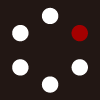
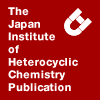
HETEROCYCLES
An International Journal for Reviews and Communications in Heterocyclic ChemistryWeb Edition ISSN: 1881-0942
Published online by The Japan Institute of Heterocyclic Chemistry
e-Journal
Full Text HTML
Received, 16th September, 2008, Accepted, 15th October, 2008, Published online, 23rd October, 2008.
DOI: 10.3987/COM-08-S(D)22
■ Stereoselective Synthesis of 5-Substituted 2-Allyl-3-oxotetrahydrofuran-2-carboxylates Using Rhodium(II)-Catalyzed Oxonium Ylide Formation–[2,3] Shift
Takayuki Yakura,* Katsuaki Matsui, Kazumasa Matsuzaka, and Masayuki Yamashita
Faculty of Pharmaceutical Sciences, Graduate School, Toyama University, 2630 Sugitani, Toyama, Toyama 930-0194, Japan
Abstract
Reaction of 5-allyloxy-2-diazo-3-ketoesters 1 with catalytic amount of dirhodium(II) tetraacetate in dichloromethane proceeded in high yields with excellent stereoselectivities to give methyl 5-substituted 2-allyl-3-oxotetrahydro- furan-2-carboxylates 2, which are suitable intermediates for synthesis of heliespirones and their derivatives.Heliespirones A,1,2 B,2,3 and C2 were isolated from leaves of Helianthus annuus L. in 1998 and 2006 as members of a new family of bioactive sesquiterpenes, potential allelopathic agents. These compounds display unusual previously unknown spirosesquiterpene skeletons. Heliespirones A and C have a 2,2,3,5-tetrasubstituted tetrahydrofuran framework; heliespirone B has a 2,2,3,6-tetrasubstituted tetrahydropyran framework. Stereoselective construction of substituted tetrahydrofurans and pyrans is an important subject for synthesis of these attractive sesquiterpenes and their analogues. Herein, we describe the synthesis of 5-substituted 2-allyl-3-oxotetrahydrofuran-2-carboxylates 2, which might become suitable intermediates for the synthesis of heliespirones, in high yields with excellent stereoselectivities by rhodium(II)-catalyzed oxonium ylide formation-[2,3] shift of α-diazo-β-ketoesters 1.
Metal-catalyzed carbenoid reactions4 have become a powerful tool for synthesis of functionalized cyclic compounds including oxacycles. However, it is sometimes difficult to control their chemoselectivity because of the high reactivity of the metal carbenoid intermediates, which can react with heteroatoms, inactivated C–H bonds, C–C multiple bonds, etc. Moreover, the bulkiness of metal carbenoid influences its chemoselectivity.5,6 Formation of cyclic allylic oxonium ylide by intramolecular reaction of diazocarbonyl compound containing suitably positioned allylic ethereal oxygen atom and its subsequent [2,3] shift offer an effective approach to the stereoselective construction of substituted cyclic ethers. In contrast to the metal-catalyzed oxonium ylide formation-rearrangement reactions of diazoketones,7,8 those of α-diazo-β-ketoester have been little investigated.9 Although the Pirrung group9a and West group9e reported, respectively, oxonium ylide formation-[2,3] shift of simple α-diazo-β-ketoester, methyl 5-allyloxy-2-diazo-3-ketopentanoate, using dirhodium(II) tetraacetate and copper(II) hexafluoroacetylacetonate [Cu(hfacac)2] to give 2-allyl-3-oxotetrahydrofuran-2-carboxylate in high yield (R=H, Scheme 1), no information related to chemoselectivity and diastereoselectivity of similar reactions of substituted α-diazo-β-ketoesters is available. Therefore we envisioned investigation of metal-catalyzed reactions of substituted α-diazo-β-ketoesters (1, R≠H) to 2,2,5-trisubstituted 3-oxotetrahydrofurans (2 or 3, R≠H), which are expected to provide a feasible method for synthesis of the 2,2,3,5-tetrasubstituted tetrahydrofuran framework of heliespirones A and C, as outlined in Scheme 1.
Starting 5-allyloxy-2-diazo-3-ketoesters 1 were prepared from the corresponding aldehyde in three steps: reaction with diketene in the presence of titanium tetrachloride then treatment with methanol,5,10 followed by allylation, subsequent diazotransfer reaction with p-toluenesulfonyl azide (Scheme 2).
First we tested the reaction of phenyl substituted α-diazo-β-ketoester 1a lacking C–H bonds at the C-6 position that can react with generated metal carbenoid (Scheme 3). Reaction of 1a with 3 mol% of Rh2(OAc)4 in dichloromethane9e at rt for 30 min proceeded stereoselectively to give separable tetrahydrofurans 2a and 3a in 94% yield and with a high level of diastereocontrol (2a:3a=91:9) (Table 1, entry 1). Another possible product 4 via Stevens [1,2] shift was not detected. A similar reaction of 1a with Cu(hfacac)2 in CH2Cl29e required higher temperature (reflux) and longer time (4 days) to afford a mixture of 2a and 3a in 94% yield with a slightly lower diastereoselectivity (79:21) (Table 1, entry 2). The trans relation between the phenyl group at C-5 position and migrated allyl group at C-2 position of 2a was determined after the reduction with diisobutylaluminum hydride (DIBAL-H) to diols 5 and 6.11 In the NOESY spectrum of 5, positive NOEs were observed among H-3 proton and H-5 proton, and allylic methylene protons at C-2 position. As an alternative stereoselective synthesis of 2a, we attempted allylation of 5-phenyl-3-oxotetrahydrofuran-2-carboxylate 7.12 The unstable 7 was treated with allyl bromide in the presence of potassium carbonate as a base in acetonitrile12a to give 2a as a major product. This procedure, however, was unsuitable for synthesis of 2a because of low yield (39% for a mixture of 2a and 3a) and low stereoselectivity (2.3:1).
Because Rh2(OAc)4 showed better results than copper catalyst did, we next investigated reactions using other solvents (Table 1, entries 3 and 4) and other rhodium(II) catalysts (Table 1, entries 5–9). Unfortunately, better results than that of entry 1 were not obtained. Interestingly, rhodium catalysts having bulky ligands such as pivaloate and triphenylacetate decreased the stereoselectivities (entries 8 and 9).
Reactions of several α-diazo-β-ketoesters 1b–1h with 3 mol% of Rh2(OAc)4 in CH2Cl2 were investigated as standard conditions.13 Table 2 presents the results. All reactions were completed within 30 min. Reaction of 1b (R = p-Tol) produced almost identical results as that of 1a (Entry 1). Alkyl substituted derivatives 1c–1g, which have reactive C–H bonds at the C-6 position, reacted chemoselectively at the ether oxygen to give the corresponding tetrahydrofurans in high yields with excellent diastereoselectivities (Entries 2–6). Reaction of 1h having bulky tert-butyl group at the C-5 position decreased the chemical yield because of steric hindrance around the ethereal oxygen atom (Entry 7). The yield was increased when 1h reacted with Rh2(OCOC7H15)4 in stead of Rh2(OAc)4 (Entry 8).
A possible rationalization of the observed stereoselectivity in rhodium(II)-catalyzed reaction of 1 is based on the consideration of the conformations of oxonium ylides A and B,7k,7m,8 generated through rhodium-bound ylide 87e (Scheme 4).14 In conformer A, both the allyl group and the substituent at C-5 position can be accommodated in pseudoequatorial positions. In contrast, in conformer B, severe steric repulsion between these two groups becomes evident. We assumed, therefore, that the oxonium ylide formation-[2,3] shift would proceed via the sterically favored conformer A to form tetrahydrofuran 2.
In conclusion, rhodium(II)-catalyzed reaction of 5-allyloxy-2-diazo-3-ketoesters 1 gave methyl 5-substituted 2-allyl-3-oxotetrahydrofuran-2-carboxylates 2 in high yields with excellent stereoselectivities. Synthetic studies on the heliespirones using this methodology are now in progress.
References
1. F. A. Macias, R. M. Varela, A. Torres, and J. M. G. Molinillo, Tetrahedron Lett., 1998, 39, 427. CrossRef
2. F. A. Macias, J. L. G. Galindo, R. M. Varela, A. Torres, J. M. G. Molinillo, and F. R. Fronczek, Org. Lett., 2006, 8, 4513. CrossRef
3. F. A. Macias and F. R. Fronczek, Acta Cryst., 2007, E63, o2104.
4. M. P. Doyle, M. A. McKervey, and T. Ye, 'Modern Catalytic Methods for Organic Synthesis with Diazo Compounds,', John Wiley & Sons, New York, 1998; CrossRef For a recent review, see: C. A. Merlic and A. L. Zechman, Synthesis, 2003, 1137. CrossRef
5. Treatment of methyl 5-tert-butyldimethylsilyloxy-2-diazo-3-oxoheptanoate with Rh(II) catalyst selectively afforded the C–H insertion product. See, T. Yakura, S. Yamada, A. Ueki, and M. Ikeda, Synlett, 1997, 185; CrossRef T. Yakura, S. Yamada, Y. Kunimune, A. Ueki, and M. Ikeda, J. Chem. Soc., Perkin Trans. 1, 1997, 3643. CrossRef
6. Chemoselectivity (C–H insertion reaction vs. oxonium ylide formation) in Rh(II)-catalyzed reaction of 5,6-dioxygenated 2-diazo-3-oxohexanoates was controlled by their O-protecting groups. See, T. Yakura, A. Ueki, Y. Morioka, T. Kurata, K. Tanaka, and M. Ikeda, Chem. Pharm. Bull., 1997, 46, 1182; T. Yakura, A. Ueki, T. Kitamura, K. Tanaka, M. Nameki, and M. Ikeda, Tetrahedron, 1999, 55, 7461. CrossRef
7. (a) E. J. Roskamp and C. R. Johnson, J. Am. Chem. Soc., 1986, 108, 6062; CrossRef (b) J. S. Clark, Tetrahedron Lett., 1992, 33, 6193; CrossRef (c) J. S. Clark, S. A. Krowiak, and L. J. Street, Tetrahedron Lett., 1993, 34, 4385; CrossRef (d) J. S. Clark and G. A. Whitlock, Tetrahedron Lett., 1994, 35, 6381; CrossRef (e) J. S. Clark, A. G. Dossetter, and W. G. Whittingham, Tetrahedron Lett., 1996, 37, 5605; CrossRef (f) J. S. Clark, M. Fretwell, G. A. Whitlock, C. J. Burns, and D. N. A. Fox, Tetrahedron Lett., 1998, 39, 97; CrossRef (g) J. S. Clark and Y.-S. Wong, Chem. Commun., 2000, 1079; CrossRef (h) J. S. Clark, A. L. Bate, and T. Grinter, Chem. Commun., 2001, 459; CrossRef (i) F. P. Marmsäter and F. G. West, J. Am. Chem. Soc., 2001, 123, 5144; CrossRef (j) F. P. Marmsäter, J. A. Vanecko, and F. G. West, Tetrahedron, 2002, 58, 2027; CrossRef (k) J. S. Clark, G. A. Whitlock, S. Jiang, and N. Onyia, Chem. Commun., 2003, 2578; CrossRef (l) F. P. Marmsäter, J. A. Vanecko, and F. G. West, Org. Lett., 2004, 6, 1657; CrossRef (m) J. S. Clark, T. C. Fessard, and C. Wilson, Org. Lett., 2004, 6, 1773; CrossRef (n) J. S. Clark, T. C. Fessard, and G. A. Whitlock, Tetrahedron, 2006, 62, 73; CrossRef (o) J. S. Clark, C. Guerot, C. Wilson, and A. J. Blake, Chem. Commun., 2007, 4134. CrossRef
8. T. Yakura, W. Muramatsu, and J. Uenishi, Chem. Pharm. Bull., 2005, 53, 989. CrossRef
9. (a) M. C. Pirrung and J. A. Werner, J. Am. Chem. Soc., 1986, 108, 6060; CrossRef (b) M. C. Pirrung, W. L. Brown, S. Rege, and P. Laughton, J. Am. Chem. Soc., 1991, 113, 8561; CrossRef (c) N. McCarthy, M. A. McKervey, T. Ye, M. McCann, E. Murphy, and M. P. Doyle, Tetrahedron Lett., 1992, 33, 5983; CrossRef (d) N. Pierson, C. Fernández-García, and M. A. McKervey, Tetrahedron Lett., 1997, 38, 4705; CrossRef (e) G. K. Murphy and F. G. West, Org. Lett., 2006, 8, 4359. CrossRef
10. T. Izawa and T. Mukaiyama, Chem. Lett., 1975, 161. CrossRef
11. Treatment of 5 with 2,2-dimethoxypropane in the presence of camphorsulfonic acid (CSA) gave the corresponding acetonide within 10 min, although a similar reaction of 6 did not proceed after 24 h.
12. (a) M. A. Calter, P. M. Sugathapala, and C. Zhu, Tetrahedron Lett., 1997, 38, 3837; CrossRef (b) M. A. Calter and C. Zhu, J. Org. Chem., 1999, 64, 1415. CrossRef
13. Typical reaction procedure: A solution of 1 (0.2 mmol) and Rh2(OAc)4 (0.006 mmol) in CH2Cl2 (20 mL) was stirred at rt. After 1 was consumed completely, as indicated by TLC, the mixture was concentrated and purified using column chromatography on silica gel to give pure 2. All new compounds gave satisfactory spectroscopic data.
14. Alternatively, the reaction may proceed through stepwise allyl-transfer to rhodium metal followed by reductive elimination, in analogy to that proposed in the case of [1,2] shift of benzyl group by Dhavale group15.
15. N. P. Karche, S. M. Jachak, and D. D. Dhavale, J. Org. Chem., 2001, 66, 6323. CrossRef