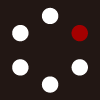
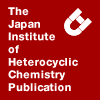
HETEROCYCLES
An International Journal for Reviews and Communications in Heterocyclic ChemistryWeb Edition ISSN: 1881-0942
Published online by The Japan Institute of Heterocyclic Chemistry
e-Journal
Full Text HTML
Received, 5th August, 2008, Accepted, 12th September, 2008, Published online, 18th September, 2008.
DOI: 10.3987/COM-08-S(F)96
■ Synthesis and Structures of Alkynylchlorocyclotriphosphazenes and Their Cluster-forming Reactions with Octacarbonyldicobalt
Takashi Komuro, Kenichi Mori, and Hiromi Tobita*
Department of Chemistry, Graduate School of Science, Tohoku University, Aoba-ku, Sendai 980-8578, Japan
Abstract
Cyclotriphosphazenes having p-tolylethynyl and chloro substituents N3P3{C≡C(p-Tol)}nCl6-n (1a: n = 2, 1b: n = 4; p-Tol = p-tolyl) were synthesized by the reaction of hexachlorocyclotriphosphazene (NPCl2)3 with 2 equiv of LiC≡C(p-Tol) in THF. 1a and 1b were characterized by spectroscopic methods and elemental analyses. The reactions of 1a and 1b with octacarbonyldicobalt Co2(CO)8 produced cobalt clusters containing tetrahedral C2Co2(CO)6(p-Tol) cluster units; namely, tetranuclear cluster N3P3{C2Co2(CO)6(p-Tol)}2Cl4 (2) and hexanuclear cluster N3P3{C2Co2(CO)6(p-Tol)}3{C≡C(p-Tol)}Cl2 (3). Crystal structures of 1a and 2 were determined by X-ray crystallography.INTRODUCTION
Functionalized cyclotriphosphazenes are attractive for their application to a multi-site-coordination ligand in coordination and organometallic chemistry.1 Cyclotriphosphazenes (NPX2)3 have six exocyclic substituents X on the three phosphorus atoms (Chart 1) and can be tailored by functionalization of the substituents X with coordinating groups to construct multi-site-coordination ligands. For example, cyclotriphosphazene ligands having N-donor substituents such as pyrazolyl, pyridyloxy, and amino groups have been extensively investigated in coordination chemistry of transition metals.1 One of the unique features of the cyclotriphosphazene ligands is their potential utility as bridging ligands for the synthesis of multinuclear metal complexes.
Since an alkynyl group acts as a ligand to form organometallic complexes by coordination of the C≡C bond to transition metals, we have aimed at the synthesis of cyclotriphosphazenes having plural alkynyl groups. Although the synthesis of several alkynyl-substituted cyclotriphosphazenes2–5 and their reactivity toward cobalt and iron complexes2,5–9 have been investigated, cyclotriphosphazenes having more than two alkynyl substituents have not yet been synthesized. To prepare cyclotriphosphazenes having different numbers of alkynyl groups, we have used a salt elimination reaction between hexachlorocyclotriphosphazene (NPCl2)3 and alkynyllithium. As the closely related works, Chivers2 and Allen et al.4,5 have reported the reactions of hexafluorocyclotriphosphazene (NPF2)3 with alkynyllithium reagents LiC≡CR (R = Ph, SiMe3, Me, n-Bu) giving mono- and disubstituted alkynylfluorocyclotriphosphazenes N3P3(C≡CR)nF6–n (n = 1, 2). Hexachlorocyclotriphosphazene (NPCl2)3 is thought to be a more convenient starting material compared to the fluoride analog (NPF2)3, since the latter is a volatile liquid and is usually derived from (NPCl2)3.10 However, to the best of our knowledge, the reaction of (NPCl2)3 with alkynyllithium has not been used for P–C(alkynyl) bond formation,11 probably because the reaction of (NPCl2)3 with organolithium reagents usually results in a complicated mixture accompanied by the ring cleavage of the cyclotriphosphazene moiety.2,12 Herein, we describe the synthesis of alkynylchlorocyclotriphosphazenes having two or four p-tolylethynyl groups by the reaction between (NPCl2)3 and p-tolylethynyllithium. We also describe the reactions of the alkynylchlorocyclotriphosphazenes with octacarbonyldicobalt that give tetra- and hexanuclear cobalt clusters.
RESULTS AND DISCUSSION
Preparation of alkynylchlorocyclotriphosphazenes 1a and 1b
Treatment of hexachlorocyclotriphosphazene (NPCl2)3 with 2 equiv of LiC≡C(p-Tol) in THF gave a mixture containing bis(p-tolylethynyl)tetrachlorocyclotriphosphazene N3P3{C≡C(p-Tol)}2Cl4 (1a) and tetrakis(p-tolylethynyl)dichlorocyclotriphosphazene N3P3{C≡C(p-Tol)}4Cl2 (1b) in ca. 6 : 1 molar ratio (eq. 1).13 Compounds 1a and 1b in the mixture were separated by silica gel flash chromatography14 and isolated in 54 and 5% yields, respectively. 1a and 1b are both one of the geminal isomers, which contain only P{C≡C(p-Tol)}2 and PCl2 moieties but no P{C≡C(p-Tol)}Cl moieties.15,16 In the 31P{1H} NMR spectrum of the reaction mixture, no signals were observed for cyclotriphosphazenes having a P{C≡C(p-Tol)}Cl moiety, i.e., non-geminal isomers of 1a and 1b as well as mono- or trisubstituted cyclotriphosphazenes (vide infra). It has been reported that the reaction of (NPF2)3 with 2 equiv of LiC≡CPh in diethylether afforded the geminal isomer of bis(phenylethynyl)tetrafluorocyclotriphosphazene N3P3(C≡CPh)2F4 almost selectively,2,4 but, in this case, formation of a small amount of non-geminal isomers was also observed.4
Compounds 1a and 1b were characterized by spectoroscopic methods (NMR, IR, and MS) and elemental analyses. In the 1H and 13C{1H} NMR spectroscopy, 1a and 1b show only one set of the signals for the p-tolylethynyl groups. This indicates that all the p-tolyl groups in 1a and also in 1b are equivalent. The 31P{1H} NMR spectrum of 1a exhibits a doublet and a triplet signal. The doublet signal appears at 19.3 ppm and, since the chemical shift is comparable to that of (NPCl2)3 (20.5 ppm), it is assignable to the chloro-substituted phosphorus atoms. The triplet signal at –32.1 ppm is assignable to the p-tolylethynyl-substituted phosphorus atom, and the chemical shift is similar to that for the geminal isomer of N3P3(C≡CPh)2F4 (–23.2 ppm).4 Therefore, the replacement of chloro groups in (NPCl2)3 by p-tolylethynyl groups results in the considerable upfield shift by 52.6 ppm of the 31P signal in 1a. Similarly, the 31P{1H} NMR spectrum of 1b shows a doublet signal (–31.9 ppm) of the p-tolylethynyl-substituted phosphorus atoms and a triplet signal (18.9 ppm) of the chloro-substituted phosphorus atom. Since the 31P{1H} NMR spectrum of (phenylethynyl)pentafluorocyclotriphosphazene N3P3(C≡CPh)F5 shows the signal of the phosphorus atom in the P(C≡CPh)F moiety at 6.0 ppm,4 similar chemical shifts are expected for those in P{C≡C(p-Tol)}Cl moieties of (p-tolylethynyl)chlorocyclotriphosphazenes, if any. However, in the 31P{1H} NMR spectrum of the reaction mixture as well as those of 1a and 1b, there are no signals in the region between 15 and –25 ppm. This suggests that neither non-geminal isomers of 1a and 1b nor mono- or trisubstituted cyclotriphosphazenes were obtained as mentioned above. These NMR observations are consistent with the geminal configuration of 1a and 1b. The IR spectra of 1a and 1b exhibit a C≡C stretching band at 2179 (1a) and 2177 (1b) cm–1.
The crystal structure of 1a was unequivocally determined by X-ray crystallography (Figure 1, Table 1). The N3P3 ring is puckered in a chair-type geometry: P1 and N2 deviate from the mean plane of P2, P3, N1, and N3 by 0.37 and –0.28 Å, respectively. The P–N bonds for the p-tolylethynyl-substituted phosphorus atom P1 [P1–N1: 1.6128(17) Å, P1–N3: 1.6088(17) Å] are longer than the other P–N bonds for the chloro-substituted phosphorus atoms P2 and P3 [1.5644(17)–1.5877(17) Å] that are close to those in (NPCl2)3 [av. 1.581(3) Å].17 This elongation is attributable to the electron donation of the alkynyl groups to the phosphorus atom P1 as also observed in several mono- and diorgano-substituted cyclotriphosphazenes.18,19 The N1–P1–N3 angle [115.13(9)°] is narrower than the other N–P–N angles [av. 119.17(9)°]. Similar reduction of the corresponding N–P–N angles was observed in geminally substituted dialkyl- or diarylcyclotriphosphazenes N3P3R2Cl4 (R = alkyl, aryl).19 We could not get single crystals of 1b suitable for a full X-ray structure analysis, but a tentative analysis using a low-quality crystal of 1b revealed a N3P3 ring structure containing four p-tolylethynyl and two chloro groups bonded geminally to phosphorus atoms.
To prepare more highly substituted cyclotriphosphazenes with p-tolylethynyl groups, the reaction of (NPCl2)3 with ca. 6 equiv of LiC≡C(p-Tol) was investigated. But, the reaction resulted in a complicated mixture. The 31P{1H} NMR spectrum of the reaction mixture shows only one sharp singlet signal at –31.3 ppm with weak intensity accompanied by unresolved broad signals in the region between –40 and 30 ppm. The chemical shift of the singlet signal is comparable to those for the p-tolylethynyl-substituted phosphorus atoms in 1a and 1b, and thus the signal would be assignable to hexakis(p-tolylethynyl)cyclotriphosphazene N3P3{C≡C(p-Tol)}6 (1c). However, the content of 1c in the mixture is considerably low, and the isolation of the pure form of 1c has been unsuccessful (see Experimental section). Undesired reactions, e.g., ring cleavage of cyclotriphosphazene,2,12 may have predominantly occurred to form unidentified products.
Reactions of the ligand precursors 1a and 1b with Co2(CO)8
To estimate the ability of (p-tolylethynyl)cyclotriphosphazenes 1a and 1b as multidentate ligands, we investigated the reactions of 1a and 1b with octacarbonyldicobalt, which is well known to react with an alkyne molecule to give a Co2C2 cluster. Thus, the reaction of 1a with 2 equiv of Co2(CO)8 in toluene at room temperature produced a tetranuclear cobalt cluster N3P3{C2Co2(CO)6(p-Tol)}2Cl4 (2) in 94% yield (eq. 2). The structure of 2 was elucidated by spectroscopy (NMR, IR, and MS), elemental analysis, and X-ray crystallography (vide infra). The 31P{1H} NMR spectrum of 2 shows two inequivalent signals: one doublet at 17.0 ppm assigned to the chloro-substituted phosphorus atoms and one triplet at 26.6 ppm assigned to the p-tolylethynyl-substituted phosphorus atom. The chemical shift of the latter signal is similar to that for the alkynyl-substituted phosphorus atom in the geminal isomer of tetranuclear cobalt cluster N3P3{C2Co2(CO)6(Ph)}2F4 (32.5 ppm).8 In comparison of the 31P resonances of 2 with those of 1a, the former signal of PCl2’s is slightly (2.3 ppm) upfield shifted, while the latter signal is significantly downfield shifted by 58.7 ppm due to the coordination of the alkynyl substituents to cobalt. The coupling constant between the inequivalent phosphorus atoms (2JPP = 8.1 Hz) is considerably decreased compared to 1a (2JPP = 45 Hz). Similar decrease of the P–P coupling constant has also been observed by conversion of N3P3(C≡CPh)2F4 (2JPP = 91.1 Hz) to cluster N3P3{C2Co2(CO)6(Ph)}2F4 (2JPP = 50.1 Hz).4,8 In the IR spectrum of 2, the C≡C stretching band observed in 1a (2179 cm−1) disappeared and a new band assignable to the C–C stretching of Co2C2 cluster units appeared at 1576 cm−1.
The X-ray structure analysis of the tetranuclear cobalt cluster 2 confirmed the molecular structure consisting of a N3P3 6-membered ring and two Co2C2 tetrahedral cluster units (Figure 2, Table 2). This is the first detailed report on the crystal structure analysis of bis(hexacarbonyldicobalt) complexes of dialkynylcyclotriphosphazenes. The N3P3 ring is slightly puckered: P2 and N2 deviate from the least-squares plane of P1, P3, N1, and N3 by 0.16 and 0.27 Å, respectively. The N3P3-ring distortion seen in the P–N bonds and N–P–N angles are similar to that in 1a. Thus, the P1–N1 and P1–N3 bonds [av. 1.616(2) Å] are longer than the other P–N bonds [av. 1.573(2) Å], and the N1–P1–N3 angle [115.16(9)°] is narrower than the other N–P–N angles [av. 119.6(1)°]. The considerable decrease of the s-character in the alkynyl C≡C bonds in 2 compared to the corresponding free ligand 1a leads to the geometric changes in the P–C≡C moieties as follows: longer P1–C bond distances in 2 [av. 1.776(2) Å] compared to those in 1a [av. 1.736(2) Å], narrower P1–C1–C2 and P1–C16–C17 angles in 2 [av. 144.4(2)°] compared to the corresponding P–C–C angles in 1a [av. 169.1(2)°], and elongation of the alkynyl C–C bonds by ca. 0.15 Å from 1a to 2. The wider C1–P1–C16 angle [109.18(10)°] compared to the corresponding angle in 1a [C1–P1–C10: 105.35(10)°] reflects the larger steric repulsion between the Co2C2 units in 2.
The reaction of 1b with excess Co2(CO)8 (ca. 5 equiv) in C6D6 at room temperature in a sealed NMR tube was monitored by 1H NMR spectroscopy. The spectroscopic analysis of the final reaction mixture indicates the formation of hexanuclear cluster having an unreacted p-tolylethynyl group N3P3{C2Co2(CO)6(p-Tol)}3{C≡C(p-Tol)}Cl2 (3) as a major product in 88% NMR yield (eq. 3).20 No signals assignable to octanuclear cluster N3P3{C2Co2(CO)6(p-Tol)}4Cl2, in which all the four alkynyl groups are coordinated to cobalt atoms, have observed. The incomplete reaction is probably due to the steric hindrance in cluster 3 that prevents further complexation of a Co2(CO)6 fragment with the remaining p-tolylethynyl group. The 1H NMR spectrum of product 3 shows four inequivalent methyl signals of the p-tolylethynyl groups with 1 : 1 : 1 : 1 intensity ratio. The 31P{1H} NMR spectrum of 3 exhibits three inequivalent signals of the phosphrous atoms in the N3P3 ring at 1.1, 17.7, and 24.1 ppm. Comparing the 31P NMR chemical shifts of 3 with those in 2 (PCl2: 17.0 ppm, P{C2Co2(CO)6(p-Tol)}2: 26.6 ppm), the signals at 17.7 and 24.1 ppm can be assigned to the phosphorus atoms in the PCl2 and P{C2Co2(CO)6(p-Tol)}2 moieties, respectively. The third signal at 1.1 ppm is assignable to the phosphorus atom in the partially complexed P{C2Co2(CO)6(p-Tol)}{C≡C(p-Tol)} moiety, which is downfield shifted by 33 ppm compared to the signal (–31.9 ppm) of the p-tolylethynyl-substituted phosphorus atoms in 1b. The analogous 32 ppm downfield-shift by coordination of one alkynyl group on cyclotriphosphazene to a Co2(CO)6 fragment has been reported for the reaction of (phenylethynyl)pentafluorocyclotriphosphazene N3P3(C≡CPh)F5 with Co2(CO)8 to give N3P3{C2Co2(CO)6(Ph)}F5.8 In the high-resolution electrospray mass spectrometry of the reaction mixture, the peak (m/z = 1545.5847) assignable to the Na+ adduct of the parent molecule was observed when NaI was added to the sample. The IR spectrum of the reaction mixture shows four terminal CO stretching bands of 3 in the range of 2033−2100 cm−1. These observations support the structural characterization of 3. Since the C6D6 or toluene solution of product 3 is thermally unstable under nitrogen atmosphere at room temperature, we have not succeeded in isolation of 3 from the reaction mixture in a synthetic-scale experiment. This makes the further characterization of 3 difficult. The thermal lability would be caused by the facile dissociation of CO ligands in 3. This was supported by the experimental result that the decomposition of 3 in toluene solution was accelerated under reduced pressure.
CONCLUSION
We synthesized (p-tolylethynyl)chlorocyclotriphosphazenes 1a and 1b by the salt elimination reaction between (NPCl2)3 and LiC≡C(p-Tol). The products were isolated in pure form and characterized. The capability of 1a and 1b as multidentate ligands was confirmed by their reactions with Co2(CO)8, where tetra- and hexanuclear cobalt clusters 2 and 3 were obtained from 1a and 1b, respectively. It is worth noting that 1b is the first example of the cyclotriphosphazene having four alkynyl groups and, of course, the hexanuclear structure of the cobalt cluster 3 is unprecedented. Our ongoing works are (1) exploration of a high-yield synthesis of hexaalkynylcyclotriphosphazene 1c, (2) isolation and full-characterization of hexanuclear cobalt cluster 3, and (3) investigation of the reactions of p-tolylethynyl-substituted phosphazenes 1a and 1b with other transition-metal complexes that provide different multinuclear clusters.
EXPERIMENTAL
General Procedures
All manipulations of air- and moisture-sensitive compounds were carried out under an inert atmosphere in a N2-filled glovebox or using standard high-vacuum-line and Schlenk techniques. CDCl3 was dried and vacuum-transferred from CaH2, and then stored under nitrogen over 4 Å molecular sieves. Hexane and toluene were dried over sodium/benzophenone and distilled before use. Dehydrated THF was purchased from Kanto Chemical Co., Inc. and used without further purification. Hexachlorocyclotriphosphazene (NPCl2)3 was recystallized with hot hexane. 4-Ethynyltoluene (Kanto Chemical) was dried over CaH2 and distilled prior to use. Octacarbonyldicobalt Co2(CO)8 (Kanto Chemical) was purchased and used as received. Flash chromatography14 was performed using silica gel 60N (Kanto Chemical, 40-50 µm, spherical, neutral) or activated alumina (Wako Pure Chemicals Industries, Ltd., 45 µm). 1H, 13C{1H}, and 31P{1H} NMR spectra were recorded on a Bruker AVANCE-300 Fourier transform spectrometer, and chemical shifts were reported in parts per million. The residual proton (C6D5H: 7.15 ppm, CHCl3: 7.24 ppm) and the carbon (C6D6: 128.0 ppm, CDCl3: 77.0 ppm) resonances of deuterated solvents were used as internal references for 1H and 13C resonances, respectively. Aromatic carbon or proton is abbreviated to ArC or ArH. 31P{1H} NMR chemical shifts were referenced to 85% H3PO4 as an external standard. Infrared spectra were recorded using a HORIBA FT-730 spectrometer. High-resolution mass spectra (HRMS) were collected on a Bruker Daltonics APEX-(III) spectrometer operating in the electrospray ionization (ESI) mode, when NaI was added to the sample. Elemental analyses were carried out using a Yanaco MT-6 and a Yanaco HNS-15/HSU-20 microanalyzers. Mass spectrometric analyses and elemental analyses were performed at the Research and Analytical Center for Giant Molecules, Tohoku University.
Synthesis of N3P3{C≡C(p-Tol)}2Cl4 (1a) and N3P3{C≡C(p-Tol)}4Cl2 (1b)
A THF/hexane solution of p-tolylethynyllithium LiC≡C(p-Tol) was prepared as follows: To a solution of 4-ethynyltoluene (1.165 g, 10.03 mmol) in THF (30 mL) was slowly added n-BuLi (1.52 M in hexane, 7.5 mL, 11 mmol) via a syringe at ca. −90 °C, and then the mixture was stirred for 1 h at this temperature. To this solution of LiC≡C(p-Tol) was added dropwise a solution of (NPCl2)3 (1.742 g, 5.011 mmol) in THF (40 mL) at −80 °C for 15 min. The yellow reaction mixture was allowed to warm up to room temperature. The reaction was monitored by 31P{1H} NMR spectroscopy until the relative intensity of signals became constant. After stirring at room temperature overnight, the dark-brown solution was evaporated under vacuum. The dark-brown residue was extracted three times with toluene (25, 7.5, 7.5 mL), and the extracts were centrifuged. The supernatants were combined and evaporated. The residue was separated by flash chromatography on silica gel (eluent: toluene/hexane 1 : 1), and three fractions were obtained. Evaporation of the first colorless fraction gave a colorless solid, and then recrystallization of the solid from toluene gave colorless crystals of 1a in 54% yield (1.37 g, 2.71 mmol). From the second yellow fraction, an unidentified yellow oil (0.047 g) was obtained. From the third fraction, pale-yellow crystals of 1b (0.180 g, 0.270 mmol) were obtained in 5% yield. The fourth fraction was obtained by elution with THF and was evaporated to give unidentified products as a brown oil (0.765 g).
Data for 1a: 1H NMR (300 MHz, CDCl3): δ 2.37 (s, 3H, CH3), 7.18 (d, 2H, 3JHH = 8.0 Hz, ArH), 7.49 (d, 2H, 3JHH = 8.0 Hz, ArH). 31P{1H} NMR (121.5 MHz, CDCl3): δ –32.1 (t, 2JPP = 45 Hz, P{C≡C(p-Tol)}2), 19.3 (d, 2JPP = 45 Hz, PCl2). 13C{1H} NMR (75.5 MHz, CDCl3): δ 22.2 (s, CH3), 83.0 (dt, 1JCP = 279 Hz, 3JCP = 7.6 Hz, C≡C(p-Tol)), 102.8 (dt, 2JCP = 54 Hz, 4JCP ~ 2 Hz, C≡C(p-Tol)), 116.4 (d, 3JCP = 5.3 Hz, ArC-ipso), 129.7 (s, ArC-meta), 133.1 (d, 4JCP = 2.0 Hz, ArC-ortho), 142.3 (s, ArC-para). IR (KBr-pellet): 2179 (s, νC≡C), 1604 (w), 1508 (w), 1228 (s, νNP), 1196 (s, νNP), 874 (m), 816 (w), 611 (m), 565 (m), 519 (m), 463 (m), 424 (m) cm–1. HRMS (ESI): m/z calcd for C18H14Cl4N3P3Na: 527.9047, found: 527.9046 [M+Na]+. Anal. Calcd (%) for C18H14Cl4N3P3: C, 42.63; H, 2.78; N, 8.29; Cl, 27.97. Found: C, 42.59; H, 2.97; N, 8.18; Cl, 27.66.
Data for 1b: 1H NMR (300 MHz, CDCl3): δ 2.35 (s, 3H, CH3), 7.11 (d, 2H, 3JHH = 8.0 Hz, ArH), 7.45 (d, 2H, 3JHH = 8.0 Hz, ArH). 31P{1H} NMR (121.5 MHz, CDCl3): δ –31.9 (d, 2JPP = 41 Hz, P{C≡C(p-Tol)}2), 18.9 (t, 2JPP = 41 Hz, PCl2). 13C{1H} NMR (75.5 MHz, CDCl3): δ 21.7 (s, CH3), 84.4 (ddd, 1JCP = 275 Hz, 3JCP = 7.8, 0.9 Hz, C≡C(p-Tol)), 99.9–101.3 (m, C≡C(p-Tol)), 116.8 (virtual t, JCP = 2.6 Hz, ArC-ipso), 129.2 (s, ArC-meta), 132.7 (s, ArC-ortho), 141.2 (s, ArC-para). IR (KBr-pellet): 2177 (s, νC≡C), 1604 (w), 1508 (w), 1190 (s, νNP), 1174 (s, νNP), 868 (m), 839 (w), 814 (m), 781 (w), 768 (w), 611 (m), 573 (m), 563 (m), 546 (m), 538 (m), 474 (m), 459 (m) cm–1. HRMS (ESI): m/z calcd for C36H28Cl2N3P3Na: 688.0765, found: 688.0768 [M+Na]+. Anal. Calcd (%) for C36H28Cl2N3P3: C, 64.88; H, 4.23; N, 6.31; Cl,
10.63. Found: C, 64.53; H, 4.53; N, 6.22; Cl, 10.38.
The reaction of (NPCl2)3 with ca. 6 equiv of LiC≡C(p-Tol)
A solution of p-tolylethynyllithium LiC≡C(p-Tol) was prepared by slow addition of n-BuLi (1.57 M in hexane, 14 mL, 22 mmol) to a solution of 4-ethynyltoluene (2.36 g, 20.3 mmol) in THF (18 mL) at 0 °C followed by stirring of the mixture for 1 h. The mixture was allowed to warm to room temperature, and a solution of (NPCl2)3 (1.16 g, 3.34 mmol) in THF (5 mL) was added dropwise to the mixture over 30 min. After stirring for 3.5 h at room temperature, the reaction was quenched by adding methanol (3.5 mL) at 0 °C. Evaporation of volatiles under vacuum gave a dark-brown oil (5.79 g). The residual oil was extracted with toluene (65 mL), and the extract was centrifuged. After the supernatant was concentrated under vacuum, the dark-brown residue (2.96 g) was separated by flash chromatography on alumina (eluent: THF/toluene 1 : 300). Evaporation of the second fraction gave a yellow oil, and then trituration and washing of the solid with hexane yielded a yellow powder containing hexakis(p-tolylethynyl)cyclotriphosphazene N3P3{C≡C(p-Tol)}6 (1c) as a major component (0.020 g). Attempt to isolate pure 1c from the yellow powder was unsuccessful.
Data assignable to 1c in the yellow powder: 1H NMR (300 MHz, CDCl3): δ 2.33 (s, 3 H, CH3), 7.06 (d, 2 H, 3JHH = 8.1 Hz, ArH), 7.42 (d, 2 H, 3JHH = 8.1 Hz, ArH). 31P{1H} NMR (121.5 MHz, CDCl3): δ –31.3 (s, P{C≡C(p-Tol)}2).
Synthesis of N3P3{C2Co2(CO)6(p-Tol)}2Cl4 (2)
A solution of 1a (0.469 g, 0.925 mmol) in toluene (12 mL) was added to a solution of Co2(CO)8 (0.704 g, 2.06 mmol) in toluene (12 mL) with stirring. After stirring the mixture for 40 min at room temperature, volatiles were removed under vacuum. The dark-red residue was dissolved in a mixture of CH2Cl2 (8 mL) and hexane (4 mL). Cooling of the solution at −30 °C afforded dark-red crystals as the first crop (0.747 g, 0.692 mmol). Concentration and cooling of the mother liquor at −30 °C gave the second crop (0.194 g, 0.180 mmol). Total yield: 94%.
Data for 2: 1H NMR (300 MHz, CDCl3): δ 2.31 (s, 3 H, CH3), 7.00 (d, 2 H, 3JHH = 8.0 Hz, ArH), 7.48 (d, 2 H, 3JHH = 8.0 Hz, ArH). 31P{1H} NMR (121.5 MHz, CDCl3): δ 17.0 (d, 2JPP = 8.1 Hz, PCl2), 26.6 (t, 2JPP = 8.1 Hz, P{C2Co2(CO)6(p-Tol)}2). 13C{1H} NMR (75.5 MHz, CDCl3): δ 21.5 (s, Me), 76.8 (dt, 1JCP = 149 Hz, 3JCP ~ 4 Hz, C−C(p-Tol)), 100.6 (s, C−C(p-Tol)), 129.6 (s, ArC), 130.1 (s, ArC), 133.4 (s, ArC), 138.9 (s, ArC), 197.8 (br s, C≡O). IR (KBr-pellet): 2102 (m, νCO), 2094 (m, νCO), 2063 (s, νCO), 2044 (s, νCO), 2027 (s, νCO), 2015 (s, νCO), 2008 (m, νCO), 1576 (w, νC−C), 1491 (w), 1209 (m, νNP), 1186 (m, νNP), 582 (m), 569 (w), 511 (m), 492 (w) cm–1. HRMS (ESI): m/z calcd for C30H14O12Cl4N3P3Co4Na: 1099.5765, found: 1099.5759 [M+Na]+. Anal. Calcd (%) for C30H14O12Cl4N3P3Co4: C, 33.40; H, 1.38; N, 3.89; Cl, 13.14. Found: C, 32.99; H, 1.38; N, 3.80; Cl, 12.89.
Monitoring the reaction of 1b with excess (ca. 5 equiv) Co2(CO)8
An NMR tube with a J. Young valve (5 mm o.d.) was charged with 1b (5 mg, 8 µmol), (C5H5)2Fe (less than 1 mg) as an internal standard, and C6D6 (0.46 mL). After measuring a 1H NMR spectrum of the solution to determine the intensity ratio of 1b to (C5H5)2Fe, the solution was transferred to a Pyrex NMR tube, and then the washing of the J. Young NMR tube with C6D6 (ca. 0.1 mL) was added. Co2(CO)8 (13 mg, 38 µmol) was then added to the Pyrex NMR tube, and the C6D6 (ca. 0.1 mL) washing of the beaker and funnel, which had been used for weighing and transferring Co2(CO)8, was also added. After the Pyrex NMR tube was flame-sealed under vacuum, the mixture was allowed to stand at room temperature for 22 h. During that time, the reaction was monitored by 1H and 31P{1H} NMR spectroscopy, showing the formation of N3P3{C2Co2(CO)6(p-Tol)}3{C≡C(p-Tol)}Cl2 (3) in 88% NMR yield. Then the tube was opened, and the HRMS and IR spectrum of 3 in the C6D6 solution were measured.
Data for 3: 1H NMR (300 MHz, C6D6): δ 1.86 (s, 3 H, CH3), 1.96 (s, 3 H, CH3), 1.98 (s, 3 H, CH3), 2.03 (s, 3 H, CH3), 6.77 (d, 2 H, 3JHH = 8.0 Hz, ArH), 6.85 (d, 2 H, 3JHH = 8.3 Hz, ArH), 6.95 (d, 2 H, 3JHH = 8.3 Hz, ArH), 6.97 (d, 2 H, 3JHH = 8.6 Hz, ArH), 7.57 (d, 2 H, 3JHH = 8.0 Hz, ArH), 7.85 (d, 2 H, 3JHH = 8.6 Hz, ArH), 7.88 (d, 2 H, 3JHH = 8.3 Hz, ArH), 7.93 (d, 2 H, 3JHH = 8.3 Hz, ArH). 31P{1H} NMR (121.5 MHz, C6D6): δ 1.1 (dd, 2JPP = 6.1 Hz, 2JPP = 12.6 Hz, P{C2Co2(CO)6(p-Tol)}{C≡C(p-Tol)}), 17.7 (t, 2JPP = 12.6 Hz, PCl2), 24.1 (dd, 2JPP = 6.1 Hz, 2JPP = 12.6 Hz, P{C2Co2(CO)6(p-Tol)}2). IR (C6D6): 2100 (w, νCO), 2092 (w, νCO), 2069 (s, νCO), 2033 (s, νCO) cm–1. HRMS (ESI): m/z calcd for C54H28O18Cl2N3P3Co6Na: 1545.5842, found: 1545.5847 [M+Na]+.
X-Ray crystal structure determination
X-Ray quality single crystals of 1a were obtained by recrystallization from toluene as colorless blocks and those of 2 from CH2Cl2/hexane as dark-red blocks. Intensity data for the analysis were collected on a Rigaku Saturn CCD diffractometer with graphite-monochromated Mo Kα radiation (λ = 0.71070 Å) under a cold nitrogen stream (T = 173 K). Numerical absorption corrections were applied to the data. The structures were solved by direct method using the SHELXS-97 program21 for 1a or the Patterson method using the DIRDIF-99 program22 for 2 and refined by full matrix least-squares techniques on all F2 data with SHELXL-97.21 Anisotropic refinement was applied to all non-hydrogen atoms, and all the hydrogen atoms were put at calculated positions. CCDC-700217 (1a) and 700218 (2) contain the supplementary crystallographic data for this paper. These data can be obtained free of charge from The Cambridge Crystallographic Data Centre via www.ccdc.cam.ac.uk/data_request/cif.
Crystallographic data for 1a: C18H14Cl4N3P3, M = 507.03, monoclinic, space group P21/n (no. 14), a = 10.623(3) Å, b = 17.038(5) Å, c = 12.194(4) Å, β = 90.4320(13)°, V = 2207.0(12) Å3, Z = 4, Dcalc = 1.526 g cm−3, µ(Mo−Kα) = 0.76 mm−1, 15524 reflections measured, 4783 unique (Rint = 0.040), R1 = 0.044 and wR2 = 0.082 (all data).
Crystallographic data for 2: C30H14Cl4Co4N3O12P3, M = 1078.87, triclinic, space group P-1 (no. 2), a = 9.050(3) Å, b = 11.436(4) Å, c = 20.122(6) Å, α = 74.483(8)°, β = 79.287(9)°, γ = 88.179(10)°, V = 1971.4(11) Å3, Z = 2, Dcalc = 1.818 g cm−3, µ(Mo−Kα) = 2.11 mm−1, 14183 reflections measured, 8395 unique (Rint = 0.030), R1 = 0.036 and wR2 = 0.066 (all data).
ACKNOWLEDGEMENTS
This work was supported by 2007 Research Grants from the Science and Technology Foundation of Japan (JSTF).
† Dedication to Professor Emeritus Keiichiro Fukumoto on the occasion of his 75th birthday
References
1. V. Chandrasekhar, P. Thilagar, and B. M. Pandian, Coord. Chem. Rev., 2007, 251, 1045, and references cited therein.
2. T. Chivers, Inorg. Nucl. Chem. Lett., 1971, 7, 827.
3. H. R. Allcock, P. J. Harris, and R. A. Nissan, J. Am. Chem. Soc., 1981, 103, 2256.
4. C. W. Allen, J. L. Desorcie, and K. Ramachandran, J. Chem. Soc., Dalton Trans., 1984, 2843.
5. C. W. Allen and M. Bahadur, Phosphorus, Sulfur, and Silicon, 1993, 76, 203.
6. H. R. Allcock, R. A. Nissan, P. J. Harris, and R. R. Whittle, Organometallics, 1984, 3, 432.
7. C. W. Allen, P. Malik, A. Bridges, J. Desorcie, and B. Pellon, Phosphorus, Sulfur, and Silicon, 1990, 49-50, 433; C. W. Allen and M. Bahadur, J. Inorg. Organomet. Polymers, 1998, 8, 23.
8. M. Bahadur, C. W. Allen, W. E. Geiger, and A. Bridges, Can. J. Chem., 2002, 80, 1393.
9. M. S. Kumar, S. Upreti, and A. J. Elias, Inorg. Chem., 2006, 45, 7835; M. S. Kumar, R. P. Gupta, and A. J. Elias, Inorg. Chem., 2008, 47, 3433.
10. R. Schmutzler, Inorg. Synth., 1967, 9, 76.
11. The reaction of (NPCl2)3 with sodium acetylide was investigated by Burg and Caron, but the product was thought to be polymeric and the detailed structure was not elucidated; A. B. Burg and A. P. Caron, J. Am. Chem. Soc., 1959, 81, 836.
12. C. W. Allen, Ind. Eng. Chem. Prod. Res. Dev., 1981, 20, 77; H. R. Allcock, J. L. Desorcie, and G. H. Riding, Polyhedron, 1987, 6, 119; P. J. Harris and C. L. Fadeley, Inorg. Chem., 1983, 22, 561.
13. Unreacted (NPCl2)3 was observed in the 31P{1H} NMR of the reaction mixture.
14. W. C. Still, M. Kahn, and A. Mitra, J. Org. Chem., 1978, 43, 2923.
15. C. W. Allen, Chem. Rev., 1991, 91, 119.
16. C. W. Allen, Coord. Chem. Rev., 1994, 130, 137.
17. G. J. Bullen, J. Chem. Soc. A, 1971, 1450.
18. H. R. Allcock, M. S. Connolly, and R. R. Whittle, Organometallics, 1983, 2, 1514.
19. H. R. Allcock, D. J. Brennan, J. M. Graaskamp, and M. Parvez, Organometallics, 1986, 5, 2434; H. R. Allcock, D. J. Brennan, B. S. Dunn, and M. Parvez, Inorg. Chem., 1988, 27, 3226; N. V. Mani, F. R. Ahmed, and W. H. Barnes, Acta Cryst., 1965, 19, 693.
20. A similar NMR-scale reaction between 1b and Co2(CO)8 in 1 : 3 molar ratio gave hexanuclear cluster 3 as a major product in a lower NMR yield (62%).
21. G. M. Sheldrick, SHELXS-97, Programs for Solving X-ray Crystal Structures, University of Göttingen, Göttingen, Germany, 1997; G. M. Sheldrick, SHELXL-97, Programs for Refining X-ray Crystal Structures, University of Göttingen, Göttingen, Germany, 1997.
22. P. T. Beurskens, G. Beurskens, R. de Gelder, S. Garcia-Granda, R. O. Gould, R. Israel, and J. M. M. Smits, The DIRDIF-99 program system, Crystallography Laboratory, University of Nijmegen, The Netherlands, 1999.