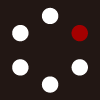
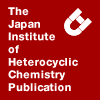
HETEROCYCLES
An International Journal for Reviews and Communications in Heterocyclic ChemistryWeb Edition ISSN: 1881-0942
Published online by The Japan Institute of Heterocyclic Chemistry
e-Journal
Full Text HTML
Received, 9th October, 2008, Accepted, 10th November, 2008, Published online, 11th November, 2008.
DOI: 10.3987/COM-08-S(F)120
■ Flexible Access to Monoterpenoid Indole Alkaloids Using a Cyclopentanoid Chiral Building Block
Masato Hayashi, Keiichi Motosawa, Atsushi Satoh, Masatoshi Shibuya, Kunio Ogasawara, and Yoshiharu Iwabuchi*
Pharmaceutical Institute, Tohoku University, Aobayama, Sendai 980-8578, Japan
Abstract
Expedient, diasterocontrolled transformations of 1 to the key synthetic intermediate of corynanthe, iboga, and aspidosperma-class of monoterpenoid indole alkaloids which led up to a formal synthesis of (+)-20R-dihydrocleavamine, (–)-eburnamonine, and a total synthesis of (+)-aspidospermidine (2) have been demonstrated.Molecules capable of dictating regio- and diastereoselective modifications thereof offer versatile use in organic synthesis. We previously reported an efficient chemoenzymatic preparation of the 2,3,4-trioxycyclopentanol 1 having a dioxabicyclo[3.3.0]octane framework in both enantiomeric forms1 and demonstrated its use in the efficient stereocontrolled synthesis of α-cuparenone,2a (+)-estrone,2b Calabar bean alkaloids,2c and Sceletium alkaloids.2d Highlighting its flexible features, we have now explored its potential as a chiral building block for the representative class of monoterpenoid indole alkaloids derived from secologanin:3 the facts that the overwhelming structural diversity of monoterpenoid indole alkaloids is originated from the common intermediate, secologanin via the adoption of multiple set of biochemical transformations and that secologanin is produced by the oxidative scission of the cyclopentane-ring of loganin (Scheme 1) stimulated our challenge to exploit the further versatility of 1. We report here the diastereocontrolled transformation of 1 to the key intermediates for the synthesis of corynanthe, iboga, and aspidosperma-class of monoterpenoid indole alkaloids which led up to a formal synthesis of (+)-20R-dihydrocleavamine (4),4 (–)-eburnamonine (6),5 and a total synthesis of (+)-aspidospermidine (5).3b,6,7,8 (Scheme 2).
The strategic point of this synthetic venture is to exploit expedient methods addressing issues on how and when to install the requisite side chains selectively at the right positions on the periphery of the cyclopentane ring and to cleave the exact bond by taking a full advantage of functionalities in 1.
Our effort to transform (+)-1 to a key synthetic intermediate of the corynanthe-class of indole alkaloids, featuring the 1,2-vicinal stereogenic centers consisting of secondary alkyl carbons, was commenced with the oxidative activation of the chiral building block (+)-1 to place the base for installation of the two alkyl branches (Scheme 3). Thus, the oxidation of the alcohol (+)-1 was first carried out using IBX9 in DMSO at 60 °C to give the enone 8 in 75% via the ketone 710 with the concomitant β-elimination of a cumyl alcohol. Upon treatment with sodium borohydride in the presence of cerium(III) chloride, the enone 8 afforded the cyclopentenol 9 having the all-cis oxygen stereochemistry as a sole product via the diasterocontrolled delivery of a hydride from the convex-face.11 The installation of the first C2 branch was then conducted by the Eschenmoser reaction of 9 with dimethylacetamide dimethyl acetal in diphenyl ether at 280 °C to give the single cyclopentene 10 having the acetamide moiety cis to the acetonide moiety.1,12,13 Due to the given stereochemical arrangement, the amide 10 allowed consecutive facile deacetonization and lactonization under acidic condition to give the δ-hydroxy-γ-lactone 11, which on treatment with MnO2 gave the enone 12 with a biased platform suitable for the stereocontrolled installation of the second branch intrinsic the corynanthe-family. As expected, reaction of 12 with allyltributylstannane in CH2Cl2 at –78 °C in the presence of TBSOTf furnished the silyl enol ether 13 in 74% yield by concurrent diastereoselective 1,4-addition and O-silylation.14
The task for a regioselective cleavage of the cyclopentane ring of 13 suitable for the corynanthe-class of indole alkaloid could be facilitated with an unexpected side reaction provoked by a protecting group. Thus, on treatment with sodium borohydride in boiling EtOH, 13 gave the diol 14 in 77% yield via the concomitant migration of a TBS group to the possible secondary oxyanion generated right after the partial reduction of the γ-lactone 13. The selective protection of the primary hydroxy group of 14 as the pivalate and PCC oxidation of the remaining secondary hydroxy group and the following Baeyer-Villiger oxidation using mCPBA afforded the lactone 15. On HF-mediated deprotection of TBS group, 15 gave 16, which on sequential sodium borohydride treatment in MeOH, and PPTS-catalyzed lactonization with azeotropic removal of water afforded the δ-lactone 17, [α]D28 +9.23o (c 0.86, CHCl3). In light of the close topological correlations and the functionalities of 16 and 17 with the non-tryptamine moieties of 2 and 3, respectively, 16 should be a potential synthon of (–)-dihydrocorynantheol (2)15 and (–)-antirhine (3).16b,g
The synthetic effort directed toward iboga-type indole alkaloid was put into practice by setting the exact target to (+)-20R-dihydrocleavamine (4), where the construction of the characterictic 1,3-vicinal tertiary alkyl centers was accomplished by applying the Stork radical cyclization protocol17 to the allyl alcohol ent-11 as the key step (Scheme 4). Thus, treament of ent-11, prepared from (–)-1 by employing the same sequence as shown in Scheme 3, with NBS in CH2Cl2 in the presence of ethyl vinyl ether and sodium bicarbonate gave quantitatively the bromoethyl acetal 20, which on treatment with AIBN in boiling toluene in the presence of Bu3SnH furnished the tricyclic lactone 21 in 77% yield.
After acidic hydrolysis of the acetal moiety of 21, the resulting lactol was converted to the dithiane 22 on treatment with ethanedithiol and BF3·OEt2. Upon exposure to Raney-Nickel in EtOH, 22 furnished the hydroxy-lactone 23 with the requisite 20R-ethyl group being arranged in 78% yield. Then, the hydroxy-lactone 23 was exposed to the complex generated from methylamine hydrochloride and trimethylaluminum in THF at reflux to give the dihydroxy-amide 24.18 On sequential periodate cleavage and borohydride reduction in the same flask, 24 afforded the lactone 26, [α]D31 -0.47 (c 0.11, CHCl3) {lit., [α]D26 -0.5 (c 1.0, CHCl3)4b}, via diol 25 through a conccurent cyclization during the acidic work up. Since 26 has been transformed into (+)-20R-dihydrocleavamine (4), this constitutes the development of new formal route to this alkaloid.4b
Exploration of a route to aspidosperma-type indole alkaloid was carried out targeting (+)-aspidospermidine (5) with the intention of adopting the Harley-Mason’s protocol.8
The synthesis was commenced with the formal substitution of the vinylic hydrogen of ent-8 with an ethyl group to prepare for the construction of the quarternary carbon characteristic to aspidosperma-family (Scheme 5). The task was executed via a sequence involving the conjugate addition of a cuprate generated from ethylmagnesium bromide in THF containing HMPA in the presence of copper(I) iodide and triethysilyl chloride at –40 °C, followed by the in situ trapping of the enolate with triethysilyl chloride, and the subsequent oxidation of the resulting silyl enol ether 27 by the palladium-catalyzed protocol developed by Ito and Saegusa19 to furnish the β-ethyl enone 28 in 89%. The reduction of 28 with sodium borohydride in MeOH at –40 °C in the presence of cerium(III) chloride gave the endo-allyl alcohol 29 as the only product by convex-face selective 1,2-reduction. The Eschenmoser rearrangement reaction1,12,13 of 29 was carried in boiling diphenyl ether (~ 280 °C) to give the acetamide 30 having the desired quarternary center in 86% yield as the single product. The acetamide moiety thus arranged was transformed into the hydroxylethyl handle, which is essential for the Harley-Mason reaction, by treating 30 with LiEt3BH20 in THF.
Having served its dual purpose as a stereocontrolling element and a protecting group, the dioxabicyclo[3.3.0]octane moiety of 31 was converted into the two side chains of the secologanin-derived C9 unit suitable for merger with a tryptamine. Thus, posterior to the hydrogenation of 31, the treatment of the resulting product with HIO4 in 1,4-dioxane-H2O afforded the dioxabicyclo[4.3.0]nonane lactol 32, via the sequential hydrolysis of the acetonide moiety, the oxidative cleavage of the resulting 1,2-glycol, and further spontaneous cyclization, which on oxidation with cat. TPAP and NMO21 yielded the dioxabicyclo[4.3.0]nonane lactone 33, as an armed form of the key C9 unit. The projected merger was then conducted by treating 33 with tryptamine in boiling toluene to give the known secondary amide 34, hereby establishing the formal synthesis6a,c of aspidospermidine. As a result of our serious attack to accomplish the synthesis, we found efficient conditions for the promotion of the Pictet-Spengler cyclization of 34. Thus, on warming in phenol22 with a catalytic amount of H2SO4 at 50 °C for 1 h, 34 induced diasteroselective cyclization to give 36 in 70% yield as a 10:1 mixture with C(3) β-epimer as the major product.23
Note that the well-precedent protocol required 48 h refluxing in acetic acid for the Pictet-Spengler reaction to give a 1:1 mixture of the C(3)α, and β-epimers as their acetate in moderate (up to 50%) yield, which required the subsequent alkaline hydrolysis to detach the acetate moiety. The conversion of the alcohol 36 to (+)-aspidospermidine (5) was carried out as described by Harley-Mason and Kaplan: treatment7 in 40% H2SO4 at 100-110 °C for 1.5 h to give 37, and immediate reduction of 37 with LiAlH4 in refluxing THF to give (+)-aspidospermidine (5) in 51% yield. The spectral data (1H and 13C NMR, IR, MS), mp (115-118 °C) and specific rotation of synthetic aspidospermidine [α]D23 +16.4° (c 1.01 EtOH) were consistent with reported values22 (mp 120-121 °C, [α]D23 +17.0 (c 0.60, EtOH)). The present synthesis implies development of a new diastero- and enantiocontrolled synthesis of ebrunamonine, the medicinally important eburnane-class of indole alkaloid, since the starting ketone 1 is available in both enantiomeric form and the transformation of 36 to eburnamonine (6) via 385c,6a has been established.
In conclusion, we have demonstrated the transformation of 1 to the key synthetic intermediates of corynanthe, iboga, and aspidosperma-class of monoterpenoid indole alkaloids which led up to a formal synthesis of (+)-20R-dihydrocleavamine, (–)-eburnamonine, and a total synthesis of (+)-aspidospermidine (2) , thereby extending the utility of 1 as a chiral building block.
This paper is dedicated to Professor Emeritus Keiichiro Fukumoto on the occasion of his 75th birthday.
References
1. H. Nakashima, M. Sato, T. Taniguchi, and K. Ogasawara, Synthesis, 2000, 817. CrossRef
2. (a) H. Nakashima, M. Sato, T. Taniguchi, and K. Ogasawara, Tetrahedron Lett., 2000, 41, 2639; CrossRef (b) K. Tanaka, H. Nakashima, T. Taniguchi, and K. Ogasawara, Org. Lett., 2000, 2, 1915; CrossRef (c) K. Tanaka, T. Taniguchi, and K. Ogasawara, Tetrahedron Lett., 2001, 42, 1049; CrossRef (d) M. Hayashi, T. Unno, M. Takahashi, and K. Ogasawara, Tetrahedron Lett., 2002, 43, 1461. CrossRef
3. (a) J. Stöckigt and M. Ruppert, ‘Comprehensive Natural Products,’ Vol. 4, ed. by D. Barton and K. Nakanishi, Elsevier, New York, 1999, 109; (b) J. E. Saxton, ‘The Alkaloids,’ Vol. 51, ed. by G. A. Cordell, Academic Press, New York, 1998, Chapter 1.
4. Enantiocontrolled synthesis of (+)-20R-15, 20-dihydrocleavamine, see (a) B. Danieli, G. Lesma, D. Passarella and A. Silvani, Tetrahedron Lett., 2000, 41, 3489; CrossRef (b) R. M. Kanada and K. Ogasawara, Tetrahedron Lett. , 2001, 42, 7311; CrossRef (c) M. Amat, C. Escolano, O. Lozano, N. Llor, and J. Bosch, Org. Lett., 2003, 5, 3139. CrossRef
5. For selected total synthesis of (–)-eburnamonine, see (a) S. Takano, M. Yonaga, M. Morimoto, and K. Ogasawara, J. Chem. Soc., Perkin Trans. 1, 1985, 305; CrossRef (b) A. G. Schultz and L. Pettus, J. Org. Chem., 1997, 62, 6855; CrossRef (c) A. G. H. Wee and Q. Yu, Tetrahedron Lett., 2000, 41, 587; CrossRef (d) W. G. H. Andrew and Y. Qing, J. Org. Chem., 2001, 66, 8935; CrossRef (e) Y. Murakami, M. Shindo, and K. Shishido, Synlett, 2005, 664. CrossRef
6. For selected enantiocontrolled synthesis of aspidospermidine, see (a) M. Node, H. Nagasawa, and K. Fuji, J. Org. Chem., 1990, 55, 517; CrossRef (b) D. Desmaële and J. d’Angelo, J. Org. Chem., 1994, 59, 2292; CrossRef (c) A. G. Schultz and L. Pettus, J. Org. Chem., 1997, 62, 6855; CrossRef (d) S. A. Kozmin, T. Iwama, Y. Huang, and V. H. Rawal, J. Am. Chem. Soc., 2002, 124, 4628; CrossRef (e) J. P. Marino, M. B. Rubio, G. Cao, and A. de Bois, J. Am. Chem. Soc., 2002, 124, 13398; CrossRef (f) R. Iyengar, K. Schildknegt, and J. Aubé, Org. Lett., 2000, 2, 1625. CrossRef
7. For recent total synthesis of Aspidosperma alkaloids, see (a) F. He, Y. Bo, J. D. Altom, and E. J. Corey, J. Am. Chem. Soc., 1999, 121, 6771; CrossRef (b) M. A. Toczko and C. H. Heathcock, J. Org. Chem., 2000, 65, 2642; CrossRef (c) Y. Fukuda, M. Shindo, and K. Shishido, Org. Lett., 2003, 5, 749; CrossRef (d) L. A. Sharp and S. Z. Zard, Org. Lett., 2006, 8, 831; CrossRef (e) I. Coldham, A. J. M. Burrell, L. E. White, H. Adams, and N. Oram, Angew. Chem. Int. Ed., 2007, 46, 6159. CrossRef
8. J. Harley-Mason and M. Kaplan, Chem. Commun., 1967, 915. CrossRef
9. (a) M. Frigerio and M. Santagosino, Tetrahedon Lett., 1994, 35, 8019; CrossRef (b) M. Frigerio, M. Santagosino, S. Sputore, and G. Palmisano, J. Org. Chem.,, 1995, 60, 7272. CrossRef
10. Previously, the conversion of 1 to 8 was conducted by a two step sequence consisting of (i) Dess-Martin periodinane-mediated oxidation to give 7, and (ii) warming 7 in acetic acid to promote the β-elimination of a cumyl alcohol; see ref. 1. The facile one-pot conversion of 1 to 8 features the inherent acidic nature of IBX in DMSO. For the discussion on pKa of IBX, see M. J. Gallen, R. Goumont, T. Clark, F. Terrier, and C. M. Williams, Angew. Chem. Int. Ed., 2006, 45, 2929. CrossRef
11. (a) J.-L. Luche, J. Am. Chem. Soc., 1978, 100, 2226; CrossRef (b) A. L. Gemal and J.-L. Luche, J. Am. Chem. Soc., 1981, 103, 5454. CrossRef
12. A. E. Wick, D. Felix, K. Steen, and A. Eschenmoser, Helv. Chim. Acta, 1964, 47, 2425. CrossRef
13. (a) H. Nakashima, M. Sato, T. Taniguchi, and K. Ogasawara, Synlett, 1999, 1754; CrossRef (b) M. Hayashi, T. Unno, M. Takahashi, and K. Ogasawara, Tetrahedron Lett., 2002, 43, 1461. CrossRef
14. S. Kim and J. M. Lee, Synth. Commun., 1991, 21, 25. CrossRef
15. For selected synthesis of (–)-dihydrocorynantheol; (a) T. Suzuki, E. Sato, K. Unno, and T. Kametani, Heterocycles, 1985, 23, 835; CrossRef (b) R. L. Beard and A. I. Meyers, J. Org. Chem., 1991, 56,, 2091; CrossRef (c) M. Ohba, T. Ohashi, and T. Fujii, Heterocycles, 1991, 32, 319; CrossRef (d) T. Itoh, M. Yokota, K. Miyauchi, K. Nagata, and A. Ohsawa, Org. Lett., 2006, 8, 1533; CrossRef (e) A. Deiters, M. Pettersson, and S. F. Martin, J. Org. Chem., 2006, 71, 6547; CrossRef (f) A. Tosaka, S. Itoh, N. Miyaszawa, M. Shibuya, K. Ogasawara, and Y. Iwabuchi, Heterocycles, 2006, 70, 153. CrossRef
16. For selected synthesis of antirhine, (±)-Antirhine: (a) S. Takano, M. Takahashi, and K. Ogasawara, J. Am. Chem. Soc., 1980, 102, 4282; CrossRef (b) S. Takano, N. Tamura, and K. Ogasawara, J. Chem. Soc., Chem. Commun., 1981, 22, 1155; CrossRef (c) T. Suzuki, E. Sato, K. Unno, and T. Kametani, Heterocycles, 1985, 23, 839; CrossRef (d) T. Suzuki, E. Sato, K. Unno, and T. Kametani, Chem. Pharm. Bull., 1986, 34, 3135; (e) A. Pancrazi, J. Kervagoret, and Q. Khuong,-Huu, Tetrahedron Lett., 1991, 32, 4483; CrossRef (f) B. Danieli, G. Lesma, M. Mauro, G. Palmisano, and D. Passarella, Tetrahedron, 1994, 50, 8837; CrossRef (g) M. Kawamura and K. Ogasawara, Tetrahedron Lett., 1995, 36,, 3369. CrossRef
17. (a) G. Stork and N. H. Baine, J. Am. Chem. Soc., 1982, 104, 2321; CrossRef (b) G. Stork and R. Mook, Jr., J. Am. Chem. Soc., 1983, 105, 3720. CrossRef
18. A. Basha, M. Lipton, and S. M. Weinreb, Tetrahedron Lett., 1977, 4171. CrossRef
19. Y. Ito, T. Hirao, and T. Saegusa, J. Org. Chem., 1978, 43, 1011. CrossRef
20. (a) H. C. Brown, S. C. Kim, and S. Krishnamuthy, J. Org. Chem., 1980, 45, 1; CrossRef (b) H. Tanaka and K. Ogasawara, Tetrahedron Lett., 2002, 43, 4417. CrossRef
21. S. V. Ley, J. Norman, W. P. Griffith, and S. P. Marsden, Synthesis, 1994, 639. CrossRef
22. S. Torii, H. TanakThe prolongation of reaction time resulted in the attenuation of the diasteroselectivity in the Pictet-Spengler reaction of 34, suggesting that C(3) β-36 is the kinetically favored producta, M. Taniguchi, and Y. Kameyama, J. Org. Chem., 1991, 56, 3633. CrossRef
23. The prolongation of reaction time resulted in the attenuation of the diasteroselectivity in the Pictet-Spengler reaction of 34, suggesting that C(3) β-36 is the kinetically favored product.
24. G. F. Smith and M. A. Wahid, J. Chem. Soc., 1963, 4002. CrossRef