, K. Ishiyama, T. Horaguchi, and T. Shimizu, J. Org. Chem., 1991, 56, 1631. CrossRef
5. E. Hasegawa, K. Ishiyama, T. Fujita, T. Kato, and T. Abe, J. Org. Chem., 1997, 62, 2396. CrossRef
6. E. Hasegawa, Y. Tamura, T. Horaguchi, K. Isogai, and T. Suzuki, Tetrahedron Lett., 1994, 35, 8643; CrossRef E. Hasegawa, H. Katagi, D. Nakagawa, T. Horaguchi, S. Tanaka, and Y. Yamashita, Tetrahedron Lett., 1995, 36, 6915; CrossRef E. Hasegawa, Y. Tamura, K. Suzuki, A. Yoneoka, and T. Suzuki, J. Org. Chem., 1999, 64, 8780. CrossRef
7. E. Hasegawa, J. Photoscience, 2003, 10, 61.
8. E. Hasegawa, T. Kato, T. Kitazume, K. Yanagi, K. Hasegawa, and T. Horaguchi, Tetrahedron Lett., 1996, 37, 7079. CrossRef
9. J. Cossy, A. Bouzide, S. Ibhi, and P. Aclinou, Tetrahedron, 1991, 47, 7775. CrossRef
10. E. Hasegawa, N. Chiba, T. Takahashi, S. Takizawa, T. Kitayama, and T. Suzuki, Chem. Lett., 2004, 33, 18. CrossRef
11. E. Hasegawa, Y. Tamura, and E. Tosaka, J. Chem. Soc., Chem. Commun., 1997, 1895. CrossRef
12. E. Hasegawa, A. Yoneoka, K. Suzuki, T. Kato, T. Kitazume, and K. Yanagi, Tetrahedron, 1999, 55, 12957. CrossRef
13. E. Hasegawa, N. Chiba, A. Nakajima, K. Suzuki, A. Yoneoka, and K. Iwaya, Synthesis, 2001, 1248. CrossRef
14. E. Hasegawa, S. Takizawa, K. Iwaya, M. Kurokawa, N. Chiba, and K. Yamamichi, J. Chem. Soc., Chem. Commun., 2002, 1966.. CrossRef
15. E. Hasegawa, T. Seida, N. Chiba, T. Takahashi, and H. Ikeda, J. Org. Chem., 2005, 70, 9632. CrossRef
16. E. Hasegawa, S. Takizawa, T. Seida, A. Yamaguchi, N. Yamaguchi, N. Chiba, T. Takahashi, H. Ikeda, and K. Akiyama, Tetrahedron, 2006, 62, 6581. CrossRef
17. ‘Photoinduced Electron Transfer Parts A-D’ eds. by M. A. Fox and M. Chanon, Elsevier, Amsterdam, 1988; G. J. Kavarnos, ‘Fundamental of Photoinduced Electron Transfer’ VCH Press, New York, 1993; ‘CRC Handbook of Organic Photochemistry and Photobiology, 1st ed’ ed. by W. M. Horspool and P. S. Song, CRC Press, Boca Raton, 1994; and ‘2nd ed’ ed. by W. M. Horspool and F. Lenci, CRC Press, Boca Raton, 2004; J. Cossy and D. Belloti, Tetrahedron, 2006, 62, 6459; CrossRef A. G. Griesbeck, N. Hoffmann, and K. D. Warzecha, Acc. Chem. Res., 2007, 40, 128; CrossRef P. E. Floreancig, Synlett., 2007, 191; CrossRef P. A. Waske, N. T. Tzvetkov, and J. Mattay, Synlett., 2007, 669; CrossRef N. Hoffmann, Chem. Rev., 2008, 108, 1052; CrossRef N. Hoffmann, J. Photochem. Photobio. C, 2008, 9, 43. CrossRef
18. J. A. Lee and S. H. Israel, J. Org. Chem., 1983, 48, 4557; CrossRef A. Nishida, T. Hamada, and O. Yonemitsu, J. Org. Chem., 1988, 53, 3386; CrossRef G. Pandey, K. S. S. P. Rao, and K. V. N. Rao, J. Org. Chem., 1996, 61, 6799; CrossRef A. Banerjee and D. F. Falvey, J. Org. Chem., 1997, 62, 6245; CrossRef T. Mikami and K. Narasaka, Chem. Lett., 2000, 338; CrossRef D. Armesto, M. J. Ortiz, A. R. Afgarrrabeitia, and M. M-Fontecha, Org. Lett., 2004, 6, 2261; CrossRef M. Kitamura, Y. Mori, and K. Narasaka, Tetrahedron Lett., 2005, 46, 2373; CrossRef N. Narasaka and M. Kitamura, Eur. J. Org. Chem., 2005, 4505; CrossRef D. E. Falvey and C. Sundararajan, Photochem. Photobiol. Sci., 2006, 5, 116; CrossRef G. Pandey, A. L. Gaikwad, and S. R. Gadre, Tetrahedron Lett., 2006, 47, 701. CrossRef
19. T. Hamada, A. Nishida, and O. Yonemitsu, J. Am. Chem. Soc., 1986, 108, 140 ; CrossRef G. Pandey, S. Hajira, M. K. Ghorai, and K. R. Kumar, J. Am. Chem. Soc., 1997, 119, 8777; CrossRef G. Pandey, K. S. S. P. Rao, D. K. Palit, and J. P. Mittal, J. Org. Chem., 1998, 63, 3968; CrossRef G. Pandey, K. S. S. P. Rao, and K. V. N. Rao, J. Org. Chem., 2000, 65, 4309. CrossRef
20. K. Okada, K. Okamoto, and M. Oda, J. Am. Chem. Soc., 1988, 110, 8736; CrossRef T. Mikami and K. Narasaka, R. Acad. Sci. Paris, Ser. IIc, Chim., 2001, 4, 447.
21. As previously reported,16 both BDMAP and 1,6-DMP are reasonably effective sensitizers. However some problems are associated with these compounds. For example, BDMAP is reported to often undergo decomposition during irradiation, 20 and the solubility of 1,6-DMP was found to be low in MeCN that is commonly used for PET reactions. On the other hand, 1,8-DMP is readily soluble in MeCN (see Experimental Section).
22. Polarity of the solvents estimated by ET30 (kcal/mol): 45.7, 43.3, 41.0, 39.3, 37.4 for MeCN, DMF, CH2Cl2, C6H5CF3, THF, respectively.24.
23. A. Ogawa and D. P. Curran, J. Org. Chem., 1997, 62, 450; CrossRef J. J. Maul, P. J. Ostrowski, G. A. Ublacker, B. Linclau, and D. P. Curran, ‘Modern Solvents in Organic Synthesis’ ed. by P. Knochel, Springer, Berlin, 1999, pp. 79-105. CrossRef
24. Recently, we reported a first example of ground-state ET reactions in BTF: E. Hasegawa, Y. Ogawa, K. Kakinuma, H. Tsuchida, E. Tosaka, S. Takizawa, H. Muraoka, and T. Saikawa, Teterahedron, 2008, 64, 7724. CrossRef
25. On the basis of the standard potential (E° = +0.83 V vs SCE, obtained from reversible cyclic voltammogram) and the excitation energy (Eex = 73.0 kcal/mol, 3.16 eV) of 1,8-DMP, oxidation potential of its excited state (Eox*) is estimated to be -2.33 V by using the equation of Eº - Eex. Eox* values for 1,6-DMP and BDMAP can be similarly caluculated to be -2.27 and -2.34 V based on the reported data.16.
26. Representative examples of inorganic salt effects on PET reactions. J. D. Simon and K. S. Peters, J. Am. Chem. Soc., 1983, 105, 4875; CrossRef K. Mizuno, N. Ichinose, and Y. Otsuji, Chem. Lett., 1985, 455; CrossRef Review : A. Loupy, B. Tchoubar, and D. Astruc, Chem. Rev., 1992, 92, 1141. CrossRef
27. Q. Liu, B. Han, Z. Liu, L. Yang, Z. L. Liu, and W. Yu, Tetrahedron Lett., 2006, 47, 1805. CrossRef
28. J. Zhang, M. Z. Jin, W, Zhang, L. Yang, and Z. L. Liu, Tetrahedron Lett., 2006, 47, 9687.
29. D. Griller and K. U. Ingold, Acc. Chem. Res., 1980, 13, 317; CrossRef A. L. J. Beckwith and C. H, Schiesser, Tetrahedron, 1985, 41, 3925. CrossRef
30. M. C. Pirrung, Chem. Rev., 1997, 97, 473; CrossRef M. C. Pirrung, L. Fallon, and G. McGall, J. Org. Chem. 1998, 63, 241; CrossRef C. G. Bochet, J. Chem. Soc., Perkin Trans. 1, 2002, 125. CrossRef
31. P. DeMayo, Adv. Org. Chem., 1960. 2, 367; J. C. Sheeehan and K. Umezawa, J. Org. Chem., 1973, 38, 3771. CrossRef
32. P. B. Jones, M. P. Pollastri, and N. A. Porter, J. Org. Chem., 1996, 61, 9455. CrossRef
33. H. R. Snyder and R. E. Heckert, J. Am. Chem. Soc., 1952, 74, 2006; CrossRef E. J. Gilbert, J. D. Chisholm, and D. L. Van Vranken, J. Org. Chem., 1999, 64, 5670; CrossRef N. K. Garg, R. Sarpong, and B. M. Sroltz, J. Am. Chem. Soc., 2002, 124, 13179. CrossRef
34. A. Padwa, A. France, J. M. Mejia-Onte, and X. Hong, Tetrahedron Lett., 2006, 47, 2409. CrossRef
35. D. E. Falvey and C. Sundararajan, J. Org. Chem., 2004, 69, 5547. CrossRef
36. On the basis of half-wave oxidation potential (Eox1/2 = +1.18 V vs SCE) and the excitation energy (Eex = 82 kcal/mol, 3.55 eV)35 of 9-MC, its excited state oxidation potential (Eox*) is calculated to be -2.37 V.
37. T. Shono, A. Ikeda, J. Hyashi, and S. Hakozaki, J. Am. Chem. Soc., 1975, 97, 4261. CrossRef
38. Although the oxidation potential of CHD radical is unknown, it would be lower than that of 1,8-DMP,25 on the basis of the reported data for some conjugated alkyl radicals such as PhCH2, PhMeCH, Ph2CH radicals (Eox1/2 = +0.73, +0.37, and +0.35 V vs SCE): D. D. M. Wayner, D. J. McPhee, and D. Griller, J. Am. Chem. Soc., 1988, 110, 132. CrossRef
39. L. Eberson, ‘Electron Transfer Reactions in Organic Chemistry’ Springer-Verlag, Berlin, 1987; ‘Advances in Electron Transfer Chemistry,’ Vols 1-6, ed. by P. S. Mariano, JAI Press, Greenwich, 1991-1999; ‘Electron Transfer in Chemistry,’ Vols 1-5, ed. by V. Balzani, Wiley-VCH, Weinheim, 2001.
40. M. Schmittel and A. Burghart, Angew. Chem., Int. Ed. Engl., 1997, 36, 2550; CrossRef D. J. Berger and J. M. Tanko, ‘The Chemistry of Double-Bonded Functional Groups’ ed. by S. Patai, Wiley, New York, 1997, pp. 1281-1354; CrossRef (c) M. Schmittel and M. K. Ghorai, ‘Electron Transfer in Chemistry’ ed. by V. Balzani, Wiley-VCH, Weinheim, 2001, Vol. 2, pp. 5-54; CrossRef H. D. Roth, ‘Reactive Intermediate Chmeistry’ ed. by R. A. Moss, M. S. Platz, and M. Jones, Jr., John Wiley & Sons, 2004, Chapter 6, pp. 205-272.
41. A. Zweig, A. H. Maurer, and B. G. Roberts, J. Org. Chem., 1967, 32, 1322. CrossRef
42. D. E. Falvey and C. Sundararajan, Org. Lett., 2005, 7, 2631. CrossRef
43. L. F. Tietze and Th. Eicher, Reaktionen und Synthesen im Organisch-chemischen Praktikum und Forschungslaboratorium, Georg Thieme Verlag, Stuttgart, 1991. CrossRef
44. E. Hasegawa, K. Ishiyama, T. Kato, T. Horaguchi, T, Shimizu, S. Tanaka, and Y. Yamashita, Y. J. Org. Chem., 1992, 57, 5352. CrossRef
45. E. Profft and R. Biela, J. Prakt. Chem., 1963, 20, 87 CrossRef