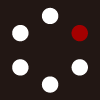
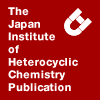
HETEROCYCLES
An International Journal for Reviews and Communications in Heterocyclic ChemistryWeb Edition ISSN: 1881-0942
Published online by The Japan Institute of Heterocyclic Chemistry
e-Journal
Full Text HTML
Received, 15th September, 2008, Accepted, 27th October, 2008, Published online, 28th October, 2008.
DOI: 10.3987/COM-08-S(F)116
■ New [11C]Phosgene Based Synthesis of [11C]Pyrimidines for Positron Emission Tomography
Koh-ichi Seki,* Ken-ichi Nishijima, Kimihito Sanoki, Yuji Kuge, Masayuki Takahashi, Hiromichi Akizawa, Nagara Tamaki, Leonard I. Wiebe, and Kazue Ohkura*
Central Institute of Radio Isotope Science, Hokkaido University, Kita 15 Nishi 7, Kita-ku, Sapporo, Hokkaido 060-0815, Japan
Abstract
Thymine, 5-FU, and uracil were successfully synthesized through a procedure involving a cyclocondensation of triphosgene with newly developed α-substituted β-aminoacrylamides intermediates (1a, X = Me; 1b, X = F; 1c, X = H). The radioligands [2-11C]thymine and [2-11C]5-fluorouracil were synthesized in high radiochemical yields in 16-17 minutes from the end of bombardment by applying the cyclocondensation method with [11C]COCl2.INTRODUCTION
Thymidine phosphorylase (TP; (EC 2.4.2.4)) is an important enzyme which catalyses reversible deoxyribosylation of thymine (Thy) to thymidine (thymidine + phosphate ⇌ thymine + 2-deoxy-α-D-ribose 1-phosphate). 5-Substituted uracils (5-XUra) including uracil (Ura) can also be substrates for TP. TP is also known to activate 5-fluorouracil (5-FU) to 5-fluoro-2’deoxyribonucleoside, which acts as TP inhibitor.1 Furthermore, it is reported that TP is associated with angiogenesis as a growth factor, and its expression is strongly associated with the growth of tumors.2 Thus, TP is an attractive target for imaging and therapy, 3 and many pyrimidine-based radiopharmaceuticals including thymine have been developed for clinical diagnosis in the field of single photon computed tomography (SPECT) or positron emission tomography (PET).4 In 1991, Vander Borght et al. synthesized [2-11C]thymine via cyclocondensation of diethyl β-methyl malate with [11C]urea.5 Although other investigators have attempted to improve this method or develop methodologies involving condensation of a malate intermediate with the labeling agent [11C]urea derived from phosgene,6 cyanide7 or carbon dioxide as the key ring closure reactions,8 those condensation reactions were carried out under conditions as drastic as those employed for 14C labeled thymine synthesis.9 The complexity of the currently available synthetic routes along with the extended length of preparation time has limited the extensive applicability of 11C-labeled nucleosides in PET studies.
5-FU, which was originally synthesized in 195710,11 as one of a new class of antitumor fluoroprymidines, has been also an attractive target as a possible PET ligand. 5-FU-mediated inhibition of thymidylate synthetase 12 was subsequently shown to be one of the major mechanisms responsible for the antitumor activity of these compounds. Today, almost 50 years later, 5-FU remains front-line therapy, alone or in combination with other drugs or radiation, for gastric,13,14 colorectal,15,16 and other cancers including advanced pancreatic cancer.17 Development of a diagnostic PET tracer based on 5-FU would be very important to assess or predict more successful outcomes in selecting drugs for cancer chemotherapy. Indeed, it has been demonstrated, for example, that tumor uptake of fluorine-18 labeled 5-FU (5-[18F]FU) serves a positive prognostic role in selection of patients for 5-FU therapy (Strauss 5-[18F]FU test).18,19 Underutilization of the ‘Strauss 5-[18F]FU test’ may be due, in part, to the proposed need for complex kinetic modeling rather than simple tumor uptake,20 and/ or to the electrophilic F-18 radiosynthetic method developed in the early 1970’s.21 The latter method remains the sole method of 5-[18F]FU radiosynthesis today, and is thus not popular in units using the 18O(p, n)18F nuclear reaction on H218O to produce aqueous radiofluoride for routine clinical radiofluorinations.
Thus, there are still clinical needs to develop efficient PET tracers directed towards TP for evaluating the grade of malignancy,22 the proliferative activity of tumor cells,23 and the outcome of cancer chemotherapy.
Meanwhile, we have recently developed a highly efficient synthesis of [11C]COCl2 with high specific activity.24 This method has been successfully applied to producing [11C]CGP-12177, a PET tracer for β-adrenoreceptors which is now supplied for clinical use.25 Application of this [11C]COCl2 to the synthesis of [11C]pyrimidines would provide a potential procedure for tumor targeting novel PET tracers.
These contexts prompted us to develop a facile and efficient synthesis of [11C]COCl2-based [11C]5-XUra (X = Me, F, H) by developing a synthetic route to the common precursors, β-aminoacrylamide derivatives (1a-c) with substituents such as Me (a), F (b), H (c) at the α-position, that is to be subjected to the cyclization with [11C]COCl2 to form versatile [2-11C]pyrimidine derivatives in the last step (Scheme 1). We report herein a novel and facile synthesis of [2-11C]thymine and [2-11C]5-fluorouracil through direct condensation of [11C]COCl2 with the key intermediates β-aminoacrylamides (1a-b).
RESULTS AND DISCUSSION
Synthesis of thymine (Thy). The key intermediate, β-aminomethacrylamide (1a), was readily synthesized from ethyl α-formylpropionate (2a).26 Treatment of 2a with ammonia afforded diastereomixture of β-aminomethacrylate (3aZ and 3aE). The Z -isomer 3aZ was benzoylated with benzoyl chloride to give N-benzoylacrylate 4aZ with the desired stereochemistry (Z-form). Treatment of the resulting 4aZ with ammonia exclusively afforded the desired intermediate (Z)-β-(N-benzoylamino)methacrylamide (5aZ) with the stereochemistry maintained in the desired Z-form (Scheme 2). Hydrolysis of the benzoylated compound (5aZ) failed to give β-aminomethacrylamide (1a). Therefore, 5aZ was used as a key intermediate for the subsequent ring closure with triphosgene, to Thy. For the synthesis of non-radio-labeled (cold) Thy, triphosgene was used as a safe and stable replacement for phosgene.
The key intermediate 5aZ was converted to the alkali metal salts (5aZ-Na or 5aZ-K) with a base. Addition of triphosgene to the resulting salt under various conditions gave rise to the formation of benzoylthymine (6a), which was readily hydrolyzed with NH3, or by passing through a short column of silica gel, to give Thy (Scheme 3).27
As summarized in Table 1, the best result was obtained when the reaction was performed with the sodium salt (5aZ-Na) in DMF.
Synthesis of 5-fluorouracil (5-FU). The key intermediate β-(N-benzoylamino)-α-fluoroacrylamide (5bE) was synthesized according to the procedure for 5aZ. Sodium ethyl 2-fluoro-3-hydoxyacrylate (2b), derived from ethyl formate and ethyl fluoroacetate in the presence of sodium methoxide28 was treated with ammonia and ammonium chloride in methanol to give β-aminoacrylate (3b).29 Benzoylation of the resulting 3b with benzoyl chloride in pyridine afforded (Z)-ethyl β-benzoylamino-α-fluoroacrylate (4bZ), wherein the ethoxycarbonyl group and the benzoylamino group occupy the undesired trans-stereochemistry on the ethylene moiety for the subsequent cyclization with phosgene. In order to effect geometric isomerization of the Z-isomer 4bZ into the E-isomer 4bE, UV-irradiation of 4bZ with a high-pressure mercury lamp afforded the equilibrium mixture of 4bZ and 4bE in the ratio of 1 : 9. The desired E-isomer 4bE was further treated with ammonia to furnish the key intermediate 5bE with the desired stereochemistry (E-form) in quantitative yield (Scheme 4).
The sodium salt of the key intermediate 5bE was subjected to cyclocondensation with triphosgene at room temperature, followed by hydrolysis with ammonia in methanol, resulting in the formation of 5-FU in high yield (75%, after purification on HPLC) (Scheme 5).
Synthesis of uracil (Ura). Ethyl formylacetate (2c), prepared from Meldrum’s acid (2,2-dimethyl- 1,3-dioxane-4,6-dione) and ethyl formate was treated with ammonia to afford β-aminoacrylate (3c), albeit in an undesired (E)-stereochemistry, which was benzoylated with benzoyl chloride to give benzoyl aminoacrylate 4cE. Treatment of the resulting 4cE with ammonia afforded benzoyliminopropanamide, (5cI), instead of giving either the desired Z-β-(N-benzoylamino)acrylamide (5cZ) or E-β-(N-benzoylamino)acrylamide (5cE). In order to obtain a key intermediate 5cZ in the desired Z-stereochemisitry, E-β-(N-benzoylamino)acrylate (4cE) was irradiated with a 500 W high-pressure mercury lamp to give the stereoisomer 4cZ. Treatment of the resulting ester (4cZ) with ammonia, however,
failed to give the desired 5cZ, but afforded the imino tautomer (5cI), which is identical with that obtained from 4cE via ammonolysis (Scheme 6). The resulting imine (5cI) is the tautomeric isomer of either 5cE or 5cZ, and hence the sodium or potassium salts, if formed sufficiently, can be inter-convertible through the tautomerism. Therefore, we decided to use 5cI for the subsequent cyclocondensation with triphosgene.
Thus, imine 5cI was converted to its sodium salts (5cI-Na) with sodium hydride, and resulting 5cI-Na was treated with triphosgene at room temperature for 5 min, to furnish the desired Ura, albeit in poor yield (2.4%) (Scheme 7). The formation of Ura was confirmed by comparison of the spectroscopic data and chromatographic behaviors with those of the authentic sample.
[11C] COCl2 based synthesis of [11C]pyrimidines as potential tumor targeting PET tracers. As described above, pyrimidine rings were formed by the cycloaddition of the developed N-benzoyl aminoacrylamide intermediates and triphosgene in short reaction times, suggesting that the present reaction would provide a versatile method for the synthesis of pyrimidine based PET tracers. Then, we carried out the synthesis of [2-11C]Thy and [2-11C]5-FU, which could serve clinically as useful PET tracers for the evaluation of the cell proliferation ([2-11C]Thy) and for the assessment and prediction of outcomes of 5-FU in chemotherapeutic treatment ([2-11C]5-FU).
Synthesis of Synthesis of [2-11C]Thy. 11C-labeled thymine was readily prepared in a similar manner as described above, using the same automated synthesis system as used for [11C]CGP-12177 production.25 The direct ring closure reaction of [11C]COCl2 with non-activated precursor (5aZ) only restored the starting material 5aZ. Therefore the precursor (5aZ) was treated with a base to convert it to the alkali metal salt (5aZ-Na 5aZ-K), into which [11C] COCl2 gas was introduced to give [2-11C]Thy (Scheme 8).
The yield of [2-11C]Thy under these conditions was 362 ± 53 MBq at EOS (n=3) (Table 2). The radiochemical yield of [2-11C]thymine was ca. 24% from [11C]COCl2.30 [2-11C]Thymine produced was identical with authentic thymine by comparison of their chromatographic behavior on HPLC. The formation of [2-11C]thymine was further confirmed by the enzymatic conversion to [2-11C]thymidine.6, 31 Thus, the present cyclocondensation of an appropriate activated N-benzoyl aminoacrylamide intermediate with highly reactive radiolabeling reagent [11C]COCl2 is a viable method for supplying [2-11C]Thy and [2-11C]thymidine for clinical PET tracer studies.
Synthesis of [2-11C]5-FU. The key intermediate 5bE, activated as the sodium salt, was subjected to cyclocondensation with [11C]COCl229 on the same automated synthesis system used for the production of S-[11C]CGP-12177.25 The total synthesis took 17 minutes from the end of bombardment (EOB) to isolation of [2-11C]5-FU. The yield of [2-11C]5-FU was 380 MBq at EOS (Scheme 9), for a radiochemical yield of ca. 25%.
In all previous reports, labeling of the 2-position of thymine was accomplished by condensation of [11C]urea and malate at 130 °C in fuming sulfuric acid. Recently, Steel et al reported an improved method for the preparation of [2-11C]Thy via a multi-step process using [11C]urea derived from [11C]COCl2. This radiosynthesis of [2-11C]thymine took approximately 30 min from EOB.6 On the other hand, our strategy involving the cyclocondensation with [11C]COCl2 for the direct production of [2-11C]thymine is operationally simple, and offers fewer reaction steps at lower temperature. The total synthesis described herein takes 16 minutes from EOB to isolation of [2-11C]Thy, thus significantly shortening reaction time, which is a crucial consideration for the preparation of short half-life radiopharmaceuticals. The success in the synthesis lies in the synthesis of the key precursor 5aZ bearing proper stereochemistry, and on the application of the highly reactive species, [11C]COCl2, for cyclocondensation in the final step.
Synthesis of [2-11C]5-FU proceeded in the same way, resulting in the comparable radiochemical yields with those of [2-11C]thymine using [11C]COCl2.29 Importantly, the radiochemical yields are adequate for in vivo studies of [2-11C]5-FU uptake in patients, and would appear sufficient for analysis of 1-h time-activity curves32 using the catenary, three-compartment, five-parameter model developed for 5-[18F]FU in vivo.20
We have provided a substantially more useful method for the synthesis of [2-11C]Thy and [2-11C]5-FU. Because of fewer reaction steps, mild reaction conditions, and reliability of product yield, the present methodology should find wide application in the preparation of many 11C labeled radiopharmaceuticals.
EXPERIMENTAL
Materials and Analyses
Triphosgene was purchased from Aldrich Chemical Co. Ltd. (St. Louis, MO). All solvents were reagent grade and distilled using the appropriate methods. Column chromatography was performed using silica gel 60N (100-210 μm) and Aluminiumoxid 90 active neutral (70-230) Mesh ASTM, 0.063-0.200 mm, purchased from Merck. Silica gel HPLC was conducted on a Shim-Pack PREP-Sil (H) (250 mm x 20 mm i.d., Silca gel) using a LC-6A (Shimadzu, Kyoto, Japan) apparatus with monitoring at 254 nm. All melting points are uncorrected. NMR spectra were measured with a JEOL JNM-EA500 (500 MHz) spectrometer, and 1H-NMR chemical shift are given on the δ (ppm) scale based on those of the signals of solvents. MS spectra and high-resolution MS (HRMS) spectra were recorded with JEOL JMS-FABmate (EI). The elemental analyses (C, H, N) were within ± 0.4% of the theoretical values for C, H and N. UV-Irradiation was carried out externally with a 500 W high-pressure mercury (h.p. Hg) lamp (Eiko-sha, Osaka) in a degassed Pyrex tube (> 300 nm) on a merry-go-round apparatus.
Synthesis of Thy
Ethyl α-formylpropionate (2a). A solution of ethyl formate (10.4 mL, 130 mmol) and ethyl propionate (7.5 mL, 65 mmol) in dry Et2O (80 mL) were added to a suspension of NaH (ca. 1.7 g, ca. 70 mmol) at 0 °C. The reaction mixture was stirred at ambient temperature for 60 h, and then neutralized with aqueous hydrochloric acid. The reaction mixture was extracted three times with CH2Cl2. After drying over anhydrous Na2SO4, the solvent was removed under atmospheric pressure to give 2a (3.1 g, 37%) as oil.
Ethyl β-aminomethacrylate (3aZ, 3aE). To an ethereal solution (5 mL) of 2a (3.1g, 24 mmol) was added 7M methanolic ammonia (9 mL, 63 mmol). After heating under reflux for 2 h, the solvent was removed under reduced pressure, to give a mixture of 3aZ and 3aE (3.0 g, 98%) as oil. The Z-isomer 3aZ gradually isomerized itself into 3aE, to afford a mixture of 3aZ and 3aE (1 : 1) when kept at ambient temperature for 21 h. Thus, the isolation of 3aE was not achieved.
(Z)-Ethyl β-aminomethacrylate (3aZ): H-NMR (CDCl3) δ: 1.25 (3H, t, J =6.9 Hz), 1.68 (3H, s), 4.03 (2H, br s), 4.14 (2H, q, J =7.1 Hz), 7.43 (1H, t, J =10.3 Hz). EI-MS m/z: 129 [M]+. EI-HRMS m/z: 129.0795 (Calcd for C6H11NO2: 129.0790).
(E)-Ethyl β-aminomethacrylate (3aE): 1H-NMR (CDCl3) δ: 1.25 (3H, t, J =6.9 Hz), 1.70 (3H, s), 4.03 (2H, br s), 4.13 (2H, q, J =6.9 Hz), 6.64 (1H, t, J =11.2 Hz).
Ethyl β-(N-benzoylamino)methacrylate (4aZ, 4aE). A mixture of 3aZ and 3aE (260 mg, 2 mmol) dissolved in CHCl3 (10 mL) was added to a solution of pyridine (320 µL, 0.4 mmol) and benzoyl chloride (235 µL, 2 mmol) in CHCl3 (20 mL) at 0 °C and kept overnight at rt. After removal of the solvent, 10% hydrochloric acid was added to the residual oil and extracted with Et2O. After drying over anhydrous Na2SO4, the ethereal layer was subjected to silica-gel column chromatography with 10% AcOEt-hexane, to afford 4aZ (240 mg, 35%).
(Z)-Ethyl β-(N-benzoylamino)methacrylate (4aZ): Colorless crystals, mp 61-62 °C, recrystalized from AcOEt. 1H-NMR (CDCl3) δ: 1.34 (3H, t, J =6.9 Hz), 1.90 (3H, d, J =1.2), 4.26 (2H, q, J =7.0 Hz), 7.48 (2H, t, J =7.4 Hz), 7.55 (1H, t, J =7.5 Hz), 7.62 (1H, dd, J =1.1, 10.9 Hz), 7.93 (2H, d, J =6.9 Hz), 11.4 (1H, d, J =9.8 Hz). EI-MS m/z: 233 [M]+. EI-HRMS m/z: 233.1053 (Calcd for C13H15NO3: 233.1052).
(E)-Ethyl β-(N-benzoylamino)methacrylate (4aE): 1H-NMR (CDCl3) δ: 1.32 (3H, t, J =7.5 Hz), 2.00 (3H, s), 4.24 (2H, q, J =7.2 Hz), 7.47 (1H, m), 7.50 (2H, t, J =7.5 Hz), 7.64 (1H, t, J =7.5 Hz), 8.13 (2H, d, J =8.0 Hz), 8.45 (1H, d, J =1.2 Hz).
(Z)-β-(N-Benzoylamino)methacrylamide (5aZ). A solution of 4aZ in MeOH was added to an excess of liquid ammonia and allowed to stand at rt for a week. The reaction mixture was subjected to column chromatography over alumina with 50% AcOEt-hexane, to give 5aZ as colorless crystals. The 1H-NMR measurement showed the reaction proceeded quantitatively. Colorless crystals, mp 182-184 °C (recrystallized from 50% AcOEt-Hexane). 1H-NMR (CDCl3) δ: 1.95 (3H, d, J =1.2 Hz), 5.40-5.80 (2H, br d, NH2), 7.46 (2H, t, J =7.2 Hz), 7.54 (1H, t, J =7.5 Hz), 7.54 (1H, d, J =7.5 Hz), 7.93 (2H, d, J =6.9 Hz), 12.3 (1H, br s). EI-LRMS m/z: 204 [M]+. EI-HRMS m/z: 204.0895 (Calcd for C11H12N2O2: 204.0899). Anal. Calcd for C11H12N2O2: C, 64.69; H, 5.92; N, 13.72. Found: C, 64.50; H, 6.05; N, 13.60.
Non-radio-labeled (cold) Thy. To a solution of 5aZ-Na (100.4 mg, 0.5 mmol) freshly prepared from NaH in DMF (8 mL), triphosgene (25 mg, 0.08 mmol) dissolved in THF (2.5 mL) was added, and stirred for 5 min. Then MeOH was added to the reaction mixture and the solvent was removed under reduced pressure. The residue was dissolved in water and extracted with CHCl3. Aqueous NaOH (10%) was added to the chloroform layer. After neutralization with 10% hydrochlolic acid, the aqueous solution was submitted to reverse-phase HPLC with 6% aq. EtOH (3 mL/min), to give Thy quantitatively (32.4 mg).
Synthesis of 5-FU
N-[(1E)-2-carbamoyl-2-fluorovinyl]benzamide (5bE). A solution of ethyl formate (2.2 mL, 27.5 mmol) and ethyl fluoroacetate (2 mL) in dry Et2O (80 mL) was added to a solution of sodium ethoxide (1.4 g, 20 mmol) in benzene (25 mL) at 0 °C. The reaction mixture was stirred overnight at ambient temperature. The solvent was evaporated under reduced pressure to give crude 2b as oil. Crude 2b (0.64 mmol, 100 mg) was dissolved in NH4Cl saturated MeOH and 2 M NH3-MeOH and stirred for 3 days at rt. After removal of the solvent, the residue was passed through a short column of alumina by using Et2O as the eluent to give 3b (69 mg, 0.6 mmol). To a solution of 3b thus obtained in Et2O, benzoyl chloride (70 µL, 0.6 mmol) and dry pyridine (48.5 µL, 0.6 mmol) were added at -10 °C. The reaction mixture was allowed to stand overnight at 0 °C, and subjected to HPLC with 10% AcOEt in hexane, to afford 4bZ-ethyl ester (0.06 mmol, 14.8 mg) and 4bZ-methyl ester (0.08 mmol, 18.4 mg), respectively.
Ethyl (2Z)-2-fluoro-3-(phenylcarbonylamino)prop-2-enoate (4bZ-ethyl ester): Colorless crystals, mp 101-102 °C (recrystallized from 20% AcOEt in hexane ). 1H-NMR (C6D6) δ: 0.83 (3H, t, J =7.45 Hz, CH3), 3.88 (2H, q, J =7.45 Hz, CH2), 6.86 (2H, aromatic), 6.99 (1H, aromatic), 7.35 (2H, aromatic), 7.62 (1H, br s, NH), 8.03 (1H, dd, J=11.45 Hz, JH-F =25.75 Hz, CH). EI-LRMS m/z: 237 [M]+. EI-HRMS m/z: 237.0799 (Calcd for C12H12NO3: 237.0801). Anal. Calcd for C12H12NO3: C, 60.76; H, 5.10; N, 5.90. Found: C, 60.80; H, 5.10; N, 5.89.
Methyl (2Z)-2-fluoro-3-(phenylcarbonylamino)prop-2-enoate (4bZ-methyl ester): Colorless crystals, mp 142-143 °C (recrystallized from 20% AcOEt in hexane ). 1H-NMR (C6D6) δ: 3.27 (3H, s, CH3), 6.84 (2H, aromatic), 6.97 (1H, aromatic), 7.26 (2H, aromatic), 7.31 (1H, br s, NH), 7.97(1H, dd, J=11.45 Hz, JH-F =25.75 Hz, CH). EI-LRMS m/z: 223 [M]+. EI-HRMS m/z: 223.0647 (Calcd for C11H10NO3: 223.0644). Anal. Calcd for C11H10NO3: C, 59.19; H, 4.52; N, 6.28. Found: C, 59.44; H, 4.59; N, 6.16.
Photochemical isomerization of 4bZ ester. A solution of 4bZ-ethyl ester (50 mg) in MeCN (10 mL) was irradiated externally in a Pyrex tube at rt for 4 h. The reaction mixture was concentrated in vacuo, and the residue was submitted to HPLC with 30% AcOEt in hexane to give the E-form 4bE-ethyl ester and unchanged 4bZ-ethyl ester in 10% and 90% yields, respectively. Similar results were obtained from 4bZ-methyl ester.
Ethyl (2E)-2-fluoro-3-(phenylcarbonylamino)-2-propenoate (4bE-ethyl ester): Colorless crystals, mp 91-92 °C (recrystallized from 20% AcOEt-hexane ). 1H-NMR (C6D6) δ: 0.83 (3H, t, J =7.45 Hz, CH3), 3.84 (2H, q, J =7.45 Hz, CH2), 6.89 (2H, aromatic), 6.95 (1H, aromatic), 7.79 (2H, aromatic), 7.86 (1H, dd, JH-F, JH-H =11.45 Hz, CH), 10.2 (1H, br s, D2O exchangeable, NH). EI-LRMS m/z: 237 [M]+. EI-HRMS m/z: 237.0792 (Calcd for C12H12NO3: 237.0801). Anal. Calcd for C12H12NO3: C, 60.76; H, 5.10; N, 5.90. Found: C, 60.56; H, 5.23; N, 5.87.
Methyl (2E)-2-fluoro-3-(phenylcarbonylamino)prop-2-enoate (4bE-methyl ester): Colorless crystals, mp 80-81 °C (recrystallized from 20 % AcOEt-hexane ). 1H-NMR (C6D6) δ: 3.19 (3H, s, CH3), 6.89 (2H, aromatic), 6.94 (1H, aromatic) 7.79 (2H, aromatic), 7.84 (1H, dd, JH-F, JH-H =11.45 Hz, CH), 10.16 (1H, br s, D2O exchangeable, NH). EI-LRMS m/z: 223 [M]+. EI-HRMS m/z: 223.0642 (Calcd for C11H10NO3: 223.0644). Anal. Calcd for C11H10NO3: C, 59.19; H, 4.52; N, 6.28. Found: C, 59.29; H, 4.54; N, 6.25.
N-[(1E)-2-carbamoyl-2-fluorovinyl]benzamide (5bE). A solution of 4bE (10 mg, 0.037 mmol) and excess liq. ammonia in MeOH (1 mL) was allowed to stand in a high-pressure reaction vessel for 4 days at rt. After evaporation of the solvent in vacuo, the reaction mixture gave N-[(1E)-2-carbamoyl- 2-fluorovinyl]benzamide (5bE) in quantitative yield. 5bE: Colorless crystals, mp 160-161 °C (recrystallized from 20% AcOEt-hexane). 1H-NMR (CDCl3) δ: 5.68, 6.10 (1H, br s, D2O exchangeable, NH2), 7.49 (2H, aromatic), 7.57 (1H, aromatic), 7.79(1H, dd, JH-F, JH-H =10.85 Hz, CH), 7.91 (2H, aromatic), 10.8 (1H, br s, D2O exchangeable, NH). EI-LRMS m/z: 208 [M]+. EI-HRMS m/z: 208.0639 (Calcd for C10H9N2O2: 208.0648). Anal. Calcd for C10H9N2O2: C, 57.69; H, 4.36; N, 13.46. Found: C, 57.65; H, 4.52; N, 13.34.
Non-radio-labeled (cold) 5-FU. A solution of the sodium salts of 5bE, prepared from 5bE (20.0 mg, 0.1 mmol) and NaH, and triphosgene (33 mg, 0.1 mmol, 3 eq) in DME (5mL) was stirred at rt for 16 h. After addition of 2 M NH3-MeOH (2 mL), the reaction mixture was condenced under reduced pressure. The residue was submitted to reverse-phase HPLC with 3% MeOH in water (1 mL/min) to give 5-FU in 75 % yield.
Synthesis of uracil (Ura)
Ethyl N-benzoylaminoacrylate (4cE). Ethyl formylacetate (2c) was prepared by refluxing a mixture of Meldrum’s acid (480 mg, 2.5 mmol) and EtOH (1.2 equv. molar) in benzene for 90 min, according to the reported procedure.33 A solution of crude 2c (107 mg, 0.92 mmol, 37%), thus prepared, and liquid ammonia in MeOH (2.0 mL) was allowed to stand for a week at rt, to give 3c. To a solution of crude 3c (69 mg, 0.6 mmol) and pyridine (48.5 µL, 0.6 mmol) in Et2O (2 mL), benzoyl chloride (70 µL, 0.6 mmol) dissolved in CHCl3 (20 mL) was added dropwise at -10 °C, and the reaction mixture was kept overnight at -10 °C. After removal of the solvent, 10% hydrochloric acid was added to the residual oil and extracted with ether. After drying over anhydrous Na2SO4, the ethereal layer was subjected to silica-gel column chromatography with 10% AcOEt-hexane as an eluent, to afford ethyl N-benzoylaminoacrylate (4cE) (14.8 mg, 0.068 mmol, 0.3%). 1H-NMR (CDCl3) δ: 1.27 (3H, t, J =6.9 Hz), 4.17 (2H, q, J =6.9 Hz), 5.64 (1H, d, J =14.3 Hz), 7.45 (2H, t, J =7.5 Hz), 7.55 (1H, t, J =7.5 Hz), 7.85 (1H, d, J = 7.5 Hz), 8.22 (1H, dd, J =11.5, 14.3 Hz), 8.67 (1H, d, J =11.5 Hz, -NH). EI-MS m/z: 219 [M]+.
Photochemical isomerization of E-form 4cE-ethyl ester to Z-form. A solution of E-form 4cE (50 mg) in MeCN (10 mL) in a Pyrex tube was irradiated externally at rt for 4 h. The reaction mixture was concentrated in vacuo, and submitted to HPLC with 30% AcOEt in hexane, to give 4cZ in the desired-Z-form in 30% yield, together with unchanged 4cE in 70% yield.
Ethyl N-benzoylaminoacrylate (4cZ): 1H-NMR (CDCl3) δ: 1.27 (3H, t, J =6.9 Hz), 4.17 (2H, q, J =6.9 Hz), 5.64 (1H, d, J =14.3 Hz), 7.45 (2H, t, J =7.5 Hz), 7.55 (1H, t, J =7.5 Hz), 7.85 (1H, d, J = 7.5 Hz), 8.22 (1H, dd, J =11.5, 14.3 Hz), 8.67 (1H, d, J =11.5 Hz, -NH). EI-MS m/z: 219 [M]+.
N-[(1E)-2-carbamoylvinyl]benzamide 5cI. A solution of 4cZ (10 mg, 0.046 mmol) and excess liq. ammonia in MeOH (2.0 mL) was kept at rt for 4 days. After evaporation of the solvent in vacuo, the residue was submitted to column chromatography on alumina with AcOEt – hexane (1 : 1) to give 5cI in the yield of 90% (7.5 mg, 0.040 mmol) as colorless crystals.
Similarly 4cE afforded 5cI in 90 % yield. 1H-NMR (CD3OD) δ: 2.66 (2H, d, J =5.7 Hz), 5.15 (1H, bs or t, J =5.7 Hz), 7.45 (2H, t, J =7.5 Hz), 7.52 (1H, t, J =7.5 Hz), 7.80 (1H, d, J = 7.5 Hz),. EI-MS m/z: 190 [M]+.
Non-radio-labeled (cold) Ura. A solution of 5cI (19.0 mg, 0.1 mmol) and NaH (21.6 mg, 60% oil, 0.5 mmol) in DME was kept at rt for 4 h. Triphosgene (33 mg, 0.1 mmol, 3 eq) was added to the solution, which was allowed to stand overnight at rt. Then, 7 M ammonia in MeOH (2 mL) was added to the solution, and the reaction mixture was kept for 2 min at rt. Removal of the solvent under reduced pressure gave Ura in 2.4% (0.3 mg, 0.0024 mmol) yield.
Synthesis of 2-[11C]Thymine
According to the procedure adopted for non-radio-labeled (cold) Thy, [11C]phosgene was bubbled with helium carrier into a solution of 5az-Na (0.2 mg) in DME (500 µL) for 1 min at 30 °C . After removal of the solvent by evaporation, the residue consisting of [2-11C]N-benzoylthymine (6a) was treated with 1.5 M methanolic ammonia for 1 min at rt, and subjected to reverse-phase HPLC (column; μ-Bondapak C18, 25 cm × 0.39 cm i.d., solvent; 3% EtOH–saline, flow rate; 0.5 mL/min at 40 °C), equipped with a UV monitor (detected at 254 nm) and a γ counter. The radioactive peak at 11 min was identified as the desired thymine. Radiochemical purity of [2-11C]thymine was estimated to be 99% by HPLC.
Synthesis of [2-11C]5-FU
According to the procedure for non-radio-labeled (cold) 5-FU, [11C]phosgene was infused with helium into a solution of 5bE-Na (0.2 mg) in DME (500 µL) for 1 min at 30 °C. After removal of the solvent by evaporation, the residue consisting of [2-11C]N-benzoyl-5-FU was treated with 1.5 M methanolic ammonia for 1 min at rt, and subjected to reverse-phase HPLC (column; Inertsil ODS-3, 250 mm x 4.6 mm i.d., solvent; 3% EtOH - Saline, flow rate; 0.5 mL/min at 40 °C), equipped with a UV monitor (detected at 270 nm) and a γ counter. The radioactive peak at 9.3 min was identified as authentic cold 5-FU.
ACKNOWLEDGMENTS
This work was supported in part by Grants-in-Aid for Scientific Research and High Technology Research Program from the Ministry of Education, Culture, Sports, Science and Technology of Japan.
This paper is dedicated to Professor Emeritus Keiichiro Fukumoto on the occasion of his 75th birthday.
References
1. C. Cole, A. J. Foster, S. Freeman, M. Jaffar, P. E. Murray, and I. J. Stratford, Anti-cancer Drug Des., 1999, 14, 383.
2. C. T. Bailie, M. C. Winslet, and N. J. Bradley, Br. J. Cancer, 1995, 72, 257; E. J. Battegay, J. Mol. Med., 1995, 73, 333; CrossRef S. Kikuyama, T. Inada, K. Shimizu, and M. Miyakita, Anticancer Res., 2000, 20, 2081.
3. R. W. Klecker Jr. and J. M. Collins, Cancer Chemother. Pharmacol., Cancer Chemother. Pharmacol., 2001, 48, 407.
4. J. Toyohara, and Y. Fujibayashi, Nucl. Med. Biol., 2003, 30, 681, and references cited therein. CrossRef
5. T. Vander Borght, D. Labar, S. Pauwels, and L. Lambotte, Int. J. Appl. Radiat. Isot., 1991, 42, 103. CrossRef
6. C. J. Steel, F. Brady, S. K. Luthra, G. Brown, I. Khan, K. G. Poole,A. Sergis, T. Jones,P. and M. Price, Appl. Radiat. Isot., 1999, 51, 377.
7. J. M. Link, J. R. Grierson, and K. A. Krohn, J. Label. Compd. Radiopharm., 1995, 37, 610.
8. P. K. Chakraborty, T. J. Mangner, and H. T. Chugani¥, Appl. Radiat. Isot., 1997, 48, 619.
9. T. Vander Borght, S. Pauwels, L. Lambotte, C. De Saeger, and C. Beckers, J. Label. Compd. Radiopharm., 1990, 28, 819. CrossRef
10. C. Heidelberger, N. K. Chaudhuri, P. Danneberg, D. Mooren, L. Griesbach, R. Duschinsky, R. J. Schnitzer, E. Pleven, and J. Scheiner, Nature, 1957, 179, 663. CrossRef
11. R. Duschinsky, E. Pleven, and C. Heidelberger, J. Am. Chem. Soc., 1957, 79, 4559. CrossRef
12. K. U. Hartmann and C. Heidelberger, J. Biol. Chem., 1961, 236, 3006.
13. M. Tahara, A. Ohtsu, N. Boku, F. Nagashima, M. Muto, Y. Sano, M. Yoshida, K. Mera, S. Hironaka, H. Tajiri, and S. Yoshida, Gastric Cancer, 2001, 4, 212. CrossRef
14. E. Van Cutsem, V. M. Moiseyenko, S. Tjulandin, A. Majlis, M. Constenla, C. Boni, A. Rodrigues, M. Fodor, Y. Chao, E. Voznyi, M. L. Risse, and J. A. Ajani, J. Clin. Oncol., 2006, 24, 4991. CrossRef
15. S. Patiyil and S. R. Alberts, Curr. Treat. Options. Oncol., 2006, 7, 389. CrossRef
16. S. Goyle and A. Maraveyas, Dig. Surg., 2005, 22, 401. CrossRef
17. J. K. Park, J. K. Ryu, J. K. Lee, W. J. Yoon, S. H. Lee, Y. T. Kim, and Y. B. Yoon, Pancreas, 2006, 33, 397. CrossRef
18. A. Dimitrakopoulou-Strauss, L. G. Strauss, P. Schlag, P. Hohenberger, G. Irngartinger, F. Oberdoorfer, J. Doll, and G. Van Kaick, J. Nucl. Med., 1998, 39, 465.
19. J. Kissel, G. Brix, M. E. Bellemann, L. G. Strauss, A. Dimitrakopoulou-Strauss, R. Port, U. Haberkorn, and W. J. Lorenz, Cancer Res., 1997, 57, 3415.
20. J. R. Bading, P. B. Yoo, J. D. Fissekis, M. M. Alauddin, D. Z. D'Argenio, and P. S. Conti, Cancer Res., 2003, 63, 3667.
21. J. S. Fowler, R. D. Finn, R. M. Lambrecht, and A. P. Wolf, J. Nucl. Med., 1973, 14, 63.
22. A. Perkins, Eur. J. Nucl. Med., 1993, 20, 573. CrossRef
23. D. L. Fraker and J. A. Norton, Gastroenterol. Clin. North Am., 1989, 18, 805.
24. K. Nishijima, Y. Kuge, K. Seki, K. Ohkura, N. Motoki, K. Nagatsu, A. Tanaka, E. Tsukamoto, and N. Tamaki, Nucl. Med. Biol., 2002, 29, 345. CrossRef
25. K. Nishijima, Y. Kuge, K. Seki, K. Ohkura, K. Morita, K. Nakada, and N. Tamaki, Nucl. Med. Commun., 2004, 25, 845. CrossRef
26. J. N. Marx, J. Craig Argyle, and L. R. Norman, J. Am. Chem. Soc., 1974, 96, 2121. CrossRef
27. K. A. Cruickshank, J. Jiricny, and C. B. Reese, Tetrahedron Lett., 1984, 25, 681. CrossRef
28. T. Miyamoto, H. Egawa, and J. Matsumoto, Chem. Pharm. Bull., 1987, 35, 2280.
29. K. Ohkura, K. Nishijima, K. Sanoki, Y. Kuge, N. Tamaki, and K. Seki, Tetrahedron Lett., 2006, 47, 5321. CrossRef
30. The yield of [2-11C]thymine was determined based on produced [11C]COCl2. We estimated the yield of [11C]COCl2 to be about 1500 MBq based on the yield of diphenylurea.
31. M. Friedkin and D. Roberts, J. Biol. Chem., 1954, 207, 257 .
32. D. A. Mankoff, A. F. Shields, M. M. Graham, J. M. Link, J. F. Eary, and K. A. Krohn, J. Nucl. Med., 1998, 39, 1043.
33. M. Sato, N. Yoneda N. Katagiri, H. Watanabe, and C. Kanako, Synth. Commun, 1986, 672.