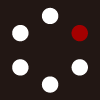
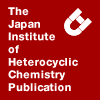
HETEROCYCLES
An International Journal for Reviews and Communications in Heterocyclic ChemistryWeb Edition ISSN: 1881-0942
Published online by The Japan Institute of Heterocyclic Chemistry
e-Journal
Full Text HTML
Received, 6th August, 2008, Accepted, 18th September, 2008, Published online, 22nd September, 2008.
DOI: 10.3987/COM-08-S(F)100
■ Synthesis of 2-Methylene-tetrahydropyran-3-ol Derivative, a Key Segment for Convergent Synthesis of Polycyclic Ethers Based on Suzuki-Miyaura Coupling
Tomohiro Kimura, Yasuyo Miyagawa, Shingo Ozawa, Mayumi Hagiwara, and Tadashi Nakata*
Department of Chemistry, Faculty of Science, Tokyo University of Science, Kagurazaka 1-3, Shinjuku-ku, Tokyo 162-8601, Japan
Abstract
2-Methylene-tetrahydropyran-3-ol derivative, a key segment for convergent synthesis of polycyclic ethers based on Suzuki-Miyaura coupling, was efficiently synthesized by two methods; (1) via SmI2-induced reductive cyclization of β-alkoxyvinyl sulfone with aldehyde followed by elimination of the sulfonyl group, and (2) via SmI2-induced cyclization of β-alkoxyvinyl sulfoxide with aldehyde followed by dehydration of the 2-hydroxymethyl group.Since brevetoxin B was isolated as a red tide toxin, many marine polycyclic ethers have been reported.1 These natural products have a characteristic trans-fused polycyclic ether ring system and exhibit potent biological activities, such as neurotoxicity, cytotoxicity, and antiviral and antifungal activities. The skeletal complexity and biological activities of these marine polycyclic ethers have attracted much attention among chemists and biochemists. Thus, numerous synthetic chemists have extensively studied the development of new synthetic methods for polycyclic ether and the total synthesis of these natural products.2 Several efficient convergent strategies have been developed as tools for the total synthesis of marine polycyclic ethers.2h Sasaki and coworkers reported an efficient convergent strategy based on Suzuki-Miyaura coupling,3 and successfully applied it to the total synthesis of gambierol,4 gymnocin-A,5 and brevenal.6 In their strategy, two cyclic ether segments, exo-methylene ether (i) and enol triflate (iii), are used as coupling partners leading toward polycyclic ether (Figure 1). After hydroboration of i with 9-BBN, the resulting 2-boryl derivative (ii) was efficiently coupled with iii under Suzuki-Miyaura coupling conditions to give the combined ether (iv), which was converted into polycyclic ether (vi) via acetal (v). The 2-methylenetetrahydropyran-3-ol derivative (i), a key coupling partner for this convergent synthesis, has usually been synthesized from 2-iodomethyl-tetrahydropyran (vii).
Recently, we have developed synthetic methods for cyclic ethers by utilizing SmI2-induced cyclization of β-alkoxyvinyl sulfone with aldehyde7 and β-alkoxyvinyl sulfoxide with aldehyde,8 affording 2,6-syn-2,3-trans-tetrahydropyrans having 2-phenylsulfonylmethyl and 2-tolylsulfoxymethyl groups, respectively. We now report the synthesis of the 2-methylene-tetrahydropyran-3-ol derivative (i) starting from these products.
First, we examined the synthesis of 2-methylenetetrahydropyran-3-ol (3) from 2-phenylsulfonylmethyltetrahydropyran-3-ol (2),7 prepared by SmI2-induced cyclization of β-alkoxyvinyl sulfone (1) (Scheme 1). Swern oxidation of the alcohol (2) afforded ketone (4) in quantitative yield. Then, elimination of the phenylsulfonyl group to give enone (5) was attempted by means of base treatment. Treatment of 4 with DBU in CH2Cl2 at 0 °C afforded dimeric compound (6) (45% yield), which would be produced via enone (5).9 Several reaction conditions were examined; elimination of the sulfonyl group proceeded at –15 °C, while the dimerization did not take place at this temperature, but started at –5 °C.
On the basis of these results, we attempted one-pot synthesis of the desired exo-methylene (3) by elimination of the phenylsulfonyl group of 4 followed by Luche reduction10 of the resulting enone (5) (Scheme 2). Upon treatment of the ketone (4) with DBU in MeOH at –15 ~ –5 °C, elimination took place to give enone (5), which was immediately reduced with NaBH4 at –78 ~ 0 °C in the presence of CeCl3·7H2O to give the desired exo-methylene compound (3) in 78% yield. The configuration of the hydroxy group in 3 was determined to be equatorial based on the value of the 1H NMR coupling constant between Ha and methylene protons (J = 10.4, 5.8 Hz) of the corresponding acetate (7), prepared by acetylation of 3. Protection of 3 with TBDMSCl and imidazole afforded TBDMS ether (8) in 78% yield, which should be useful for the convergent synthesis of polycyclic ethers.
As an application of this method, we synthesized the 2-methylenetetrahydropyran (16), a key segment in Sasaki’s total synthesis of gymnocin-A,5 in which 16 was synthesized starting from 2-deoxy-D-ribose in fifteen steps with 38% overall yield.5b,11 First, we examined a route via SmI2-induced cyclization of β-alkoxyvinyl sulfone with aldehyde (Scheme 3). Treatment of the known thioacetal (9),12 prepared from 2-deoxy-D-ribose by thioacetalization and benzylidenation (78% yield), with LHMDS and (E)-bis(phenylsulfonyl)-1,2-ethylene stereoselectively afforded (E)-β-alkoxyvinyl sulfone (10). Removal of the thioacetal from 10 by treatment with MeI in the presence of CaCO3 in aqueous MeCN13 afforded aldehyde (11) in 78% yield (two steps from 9). Treatment of 11 with SmI2 in the presence of MeOH in THF stereoselectively afforded 2,6-syn-2,3-trans-tetrahydropyran (12) in 98% yield. The stereochemistry of 12 was supported by the value of the 1H NMR coupling constant (ddd, J = 10.2, 10.2, 4.5 Hz; Ha) and NOE of the corresponding acetate (17), prepared by acetylation of 12 (Figure 2). Swern oxidation of the alcohol (12) gave ketone (13) in 87% yield. Treatment of 13 under the same conditions used for 4 (DBU, MeOH) gave unsatisfactory results. After several attempts, treatment of 13 with DBU in THF at –15 °C for 1 h, followed by Luche reduction (NaBH4, CeCl3·7H2O, MeOH) at –78 °C in one pot afforded exo-methylene alcohol (15), which without further purification was treated with chlorotriethylsilane (TESCl) and imidazole in DMF to give the desired exo-methylene TES ether (16)14 in 65% yield (two steps from 13). The spectral data of the synthetic 16 were identical with those reported for authentic 16.5 Thus, a new synthesis of 16 was accomplished in only eight steps from 2-deoxy-D-ribose with 34% overall yield.
Next, we examined an alternative route for the synthesis of 2-methylenetetrahydropyran (16) via SmI2-induced cyclization of β-alkoxyvinyl sulfoxide with aldehyde (Scheme 4). Treatment of 9 with (S)-ethynyl p-tolylsulfoxide in the presence of N-methylmorpholine (NMM) afforded (E)-β-alkoxyvinyl sulfoxide (18) in 95% yield. After removal of the thioacetal from 18 (91% yield), the resulting aldehyde (19) was treated with SmI2 in THF-MeOH to give 2,6-syn-2,3-trans-tetrahydropyran (20) as a single product, which was protected with TESCl to give TES ether (21) in 93% yield (two steps). The stereochemistry of 20 was supported by the value of the 1H NMR coupling constant (ddd, J = 10.4, 10.4, 4.8 Hz; Ha) and NOE measurement of the corresponding acetate (23) (Figure 2). Pummerer rearrangement of the sulfoxide (21) with TFAA afforded aldehyde, which was reduced with NaBH4 in one pot to give alcohol (22)15 in 79% yield. The alcohol (22) was previously converted into exo-methylene (16) in two steps (1. I2, Ph3P, imidazole, 2. t-BuOK) in 84% yield.5 Thus, an alternative synthesis of 16 was accomplished in nine steps from 2-deoxy-D-ribose with 42% overall yield.
We have developed new synthetic routes for 2-methylenetetrahydropyran-3-ol derivative, which is a key segment for convergent synthesis of marine polycyclic ethers based on Suzuki-Miyaura coupling. In most total syntheses of polycyclic ethers based on Suzuki-Miyaura coupling,4-6 the starting compounds contain cyclic ether having a 2-hydroxymethyl group, which is transformed to exo-methylene at the final stage. In the present method, the exo-methylene cyclic ether ring can be synthesized at the final stage by SmI2-induced cyclization followed by elimination. Thus, this method provides another synthetic route to the coupling partner, exo-methylene cyclic ether, in the convergent synthesis.
ACKNOWLEDGEMENTS
This work was financially supported in part by the NOVARTIS Foundation (Japan) for the Promotion of Science and by a Grant-in-Aid for Scientific Research on Priority Areas from the Ministry of Education, Culture, Sports, Science and Technology of Japan.
† Dedicated to Professor Emeritus Keiichiro Fukumoto on the occasion of his 75th birthday.
References
1. For reviews on polycyclic ethers, see: (a) T. Yasumoto and M. Murata, Chem. Rev., 1993, 93, 1897; CrossRef (b) Y. Shimizu, Chem. Rev., 1993, 93, 1685; CrossRef (c) M. Murata and T. Yasumoto, Nat. Prod. Rep., 2000, 17, 293; CrossRef (d) T. Yasumoto, Chem. Rec., 2001, 1, 228; CrossRef (e) A. H. Deranas, M. Norte, and J. J. Fernández, Toxicon, 2001, 39, 1101. CrossRef
2. For reviews, see: (a) E. Alvarez, M.-L. Candenas, R. Pérez, J. Ravelo, and J. D. Martín, Chem. Rev., 1995, 95, 1953; CrossRef (b) K. Fujiwara, N. Hayashi, T. Tokiwano, and A. Murai, Heterocycles, 1999, 50, 561; CrossRef (c) Y. Mori, Chem. Eur. J., 1997, 3, 849; CrossRef (d) F. P. Marmsäter and F. G. West, Chem. Eur. J., 2002, 8, 4347; CrossRef (e) M. Inoue, Org. Biomol. Chem., 2004, 2, 1811; CrossRef (f) K. Fujiwara and A. Murai, Bull. Chem. Soc. Jpn., 2004, 77, 2129; CrossRef (g) T. Nakata, Chem. Rev., 2005, 105, 4314; CrossRef (h) M. Inoue, Chem. Rev., 2005, 105, 4379. CrossRef
3. (a) M. Sasaki, H. Fuwa, M. Inoue, and K. Tachibana, Tetrahedron Lett., 1998, 39, 9027; CrossRef (b) M. Sasaki, H. Fuwa, M. Ishikawa, and K. Tachibana, Org. Lett., 1999, 1, 1075; CrossRef (c) H. Fuwa, M. Sasaki, and K. Tachibana, Tetrahedron, 2001, 57, 3019; CrossRef (d) H. Fuwa, M. Sasaki, and K. Tachibana, Org. Lett., 2001, 3, 3549; CrossRef (e) M. Sasaki, M. Ishikawa, H. Fuwa, and K. Tachibana, Tetrahedron, 2002, 58, 1889; CrossRef (f) H. Takakura, M. Sasaki, S. Honda, and K. Tachibana, Org. Lett., 2002, 4, 2771. CrossRef
4. (a) H. Fuwa, M. Sasaki, M. Satake, and K. Tachibana, Org. Lett., 2002, 4, 2981; CrossRef (b) H. Fuwa, N. Kainuma, K. Tachibana, and M. Sasaki, J. Am. Chem. Soc., 2002, 124, 14983. CrossRef
5. (a) C. Tsukano and M. Sasaki, J. Am. Chem. Soc., 2003, 125, 14294; CrossRef (b) C. Tsukano, M. Ebine, and M. Sasaki, J. Am. Chem. Soc., 2005, 127, 4326. CrossRef
6. (a) H. Fuwa, M. Ebine, and M. Sasaki, J. Am. Chem. Soc., 2006, 128, 9648; CrossRef (b) H. Fuwa, M. Ebine, A. J. Bourdelais, D. G. Baden, and M. Sasaki, J. Am. Chem. Soc., 2006, 128, 16989. CrossRef
7. T. Kimura and T. Nakata, Tetrahedron Lett., 2007, 48, 43. CrossRef
8. T. Kimura, M. Hagiwara, and T. Nakata, Tetrahedron Lett., 2007, 48, 9171. CrossRef
9. The same type of dimerization of monocyclic enone was reported. O. R. Martin and F. Xie, Carbohydr. Res., 1994, 264, 141. CrossRef
10. J. L. Luche, J. Am. Chem. Soc., 1978, 100, 2226. CrossRef
11. K. C. Nicolaou, P. A. Wallace, S. Shi, M. A. Ouellette, M. E. Bunnage, J. L. Gunzner, K. A. Agrios, G.-Q. Shi, P. Gärtner, and Z. Yang, Chem. Eur. J., 1999, 5, 618. CrossRef
12. (a) M. Morita, T. Haketa, H. Koshino, and T. Nakata, Org. Lett., 2008, 10, 1679; CrossRef (b) K. Krohn and G. Börner, J. Org. Chem., 1991, 56, 6038. CrossRef
13. (a) M. Fetizon and M. Jurion, J. Chem. Soc., Chem. Commun., 1972, 382; CrossRef (b) S. Takano, S. Hatakeyama, and K. Ogasawara, J. Chem. Soc., Chem. Commun., 1977, 68; CrossRef (c) D. J. Edmonds, K. W. Muir, and D. J. Procter, J. Org. Chem., 2003, 68, 3190. CrossRef
14. Data for synthetic 16: [α]D24 +43.1 (c 1.01, C6H6); IR (film) 2956, 2877, 1661, 1216, 1095, 1028 cm-1; 1H NMR (500 MHz, C6D6) δ 7.62-7.60 (m, 2H), 7.19-7.10 (m, 3H), 5.33 (s, 1H), 4.88 (s, 1H), 4.80 (s, 1H), 4.24 (dd, J = 9.5, 4.0 Hz, 1H), 4.19 (dd, J = 10.4, 5.5 Hz, 1H), 3.56 (ddd, J = 11.3, 10.4, 5.8 Hz, 1H), 3.52 (dd, J = 9.8, 9.8 Hz, 1H), 3.36 (ddd, J = 11.9, 9.5, 4.3 Hz, 1H), 2.38 (ddd, J = 11.3, 5.5, 5.5 Hz, 1H), 1.90 (ddd, J = 11.3, 11.3, 11.3 Hz, 1H), 0.97 (t, J = 8.0 Hz, 9H), 0.57 (q, J = 8.0 Hz, 6H); 13C NMR (125 MHz, C6D6) δ 161.7, 138.3, 129.0, 128.2 (2C), 126.7 (2C), 101.7, 93.3, 76.1, 74.3, 69.3, 67.3, 39.6, 7.0 (3C), 5.1 (3C); HRMS (ESI) calcd for C20H30O4SiNa [(M+Na)+] 385.1806, found 385.1806.
15. Data for synthetic 22: [α]D24 -48.0 (c 0.93, CHCl3); IR (film) 3495, 2955, 2876, 1092 cm-1; 1H NMR (500 MHz, CDCl3) δ 7.50-7.48 (m, 2H), 7.37-7.34 (m, 3H), 5.52 (s, 1H), 4.33 (dd, J = 10.5, 5.0 Hz, 1H), 3.87 (ddd, J = 11.5, 5.5, 3.0 Hz, 1H), 3.76 (ddd, J = 11.0, 9.5, 4.5 Hz, 1H), 3.68 (m, 1H), 3.68 (dd, J = 10.5, 10.5 Hz, 1H), 3.52 (ddd, J = 11.0, 9.0, 4.0 Hz, 1H), 3.43 (ddd, J = 10.0, 10.0, 4.5 Hz, 1H), 3.35 (ddd, J = 8.5, 5.5, 3.0 Hz, 1H), 2.43 (ddd, J = 11.5, 4.5, 4.5 Hz, 1H), 1.91 (dd, J = 6.0, 6.0 Hz, 1H), 1.76 (ddd, J = 11.5, 11.5, 11.5 Hz, 1H), 0.96 (t, J = 8.0 Hz, 9H), 0.63 (q, J = 7.5 Hz, 6H); 13C NMR (125 MHz, CDCl3) δ 137.3, 129.1, 128.3 (2C), 126.1 (2C), 101.7, 82.3, 76.4, 72.9, 69.2, 66.5, 62.3, 38.6, 6.7 (3C), 4.9 (3C); HRMS (ESI) calcd for C20H32O5SiNa [(M+Na)+] 403.1911, found 403.1893