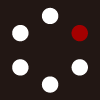
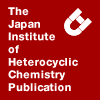
HETEROCYCLES
An International Journal for Reviews and Communications in Heterocyclic ChemistryWeb Edition ISSN: 1881-0942
Published online by The Japan Institute of Heterocyclic Chemistry
e-Journal
Full Text HTML
Received, 9th October, 2008, Accepted, 17th November, 2008, Published online, 19th November, 2008.
DOI: 10.3987/COM-08-11574
■ Concise Synthesis of Xyloketal C Analogues
Yan Huang and Yong-Cheng Lin*
School of Chemistry and Chemical Engineering, Sun Yat-Sen University, Guangzhou 510275, China
Abstract
The synthesis of xyloketal C analogues 12-methylxyloketal C(8) and 12-dehydroxylxyloketal C(11) have been accomplished in three steps, which featured the radical cyclisation as the key step. This is the shortest approach to construct the B C ring systems of the type of ketal skeleton reported to date. The structures of title compounds were elucidated by spectroscopic data and X-ray structure analysis. The final radical cyclisations give mainly the cis-fused anti-substituted stereoisomers.INTRODUCTION
The xyloketals are a group of new natural products, which were isolated from the mangrove fungus Xylaria Sp(#2508) in our lab by Lin and co-workers,1 these new compounds include xyloketals A, B, C, D and E (Figure 1), they are a family of ketal compounds which incorporating identical 5,6-bicyclic acetal moieties fused to a benzene core. The molecular structures and relative stereochemistries of these natural products were determined by extensive spectroscopic studies and X-ray crystallography. The absolute stereochemistries of these natural products were determined by interpretation of their CD spectra.1 Xyloketal A has a unique chiral C3-symmetric molecular structure that incorporates three bicyclic acetal moieties, xyloketal B and the minor C2-symmetric component, xyloketal C, incorporate two bicyclic acetal moieties. Xyloketal A has also been shown to be a potent inhibitor of acetylcholine esterase in a dose-dependent fashion and so it is expected to be an important lead compound for the treatment of neurological diseases. In addition, xyloketals A and B have been shown to have L-calcium channel blocking activity.2 Therefore, an efficient synthetic methodology to xyloketals and their structural analogues, which would allow extensive diversity for biological screening purposes, is highly desirable.
Much effort has been made for the total synthesis of xyloketals and their analogues since the finding of these natural compounds. Krohn and co-workers have reported an asymmetric synthesis of (-)-xyloketal D from (3R)-3-methylbutyrolactone and 2,4-dihydroxyacetophenone as well as the synthesis of racemic xyloketals A and B and their analogues 5-demethylxyloketals by condensation of phloroglucinol with appropriate enone in multistep, one-pot, domino reaction.3,4 Wilson et al. have attempted a racemic synthesis of the two possible diastereoisomers of a tris-demethyl analogue of xyloketal A and a racemic synthesis of a demethyl analogue of xyloketal D, and the asymmetric total synthesis of (-)-xyloketal D and its enantiomer.5 These syntheses featured the cycloaddition reaction of appropriately functionalized 0-quinone methides and dihydrofurans as a key step. However, the further application of this chemistry, to complete the total synthesis of xyloketal A, has not been successful. Recently, Wilson and co-workers have achieved the total synthesis of (-)-xyloketal A and syntheses of xyloketal A, B, C, D and G analogues, these process involved an exceedingly facile and diastereoselective boron trifluoride diethyl etherate-promoted triple electrophilic aromatic substitution reaction that was coupled to three bicyclic acetal formation reactions.6
We have attempted a new route to the synthesis of xyloketals and their analogues, these syntheses featured the radical cyclisation as the key step. Thus, concise syntheses of the structural analogues of the natural product xyloketal C were achieved in three steps. This is the shortest approach to construct the B C ring systems of the type of ketal skeleton reported to date, which required only commercially available reagent and moderate reaction conditions.
RESULTS AND DISCUSSION
The synthesis of 12-methylxyloketal C(8) is outlined in Scheme 1. Treatment of orcinol and methyl vinyl ketone in benzene in the presence of Amberlyst-15® resin at reflux with azeotropic removal of water, afforded enol ether 6. Mechanistically, the two fragments orcinol and methyl vinyl ketone may be combined in a Michael-type reaction. Product 6 was found to be somewhat unstable which decomposed gradually even at -60 oC and so it should be used in subsequent reaction as soon as possible.
Coupling of enol ether 6 with allyl alcohol and N-iodosuccinimide(NIS) in a one-pot procedure at -20 oC afforded the iodo ketal 7. Treatment of iodo ketal 7 with 1-ethylpiperidine hypophosphite(1-EPHP) in refluxing benzene in the presence of a catalytic amount of azoisobutyronitrile (AIBN) efficiently yielded the 12-methylxyloketal C(8a~8d)(51%, combined yield). According to literatures, the radical cyclization can be carried out in water or aqueous ethanol,7,8 we found that the removal of traces of water by azeotropic distillation with benzene before starting the reaction by adding AIBN could raise the yield from 10% to about 50%. Although mixtures of stereoisomers were to be expected for 8, only one homogeneous fraction was separated chromatographically.
8 had the molecular formula of C21H28O4, established by HREIMS[ M+, m/z 334.1982, Δ 0.2 mmu]. The sets of signals in 1H and 13C NMR spectra of 8 is similar to the xyloketal C except for the signal of C5-H that appeared at δ2.53 rather than at 1.89 as in the case of natural product, the chemical shifts are somewhat different from the natural product due to the absence of hydroxyl group in C-12 and the signals were slightly broadened by overlapping. In the 1H-1H COSY no cross-peaks for H-13/H-14 was detected, confirming 8 was linearly fused rather than angularly.
The stereochemical outcome of the radical-cyclisation was complicated. In principle, the two oxygen-containing pyran and furan rings B and C can be connected in a cis or trans fashion. Also, the orientation of these stereogenic centers at the ring-junction positions C-2 and C-6 with respect to the methyl at C-5 is an obvious question. Another question concerns the relative orientation of the stereocenters of rings B and C with respect to the remote centers at rings B’and C’.
Therefore, it was possible that two sets of stereoisomers can be formed for 8: syn,syn(8a,8b) and syn,anti(8c,8d). These stereoisomers were a chromatographically homogeneous mixture. Form the integral of the carbon atoms C-10 and C-10’, a ratio of the isomers syn,syn and syn,anti of approximately 1:1.2 can be deduced.4 According to the stereochemistry of radical-cyclisation reaction,9 ring B and C as well as B’ and C’ are always cis orientated, the methyl group at C-5 (or C-5’) could be cis or trans with respect to the stereogenic centers at the ring junction at C-2 (or C-2’) and C-6 (or C-6’), the ratio of trans-cis is about 7 to 1. In fact, the hydrogen atoms at C-5 (or C-5’) in trans and cis isomers resonated at δ2.53 and δ1.89 respectively, from the integral of these two hydrogen atoms, a ratio of the isomers trans and cis of proximately 6.6:1 can be deduced.
X-Ray single-crystal structure analysis was used to determine the relative stereochemistry of the major product of 12-methylxyloketal C(8). The crystal of the major isomer was obtained on crystallization from ethyl acetate-petroleum ether. The structure was depicted in Figure 2, showing the major syn,anti isomer 8c(Me11, Me10- trans), the ring junction of the pyran and furan ring B and C (at C-2 and C-6) or B’ and C’ (at C-2’ and C-6’) were all cis. The C-5 (or C-5’) methyl group was oriented trans to the respective methyl group at C-2 (or C-2’), that was in agreement with the relative stereochemistry of the radical-cyclisation, however, this stereochemistry was different from naturally occurring xyloketals which show 2,5-cis configuration. Furthermore, it should be noted that the formation of linearly fused compound 8 are rare, as in most case the thermodynamically more stable angularly fused isomers are easier to form than the linearly fused isomers.4
In a similar way to that described previously for the synthesis of 8, the 12-dehydroxylxyloketal C (11) was synthesized using resorcinol and methyl vinyl ketone as starting materials (Scheme 2). 11 had a molecular formula of C20H26O4 as revealed by HREIMS[M+, m/z 330.1824, Δ -0.1 mmu]. That 11 was linearly fused rather than angularly was confirmed by 1H NMR and 1H-1H COSY. The benzene proton appeared as singlets in 1H NMR, and the 1H-1H COSY spectrum revealed no proton connectivities between H-12 and H-13, which established the symmetrical linear structure of 11. The relative stereochemistry of 11 was similar to that of compound 8.
In summary, the synthesis of new xyloketals congeners of 12-methylxyloketal C(8) and 12-dehydroxylxyloketal C(11) have been accomplished in 3 steps from readily available starting materials phenols and methyl vinyl ketone, the relative stereochemistry of major products obtained from the final radical cyclisation is in agreement with the predictions of both Schäfer and Beckwith,9a but different from naturally occurring compounds. The concise and facile synthetic methodology will be useful for the syntheses of diverse xyloketals analogues for biological screening purposes.
EXPERIMENTAL
General methods and instrumentation: NMR spectra were recorded with a Varian INOVA 500NB NMR spectrometer, chemical shifts are referenced to internal TMS(δ =0.00 ppm), mass spectra were determined on VGZAB-HS instrument or MAT95XP spectrometer, and X-ray data on a Bruker Smart 1000 CCD system diffractometer. Dry benzene was distilled from sodium benzophenone ketyl. Dichloromethane(CH2Cl2) was dried over anhydrous CaCl2 and distilled.
2,5,8-Trimethyl-4H,10H-pyrano[2,3-f]chromene (6): A mixture of orcinol (1.69 g, 13.6 mmol), methyl vinyl ketone (Aldrich, 2.48 g, 35.36 mmol), Amberlyst-15 resin (0.6 g) and benzene (20 mL) in a Dean-Stark trap was heated at reflux under argon with azeotropic removal of water for 5 h, the resultant mixture was cooled to rt and filtered, the filtrate was concentrated in vacuo, purification by column chromatography on silica gel using petroleum ether : EtOAc (9:1) as the eluant afforded the title compounds 6 (663 mg, 21.3%) as a white solid. Compound 1. 1H NMR (CDCl3, 300 MHz) δ: 1.86(d, J=1.2Hz, 6H, CH3); 2.16(s, 3H); 3.265(m, 4H, ArCH2); 4.68(m, 2H, ArCH2CH); 6.33(s, 1H, ArH).
Compound 6 was found to be somewhat unstable which decomposed gradually even at -60 oC and so it should be used in subsequent reaction as soon as possible.
2,6-Bis(allyloxy)-3,7-diiodo-2,6,9-trimethyl-3,4,7,8-tetrahydro-2H,8H-pyrano[3,2-f]chromene (7): Under argon atmosphere, the allyl alcohol (479 mg, 8.2 mmol) and ring enol ether 6 (468 mg, 2.05 mmol) in dry CH2Cl2 (10 mL) were added to a suspension of N-iodosuccinimide (969 mg, 4.31 mmol) in dry CH2Cl2 (1 mL) at -20 oC, the reaction mixture was stirred at that temperature for 3 h. It was then filtered, the solvent was removed under reduced pressure and the residue obtained was purified by column chromatography on silica gel using petroleum ether : EtOAc (5:1) as the eluant afforded the title compound 7 (832 mg, 68%) as a viscous oil. 1H NMR (CDCl3, 300 KHz) δ: 1.75(s, 3H); 1.76 (s, 3H); 2.04(s, 3H); 3.10(d, J=4.5, 1H); 3.14(d, J=4.5, 1H); 3.622(t, 1H), 3.683(t, 1H); 4.078-4.157(m, 4H); 4.56(q, 2H); 5.034-5.210(m, 4H); 5.67-5.83(m, 2H); 6.335(s, 1H); 13C NMR (CDCl3, 300 MHz): 14.5, 14.6, 23.2, 23.3, 28.6, 28.9, 33.7, 33.8, 63.9, 64.0, 98.6, 98.7, 103.0, 103.1, 112.3, 116.1, 116.2, 116.3, 134.5, 134.6, 134.7, 134.8, 150.4.
12-Methylxyloketal C (8): A solution of iodo ketal 7 (450 mg, 0.838 mmol), 1-EPHP (3.0 g, 16.76 mmol) and AIBN (20.6 mg, 0.251 mmol) in benzene (10 mL) was heated at reflux, a second portion of AIBN (20.6 mg, 0.251 mmol) being added after reflux for 1 h. Reflux was maintained for 5 h, on cooling, the reaction mixture was diluted with EtOAc (20 mL), the organic layer was washed with hydrochloric acid (2M) and brine, the remaining organic solution was dried over anhydrous magnesium sulfate, filtered and concentrated in vacuo. The residue was purified by column chromatography on silica gel using petroleum ether: EtOAc(3:1) as the eluant to give the title compound 8 (147 mg, 51%) as colorless solid. 1H NMR (CDCl3, 300 MHz) δ: 0.85(d, J=7.2, 6H, H-11, H-11’); 1.52(s, 6H, H-10, H-10’); 2.17(s, 3H, H-14); 2.48(m, 2H, H-6, H-6’); 2.55(m, 2H, H-5, H-5’); 2.72(d, J=6.5, 2H, H-7a, H-7a’); 2.72(d, J=6.5, 2H, H-7b, H-7b’);3.55(dd, J=8.4, 17.0, 2H, H-4b, H-4b’); 4.09(dd, J=7.6, 17.0, 2H, H-4a, H-4a’); 6.31(s, 1H, H-13)ppm; 13C NMR (CDCl3, 300 MHz): δ=13.8,13.6(C-11), 14.5(C-11’), 21.5(C-14), 22.5, 22.6(C-7), 22.7, 22.9(C-7’), 23.5, 24.1 (C-10), 24.5, 25.2(C-10’), 35.6, 35.7(C-5, C-5’), 44.1, 44.2, 44.4(C-6, C-6’), 74.6, (C-4, C-4’), 103.6, 104.2(C-2, C-2’),107.9, 108.0(C-13), 114.4, 114.6(C-8, C-8’), 132.4(C-12), 153.2, 153.3(C-9, C-9’); EIMS m/z: 344 [M]+, 286, 247; HREIMS Found: m/z 344.1984[M]+. C21H28O4. Calculated: M= 344.1982.
2,8-Dimethyl-4H, 10H-[2,3-f]-chromene (9): Compound 9 (0.816 g, 26.6%, a white solid) was prepared from resorcinol and methyl vinyl ketone by the same procedure as described for 6. 1H NMR(CDCl3, 300 MHz) δ: 1.87(d, J=1.2 Hz, 6H, CH3); 3.269(m, 4H, ArCH2); 4.66(m, 2H, ArCH2CH); 6.36(s, 1H, ArH); 6.65(s, 1H, ArH).
Like compound 6, Compound 9 was found to be somewhat unstable which decomposed gradually even at -60 oC and so it should be used in subsequent reaction as soon as possible.
2,8-Bis(allyloxy)-3,7-diiodo-2,8-dimethyl-3,4,7,8-tetrahydro-2H,6H-pyrano[3,2-g]chromene (5): compound 10 (510 mg, 65%, viscous oil) was prepared from 9 by the same procedure as described for 7. 1H NMR (CDCl3, 300 KHz) δ: 1.554(s, 6H); 3.04(t, 2H); 3.84(t, 2H); 4.13(m, 4H); 4.50(m, 2H); 5.03-5.07 (m, 2H); 5.13-5.18(m, 2H); 5.70-5.79 (m, 2H); 6.42(d, J=9.9, 2H); 6.71(d, J=5.1, 1H).
12-Dehydroxylxyloketal C (11): Compound 6 (148 mg, 49%, colorless solid) was prepared from 10 by the same procedure as described for 8. 1H NMR (CDCl3, 300 MHz) δ: 0.90(d, J=6.0, 6H, H-11, H-11’); 1.59(s, 6H, H-10, H-10’); 2.40(m, 2H, H-6, H-6’); 2.53(m, 2H, H-5, H-5’); 2.70(dd, J=6.2, 17.0, 2H, H-7b, H-7b’); 2.80(d, J=17.0, 2H, H-7a, H-7a’); 3.50(t, J=8.0, 2H, H-4b, H-4b’); 4.07(t, J=8.0, 2H, H-4a, H-4a’); 6.43(s, 1H, H-12); 6.77(s, 1H, H-13)ppm; 13C NMR (CDCl3, 300 MHz): 13.4,13.2(C-11), 23.7, 23.5, 23.4, 23.3(C-7), 25.2, 25.0(C-10), 35.7, 35.1(C-5), 44.8(C-6), 74.5, 73.9(C-4), 106.1(C-2), 109.1(C-13), 116.5(C-8), 126.3(C-14), 153.5(C-9, C-9’); EIMS m/z: 330 (M+), 288, 272, 232; HREIMS Found:m/z 330.1824[M] +. C20H26O4. Calculated: M=330.1826.
X-Ray crystallographic data of 8c. crystal system, space group tetragonal, P4(3)2(1)2; Unit cell dimensions a = 11.7950(9) Å, α = 90o; b = 11.7950(9) Å, β = 90o; c = 12.825(2) Å, γ = 90o; volume = 1784.3(3) Å3, Z = 4, Dcalcd = 1.282 Mg/m3, absorption coefficient m = 0.087 mm-1, F(000)=744, crystal size 0.48 × 0.42 × 0.38 mm3; final R indices [I > 2σ(I )], R1 = 0.0446, wR2 = 0.1156.
References
1. Y. C. Lin, X. Y. Wu, S. Feng, G. C. Jiang, J. H. Luo, S. N. Zhou, L. L. P. Vrijmoed, E. B. G. Jones, K. Krohn, K. Steingröver, and F. Zsila, J. Org. Chem., 2001, 66, 6252. CrossRef
2. X. Y. Wu, X. H. Liu, Y. C. Lin, J. H. Luo, Z. G. She, L. Houjin, W. L. Chan, S. Antus, T. Kurtan, B. Elsässer, and K. Krohn, Eur. J. Org. Chem., 2005, 4061. CrossRef
3. K. Krohn and M. Riaz, Tetrahedron Lett., 2004, 45, 293. CrossRef
4. K. Krohn, M. Riaz, and U. Flörke, Eur. J. Org. Chem., 2004, 1261. CrossRef
5. J. D. Pettigrew, J. A. Bexrud, R. P. Freeman, and P. D. Wilson, Heterocycles, 2004, 62, 445; CrossRef J. D. Pettigrew, R. P. Freeman, and P. D. Wilson, Can. J. Chem., 2004, 82, 1640. CrossRef
6. J. D. Pettigrew and P. D. Wilson, Org. Lett., 2006, 8, 1427; CrossRef J. D. Pettigrew and P. D. Wilson, J. Org. Chem., 2006, 71, 1620. CrossRef
7. H. Nambu, G. Anilkumar, M. Matsugi, and Y. Kita, Tetrahedron, 2003, 59, 77. CrossRef
8. H. Yorimitsu, H. Shinokubo, and K. Oshima, Chem. Lett., 2000, 104. CrossRef
9. C. Hackmann and H. J. Schäfer, Tetrahedron, 1993, 49, 4559; CrossRef A. L. J. Beckwith and D. M. Page, Tetrahedron, 1999, 55, 3245; CrossRef S. R. Graham, J. A. Murphy, and A. R. Kennedy, J. Chem. Soc., Perkin Trans. 1, 1999, 3071. CrossRef